Syllabus (Fourth Edition, 2023)
Topics
i. Describe the normal nutritional requirements, including vitamins and trace elements.
ii. Describe the physiology of fat, carbohydrate, and protein metabolism.
iii. Define basal metabolic rate and outline the factors that influence it.
iv. Describe the measurement of metabolic rate.
v. Describe anaerobic metabolism and ketone production.
vi. Describe the pharmacology of enteral and parenteral nutrition.
Topics not covered in previous SAQs
.
Learning Objectives for the First Part Examination in Intensive Care Medicine
- This will ensure that trainees, tutors, and examiners can work from a common base.
- All examination questions are based around this Syllabus.
- These learning objectives are designed to outline the minimum level of understanding required for each topic.
- The accompanying texts are recommended on the basis that the material contained within them provides sufficient information for trainees to meet the learning objectives.
- Trainees are strongly encouraged to explore the existing and evolving body of knowledge of the Basic Sciences as they apply to Intensive Care Medicine by reading widely.
- For all sections of the syllabus an understanding of normal physiology and physiology at extremes of age, obesity, pregnancy (including foetal) and disease (particularly critical illness) is expected.
- Similarly, for pharmacology, trainees are expected to understand a drug’s pharmacology in these contexts.
- An understanding of potential toxicity and relevant antidotes is also expected.
Definitions
Throughout the document specific wording has been used under the required abilities to indicate the level of knowledge and understanding expected and a glossary of these terms is provided.
Definitions
Calculate | Work out or estimate using mathematical principles. |
Classify | Divide into categories; organise, arrange. |
Compare and contrast | Examine similarities and differences. |
Define | Give the precise meaning. |
Describe | Give a detailed account of. |
Explain | Make plain. |
Interpret | Explain the meaning or significance. |
Outline | Provide a summary of the important points. |
Relate | Show a connection between. |
Understand | Appreciate the details of; comprehend. |
SAQs
i. Describe the normal nutritional requirements, including vitamins and trace elements.
2020B 09 – 2017B 02
Outline the daily nutritional requirements, including electrolytes, for a normal 70 kg adult
CICMWrecks Answer
MACRONUTRIENTS
CHNOPS (carbon, hydrogen, nitrogen, oxygen, phosphorus, and sulphur)
Provide bulk energy
- Carbohydrates: compounds made up of types of sugar.
- monosaccharides (such as glucose and fructose)
- disaccharides (such as sucrose and lactose)
- oligosaccharides
- polysaccharides (such as starch, glycogen, and cellulose).
- Proteins: are organic compounds that consist of amino acids joined by peptide bonds.
- Essential (cannot be manufactured in the body: phenylalanine, valine, threonine, tryptophan, methionine, leucine, isoleucine, lysine, and histidine) and non-essential
- Fats: glycerin molecule with three fatty acids attached.
- Essential (alpha-linolenic acid (ω-3) and linoleic acid (ω -6)) and non-essential
- Saturated and Unsaturated
Biomolecule | Kilocalories per 1 gram |
---|---|
Protein | 4 |
Carbohydrate | 4 |
Fat | 9 |
MICRONUTRIENTS
Support metabolism
- Dietary minerals: exogenous chemical elements indispensable for life
- Vitamins: organic molecules essential for an organism that are not classified as amino acids or fatty acids. They commonly function as enzymatic cofactors, metabolic regulators, or antioxidants.
Suggested daily nutritional requirements in humans
Requirement | 70 kg Adult | |
---|---|---|
ENERGY REQUIREMENTS | ||
Calories | 25kcal/kg | 1750kcal |
Energy | 100kJ/kg | 7000kJ |
MACRONUTRIENTS | ||
Carbohydrate | 4g/kg | 280g |
Protein | 1.5g/kg | 105g |
Fat | 1g/kg | 70g |
WATER AND ELECTROLYTES | ||
H2O | 30 mL/kg | 2100ml |
Na+ | 2mmol/kg | 140mmol |
K+ | 1mmol/kg | 70mmol |
Ca2+ | 0.1mmol/kg | 7mmol |
Mg2+ | 0.1mmol/kg | 7mmol |
PO4 | 0.1mmol/kg | 7mmol |
VITAMINS | |
Water sol B complex folate vitamin C vitamin B12 | |
Fat soluble ADEK | |
TRACE ELEMENTS | |
Fe | 10mg |
Zn | 15mg |
Cu | 3mg |
iodine | 150μg |
manganese | 5mg |
chromium | 200μg |
selenium | 200μg |
Please include common sources of specific nutrients – This is best done based on individual candidate’s method of presentation
JC 2020
Examiner Comments
2020B 09: 40% of candidates passed this question.
This topic is well covered in the recommended physiology textbooks. Many answers unfortunately simply listed the various components without providing sufficient detail; outline questions require some context around the key points as opposed to just a list. Most candidates had a good estimate for the basal energy requirements of a resting adult. Good candidates were able to outline the g/kg daily protein requirements and the distribution of remaining energy intake between carbohydrates and lipids and included how this may change during periods of stress. They also stated the energy derived per gram of each of those food groups. Few candidates mentioned the need to include essential amino acids. Similarly, with fat intake, few candidates mentioned the need for essential fatty acids. A definition of “vitamin” would have received credit. Most candidates were able to classify vitamins as water soluble or fat soluble. Most candidates mentioned trace elements (with an abbreviated list) and mentioned bone minerals. A daily intake requirement for Na and K was expected, though not for bone minerals or trace elements.
2017B 02: 21% of candidates passed this question.
The provision of nutrition is a core skill in ICU. An understanding of its key elements enables prescription and modification. However, most answers lacked detailed information which is available in the standard texts. Better responses outlined the caloric requirements including each major element (water, carbohydrate, fat and protein) along with the caloric values and potential sources. Essential amino acids, fatty acids, fat and water-soluble vitamins were expected. A list of the requirements for major electrolytes and some of the trace elements were expected. Some candidates seemed to confuse calories, kilocalories and kilojoules. Some answers did not provide the nutritional requirements, as asked, but instead discussed the fate of the nutrients; hence did not score marks. Candidates are reminded to read the question carefully.
ii. Describe the physiology of fat, carbohydrate, and protein metabolism.
2013A 05
Describe the hormonal response to a meal.
CICMWrecks Answer
GIT
- Complex interplay between neuronal (ANS and ENS) and hormonal functions
Cephalic phase
- Initiation of GI secretions in preparation of a meal
- Vagal stimulation via acetylcholine
- Enterochromaffin cells cause release of histamine
- Acts on histamine receptors on gastric parietal cells
- Increase gastric HCl secretion
- G-cells → secreation of Gastrin
- Enterochromaffin cells cause release of histamine
Gastric phase
- Gastric wall stretch and peptide content in gastric lumen → stimulates G-cells in antrum → increases secretion of gastrin
- Peptide hormone
- Acts on CCK B receptors on enterochromaffin cells
- Increased histamine release → HCl secretion
- Stimulates secretion of pepsinogen by chief cells
- Increases antral pump function
Intestinal phase
- As gastric contents emptied into duodenum, low pH stimulates secretion of secretin by duodenal S cells
- Peptide hormone
- Inhibits gastric emptying
- Increases pancreatic secretion of a bicarbonate rich fluid
- Protein and FFA content in the duodenum stimulates secretion of cholecystokinin by enteroendocrine cells of the duodenum
- Peptide hormone
- Inhibits gastric emptying
- Increases pancreatic secretion of enzymes for digestion of fats and proteins
- Causes gall bladder contraction and release of bile for absorption of fats
As nutrients is absorbed from intestine
- Blood [glucose] increases → insulin secretion by pancreatic β cells
- Increases hepatic glucose uptake and glycogenesis, protein and lipid synthesis
- Increases skeletal muscle glucose uptake and glycogenesis, protein synthesis
- Increases adipocyte lipid storage
- Adipocytes secrete leptin in response to increased fat stores → suppress appetite
Long after meal
- Stomach Ghrelin cells secrete ghrelin in response to decreased gastric stretch
- Increased sensation of hunger
Sakurai 2016
Examiner Comments
2013A 05:
For a good answer candidates were expected to have an integrated knowledge of gastrointestinal physiology. Gut function is regulated by the enteric nervous system and by paracrine and endocrine hormones released by hormone secreting cells in the mucosa of the gut (enteroendocrine cells). These cells secrete hormones in response to neural innervation or in response to triggers associated with ingested food. Gut functions influenced include secretion, digestion, absorption and motility. The endocrine system also has an important role in the handling of nutrients following absorption and some mention of insulin was required.
2012A 22
Outline the importance of the citric acid cycle in metabolism
CICMWrecks Answer
The Citric Acid Cycle
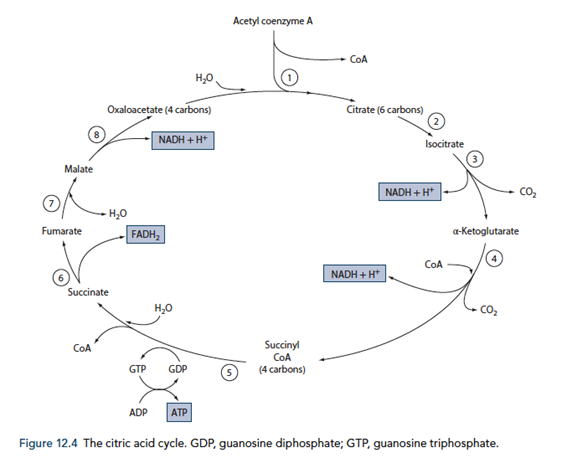
Energy production
- Required for the total metabolism of glucose, for the production of approx. 38 ATP (Compared to 8 ATP production from metabolism of glucose to pyruvate)
- Also used in the metabolism of fats, ketone bodies and amino acids via Acetyl CoA
- 12 ATP generated for each Acetyl CoA molecule
- Occurs within the mitochondrial matrix
- Pyruvate generated in glycolysis is converted to Acetyl CoA by pyruvate dehydrogenase releasing 1 NADH per pyruvate molecule (leading to the generation of 6 ATP equvalents per glucose molecule)
- Acetyl CoA condensed with oxaloacetate to for citric acid and in a number of subsequent steps forms 1GTP, 3NADH and 1FADH2 (Total of 24 ATP equivalents per glucose molecule)
- The NADH and FADH2 generated in the citric acid cycle is used to generate ATP with O2 as the terminal electron carrier to form water.
- Therefore O2 is required for the citric acid cycle to continue
- The electron transport chain
- Inner mitochondrial membrane
- Series of 4 cytochrome enzymes that pump H+ across into intermembrane space as electrons are transferred from NADH and FADH2
- NAD and FAD regenerated in the process replenishing substrates for the citric acid cycle
- Proton gradient used to generate ATP from ADP and Pi
- CO2 in generated in the citric acid cycle
Note: Erythrocytes lack mitochondria and therefore are unable to utilize the citric acid cycle for energy production (Therefore can only utilize glucose)
Biosynthesis
- Components of the citric acid cycle are used for biosynthesis of fuels
- Malate and oxaloacetate – used in gluconeogenesis
- Citrate – used in fatty acid and cholesterol generation
- Oxaloacetate and αketoglutarate – used in amino acid synthesis
- Succinyl CoA – used in porphyrin synthesis
- Oxaloacetate – Porphyrins
Sakurai 2016
Examiner Comments
2012A 22: 2 (20%) of candidates passed.
Only an outline of the importance of the citric acid cycle (CAC) was expected, and not a detailed understanding. Candidates struggled to appreciate its importance. For a good answer candidates were expected to mention that the CAC has two main functions: energy production and biosynthesis. Some understanding of the overall reaction ( AcCoA + 3NAD+ + FAD + GDP + Pi + 2H2O 2CO2 + 3NADH + FADH2 + GTP +CoA ), the link between oxidation of metabolic fuel (carbohydrates, lipids and protein) and aerobic energy production (oxidative phosphorylation), that Acetyl CoA is final common product of these oxidations (Glucose via glycolysis (cytoplasm), Free fatty acids via β oxidation (mitochondrial matrix) and Amino acids – glucogenic and ketogenic reactions), that CAC is first stage of aerobic respiration, requires oxygen to proceed, that the CAC
together with oxidative phosphorylation accounts for 2/3 to 3/4 of ATP generated from fuel oxidation and anabolic involvement, whereby several biosynthetic pathways utilize CAC intermediates, e.g. Glucose biosynthesis (gluconeogenesis) via malate then oxaloacetate, fatty acid and cholesterol biosynthesis via citrate + CoA AcCoA, Amino acid biosynthesis via reductive amination and transamination reactions, Porphyrin (organic component of haeme) via succinyl CoA and Purine and pyrimidine biosynthesis – precursors of nucleotide bases for DNA and RNA.
2020B 04 – 2015A 18
Outline the role of the liver in the metabolism of fat (1/3 of marks),
carbohydrate (1/3 of marks) and proteins (1/3 of marks).
CICMWrecks Answer
Fat
Anabolic Role
Synthesis or lipoproteins for transport of lipids from dietary FFA’s
- Chylomicrons
- LDL/HDLs
Lipogenesis via citrate in the TCA:
- Citrate leaves mitochondria and is converted back to Acetyl-CoA
- Acetyl-CoA goes to Malonyl-CoA (conversion ↑d by insulin)
- Fatty acids: via fatty acyl-CoA
- Malonyl-CoA → Fatty-Acyl CoA in the reverse of B-oxidation and creates Triglycerides
- Cholesterol: via HMG-CoA
- blocked by statins
Catabolic Role
Β-oxidation:
- FACoA synthase in cytosol makes FACoA
- FACoA → Acyl carnitine by CPT1 and transported into cell
- Acyl carnitine → FACoA by CPT2
- Undergoes B oxidation
- 1 Acetyl CoA (by cleaving the 2 carbon-CoA from the whole molecule) → Used in TCA cycle
- 1 FADH2 + 1 NADH to be used in the Electron Transport Chain.
- → up to 17 molecules of ATP
Carbohydrate
Anabolic Role
Glucostat Function
- Gluconeogenesis from pyruvate derived from
- Complex polysaccharides (fructose and galactose)
- Glycogen
- Gluconeogenesis
- lactate, pyruvate, glycerol (from TAGs), glucogenic a.a’s (esp Ala and Glu)
- Pentose phosphate shunt
- Glycerol (but not FFAs)
- Glycogen synthesis
Catabolic Role
Glycolysis:
- Primary function
- For generation of pyruvate + 2 ATP
- Pyruvate then utilised to form
- fat, AA’s and ketones
- Lactate
Glycogenolysis:
- From G6P.
Protein
Anabolic Role
Generation of functional proteins in serum:
- Albumin
- Fibrinogen
- Coagulation proteins
- Regulatory proteins
- Coagulation factors
- Complement proteins
- Globulins
- α1 (α1 anti-trypsin, α1-fetoprotein)
- α2 (haptoglobulin),
- β (transferrin)
Amino acids and nucleosides from α-ketogluterate in the TCA
Haeme from Succinyl-CoA in the TCA
Catabolic Role
- Breakdown of proteins to form
- ketones as energy source (liver, heart and brain)
- free amino acids for addition to the AA pool.
- Amino acids utilisation for energy or protein synthesis
- Ammonia metabolism and recycling
- Deamination of fatty acids
- Urea formation for ammonia removal Part of the urea cycle takes place in liver
Gladwin 2016
Examiner Comments
2020B 04: 54% of candidates passed this question.
This question relates to basic hepatic physiology and is well described in the recommended texts.
The mark allocation and division of time was indicated in the question. Better answers used the categorisation in the question as an answer structure. Many candidates gave a good description of beta oxidation, the formation of Acetyl Co A and ketone synthesis. A description of the synthesis of cholesterol, phospholipids, lipoproteins and fatty acid synthesis from proteins and carbohydrates mainly using glycogen, glucose and lactate also received marks. Candidates seem to have a better understanding of fat and glucose metabolism than protein metabolism. Higher scoring candidates appreciated the anabolic and catabolic processes of each component.
2015A 18: 50 % of candidates passed this question.
Most candidates seemed not to have thought about this before and so collated information from answers about insulin and glucagon, and starvation. Many added information about absorption and digestion which was not required.
Metabolic functions of the liver form part of the “standard list” of functions of the liver yet few details could be provided beyond that. It was expected answers would detail the central role of the liver as a “glucostat” and its role in glucose utilization. It has two main roles in lipid metabolism, the synthesis of fatty acids and the partial oxidation of fatty acids to ketone bodies. The liver also plays a central role in protein catabolism and anabolism. It plays a major role in the breakdown of amino acids gluconeogenesis and protein synthesis. The liver also releases amino acids into the blood for utilization by peripheral tissues.
2017A 02
Outline the components of dietary fat (20% of marks).
Describe their possible metabolic fates (80% of marks)
CICMWrecks Answer
- Fat
- triglycerides -> hydrolysed to FFAs & glycerol
- transported to tissues
- glycerol -> glycerol-3-phosphate & enters glycolytic pathway
- FFA’s transported to mitochondria by carnitine carrier -> degraded to ACoA (beta oxidation) -> enters the citric acid cycle.
- essential fatty acids = linolenic, linoleic & arachidonic acid
- used in the production of leukotrienes, prostaglandins, prostacyclin
- Main Components of Dietary Fat
- Fatty acids: Acylglycerides (esters of glycerol+FA), Phospholipids (2FA+glycerol+Phosphate group)
- Non Glyceride Components: Vitamin E, Carotenoids, Vitamins A & D
- Other components: Sterols, Methylsterols and trierpene alcohols, Squalene, Oryzanols
Lipid Transport
- Digestion: splitting TGs into monoglycerides + FFAs
- Absorption: inside intestinal epithelial cells; resynthesis into TGs + packaged as chylomicrons → circulate in venous system for transport to liver + adipose tissue
- Storage: lipoprotein lipase hydrolyses TGs + phospholipids in chylomicrons → FFAs + glycerol → diffuse into fat (adipose cells) + liver (hepatocytes) → resynthesises as TGs inside cells for storage
- Transport: via synthesised lipoproteins which contain TGs, cholesterol, phospholipids, and protein; formed in liver; transport + deposit lipid components in blood + peripheral tissues. E.g. of lipoproteins – chylomicron, VLDL, LDL, HDL
- Synthesis: liver can synthesise TGs from carbohydrates; cholesterol + phospholipids from FFAs
- Mobilisation: stored fat is hydrolysed by hormone sensitive lipase → released + transported as glycerol + FFAs → ionised and carried on albumin. Activation by starvation
Lipid Metabolism
- Glycerol can be converted to Glucose by the liver
- Free Fatty Acids:
- transported from ECF to ICF by fatty acid transporter
- FACoA synthase in cytosol makes FACoA
- Converted to Acyl carnitine by CPT1 and transported into mitochondrion
- Converted back to FACoA by CPT2
- β-oxidation of FFA (Phase 1 reaction)
- FFA derived from diet and lipolysis of fat stores (via lipoprotein transport) is partially oxidised in mitochondrial matrix by removal of 2-C moieties (as acetyl CoA) at a time
- Lipolysis of fat stores is ↑ by GH, GC and Adr (stimulates TAG lipase)
- TCA cycle (Phase 2 reaction)
- occurs in mitochondrial matrix under aerobic conditions only
- → consumes breakdown products of glucose (as acetyl CoA), FFA (as acetyl CoA) and a.a (as TCA intermediates – α-ketoglutarate, oxaloacetate, fumarate, succinyl CoA) to produce
- → (i) 2x CO2, (ii) 1x ATP, (iii) 3x NADH + H+ / 1x FADH2 – per acetyl-CoA metabolised
- TCA cycle stimulated by ↓ NADH/NAD+ ratio (as NADH inhibits dehydrogenase enzymes of the cycle)
- Electron Transport Chain (Phase 3 reaction)
- Electrons donated to ETC by NADH/FADH2 → passed along series of cytochromes (along inside surface of inner mitochondrial membrane) down its energy gradient until they are accepted by O2 at the end (via cytochrome a)
- transported from ECF to ICF by fatty acid transporter
- Transported as chylomicrons to Adipocytes
- o Degraded by Lipoprotein lipase to FFAs and Glycerol (Heparin is a cofactor)
- o FFAs and Glycerol transported into Adipocyte
- o Converted back to Triglycerides for storage.
- Ketone body metabolism:
- Ketone bodies (acetoacetate, β-OH-butyrate) are formed in liver only when there is extra acetyl CoA formed by β-oxidation of excess FFA (2° to ↑ lipolysis by GH, GC, Adr)
- Ketone bodies are released from liver → utilised peripherally by skeletal muscle, heart, kidney (and brain/nervous tissue during starvation)
Bianca / Kerr 2016
Examiner Comments
2017A 02: 21% of candidates passed this question.
Almost all candidates interpreted “metabolic fate” to mean absorption, digestion and transport of fat. Hence a lot of time was spent on this and little on the fate of fat once it enters the blood stream. The processes of neither beta oxidation, nor lipogenesis were not well understood.
Ketone body production was better understood.
2014B 09
Outline the fate of the triglyceride component of orally ingested fat.
CICMWrecks Answer
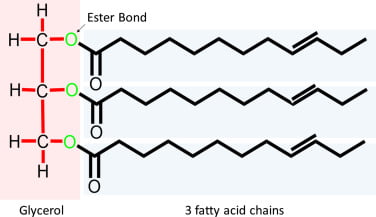
Triglyceride
- 3x long fatty acid molecules joined by glycerol
- eg. stearic/oleic/palmitic acid
Minimal Breakdown in the Upper GIT.
After exiting the stomach:
- Pancreatic lipase
- Triglycerides to FFAs and 2-monoglycerides
- Pancreatic esterase
- Cholesterol esters to cholesterol
- Actions accelerated by emulsification from bile salts
- Facilitate intestinal digestion of lipids
- aids pancreatic lipase activity
- Enhances intestinal absorption of lipids by
- emulsifying lipids
- forming “micelles” (as it is amphipathic)
- Induces intestinal motility
- Choleretic action
- bile salts → ↑ hepatocyte bile production
- Facilitate intestinal digestion of lipids
Transfer into Enterocytes by Passive diffusion as FFAs
In Enterocytes:
- Short/Medium Chain FFAs
- Diffusion into portal circulation
- Bound to albumin and trasported to liver
- Triglyceride formation
- Cholesterol
- Esterified in enterocytes
- Long Chain FFAs
- Encapsulated in phospholipids
- Cholesterol and LC FFAs combine to form TGs
- Packaged in Chylomicrons
- Exocytosed to lymphatics for transport to liver
- Cholesterol
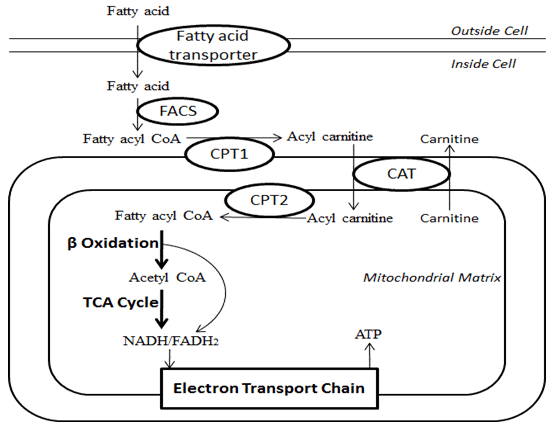
Transport to Liver
- TAGs absorbed as glycerol, FAs, MAG, DAG
- Transported to the liver:
- Short-chain FAs (< 12 C)
- transferred directly tvia portal vein without re-esterification
- Long-chain FAs
- re-esterified to form TAGs
- packaged with cholesterol esters within Chylomicrons
- transported via lymphatics to adipocytes
- Lipids deposited in liver
- Chylomicron remnants with remaining lipids are then taken up by liver
- Short-chain FAs (< 12 C)
Fate
- Glycerol can be converted to Glucose by the liver
- Fatty acids transported from ECF to ICF by fatty acid transporter
- FACoA synthase in cytosol makes FACoA
- Converteted to Acyl carnitine by CPT1 and transported into cell
- Converted back to FACoA by CPT2
- Undergo β-oxidation
- Each cycle generates:
- 1 molecule of Acetyl CoA (by cleaving the 2 carbon-CoA from the whole molecule) which is used in TCA cycle
- 1 molecule of both FADH2 and NADH to be used in the Electron Transport Chain.
- These together produce up to 17 molecules of ATP
- Each cycle generates:
- Transported as chylomicrons to Adipocytes
- Degraded by Lipoprotein lipase to FFAs and Glycerol
- FFAs and Glycerol transported into Adipocyte
- Converted back to Triglycerides for storage.
- In Starvation:
- β-oxidation → ↑ acetyl-CoA → converted to HMG-CoA in liver → ketone bodies (AcAc, β-OH-butyric acid, acetone)
- used as alternate fuel source in liver, heart and brain
Gladwin 2016
Examiner Comments
2014B 09: 8% of candidates passed this question.
Triglycerides (TGs) consist of a 3 carbon glycerol backbone with 3 fatty acids attached. It was expected candidates would detail the fate from digestion and absorption, through distribution to storage and metabolism. Most candidates knew the absorptive processes very well but knowledge of TGs fate once it was packaged into a chylomicron was lacking. Some detail was expected on the passage to the liver via the portal circulation, packing and unpacking and distribution to the body. Very few candidates mentioned appropriate hormones and enzymes, target cells or ketogenesis. Some discussion was expected on the possible fate of acetyl Co A. Comment on the synthesis of lipids and the existence of essential fatty acids gained additional marks.
2011A 05
Outline the process of digestion and absorption of dietary carbohydrate.
CICMWrecks Answer
Introduction
Carbohydrate
- Equal proportions of carbon and H20
- C:H:O = 1:2:1
Starch
- Polysaccharide which functions as a carbohydrate store in green plants.
- Range in structure from linear to highly branched.
Dietary Forms
- Polysaccharides
- Plant sources: Amylopectin, amylose
- Animal souces: Glycogen
- Disaccharides
- Lactose and Sucrose
- Monosacchaerides
- Glucose and Fructose
Absorption and digestion: Takes place throughout GIT
- Conversion of Starches and Glycogen to Di and monosaccharides
- sucrose: Glucose-Fructose (α-glycosidic)
- maltose: Glucose-Glucose (α-glycosidic)
- lactose: Glucose-Galactose (β-glycosidic)
- Initial breakdown in Upper GIT
- Salivary alpha-amylase in mouth
- Action terminated by acidic pH of stomach.
- Salivary alpha-amylase in mouth
- On exiting the stomach
- Pancreatic alpha-amylase on brush border
- Maltose by Maltase
- Maltotriose
- Glucose Polymers
- alpha-limit dextrans
- Lactase
- Sucrase
- Pancreatic alpha-amylase on brush border
- Absorption in intestine (maximal rate = 120g/hr)
- Galactose (C4)
- Pentoses (C5)
- Rapidly absorbed
- Hexoses (C6)
- Rapidly absorbed
- Glucose:
- Secondary active transport
- Basal Na/K atpase
- 20% Cotransport of Glucose/Na from lumen via SGLUT (shares with Galactose)
- 80% Passive diffusion down concentration gradient.
- Apically Via Glut2 to portal circulation
- Fructose (C6)
- Carrier mediated facilitated diffusion via GLUT5
Transported to the liver via the portal circulation
- 10% stored as glycogen
- 40% converted to TAGs as fat stores
- 50% used in glycolysis (forms ATP for other liver functions)
Gladwin 2016
Examiner Comments
2011A 05: 2 (17%) of candidates passed this question.
A number of candidates had absolutely no understanding of this subject. Some candidates had a basic understanding of either digestion or absorption but few demonstrated knowledge of both processes. Inaccuracies were common with many discussing the role of the gastric acid & enzymes in carbohydrate digestion. Most forgot to mention simple dietary carbohydrates. For a good answer candidates were expected to outline the forms of dietary carbohydrates, gastro-intestinal enzyme action and mechanism of absorption
Syllabus: Q1, 2c
Recommended sources: Review of Medical Physiology, Ganong, Chp 27
2011B 11
Outline protein metabolism, including the effects of starvation
CICMWrecks Answer
Protein Metabolism
Amino acids
- Proteins are polypeptides created from amino acids linked together by peptide linkages
- 20 amino acids present in the body in significant quantities
- Of the 20, 10 are essenstial amino acids, which cannot be synthesized within the body in sufficient quantities and must be obtained, pre-formed, from food.
- Amino acids absorbed from diet
- Absorbed by cells, especially hepatocytes
- Amino acids re-absorbed from filtrate in PCT
Protein anabolism
- Peptide linkages → covalent bonding of amino acid amine group and carboxyl group by condensation
- Proteins created in ribosomes in rough endoplasmic reticulum, according to translation of mRNA, which is itself transcribed from cell DNA
- Further post-translational processing of proteins occur in the smooth endoplasmic reticulum (e.g. glycosylation)
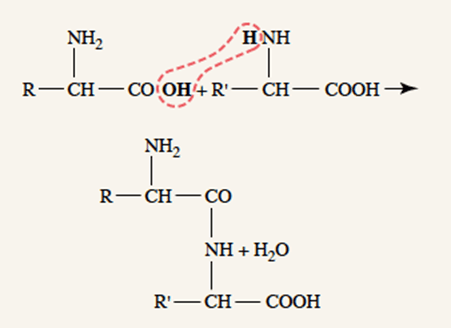
Protein storage
- After entering a cell, amino acids almost immediately converted into proteins, therefore intracellular amino acid concentration remains low
- Amino acids “stored” as proteins
- The liver stores large amounts of exchangeable proteins as rapidly available amino acid source
Protein Catabolism
- Proteins destined for degradation are “tagged” by another regulatory protein, ubiquitin, and targeted for degradation into it amino acid components by the proteosome in the cytosol
- Amino acids are recycled for formation of other proteins
- Use of proteins for energy
Deamination → Release of NH3 + NADH + H+- Ammonia released from deamination is removed from the blood by conversion to urea in the liver
- 2 x NH3 + CO2 → Urea + H2O
- Deaminated amino acids can be used in the citric acid cycle
- via Acetyl-CoA, ketogenesis or conversion to fatty acids
- Deaminated amino acids can be used for gluconeogenesis
- Ammonia released from deamination is removed from the blood by conversion to urea in the liver
Regulation of Protein Metabolism
- Insulin and growth hormone promote storage of amino acids as proteins
- Glucocorticoids promote release of amino acids from protein depots
- Testosterone promotes storage of proteins in cells (esp. muscle)
- Thyroxine → increases metabolism
- In presence of excess fat and carbohydrate → protein synthesis and storage
- In fat and carbohydrate defecit → increased protein break-down
Starvation
- Daily obligatory protein loss
- ~30g
- Except for obligatory protein loss, body utilizes carbohydrates and fats almost exclusively
- Once body carbohydrate and fat stores are depleted, amino acids are oxidized and used for energy
- ~125g protein / day
Sakurai 2016
Examiner Comments
2011B 11: 1 (4%) of candidates passed this question.
For a good answer candidates were expected to mention that there were “essential” and nonessential proteins”, that protein synthesis draws on amino acids sourced from the common amino acid pool (because there is no cellular storage of amino acids) which is the combination of amino acids derived from gut absorption of digested dietary protein and also the continuous turnover of endogenous protein, amino acids filtered via the glomerulus are reabsorbed within the renal tubule and that in health losses are minor, but increases with illness. Protein synthesis is a semicontinuous activity, the rate of which varies considerably between cells under the influence of factors neuro- endocrine factors, substrate availability and energy availability. Proteolysis occurs within nuclear and cytoplasmic proteasomes, via peptide bond disruption catalysed by proteases, which are then processed further into amino acids being then available for release into the amino acid pool or utilised immediately for protein synthesis.
In relation to starvation candidates were expected to mention that it results from a severe ongoing restriction of protein, energy and nutrient intake. That initially, glycogen stores from the liver and skeletal muscle are utilised after conversion to glucose and provide energy, thus sparing of fat and protein stores from use as an energy source for a period of less than a day, following which fats are increasingly utilised and finally protein catabolism accelerates, especially from the liver and skeletal muscle, with relative sparing of the heart and brain.
Syllabus: K2c,f
Recommended sources: Ganong Review of Medical Physiology Chp 2
2010A 20
Explain the role of urea in the body.
CICMWrecks Answer
Urea
- Amino acids -> ammonia -> urea
- 30g produced per 100g of dietary protein per day
- Normal serum concentration: 3-6mmol/L
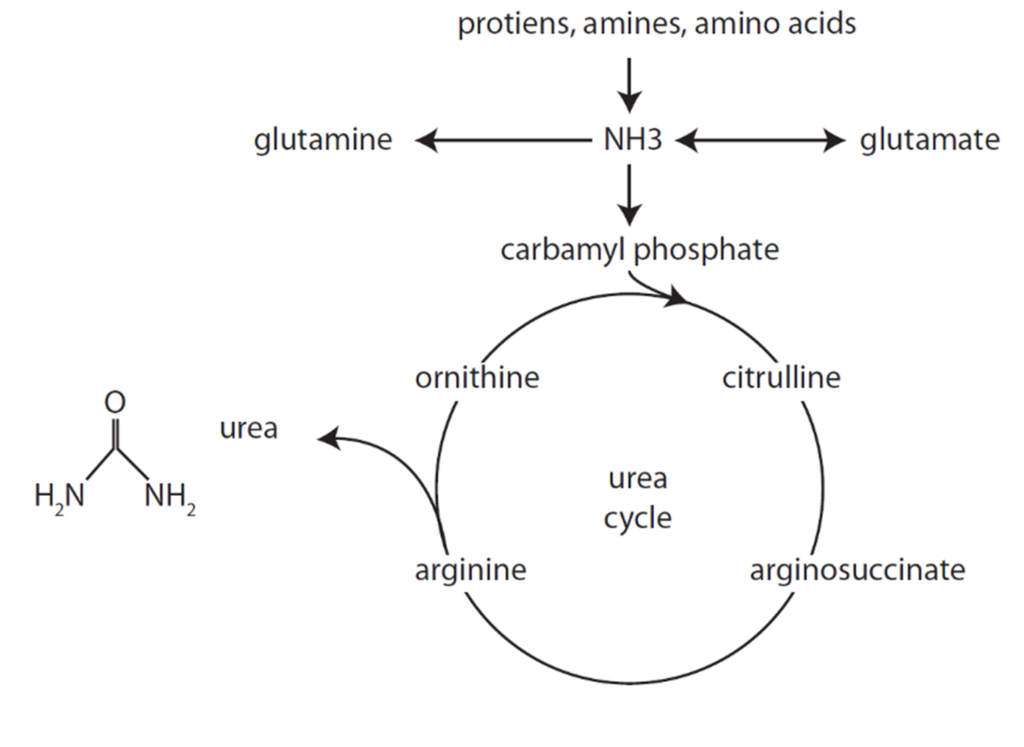
- 60% of renally filtered urea is eliminated
Important in maintaining the counter-current multiplier in the renal loop of Henle and collecting duct
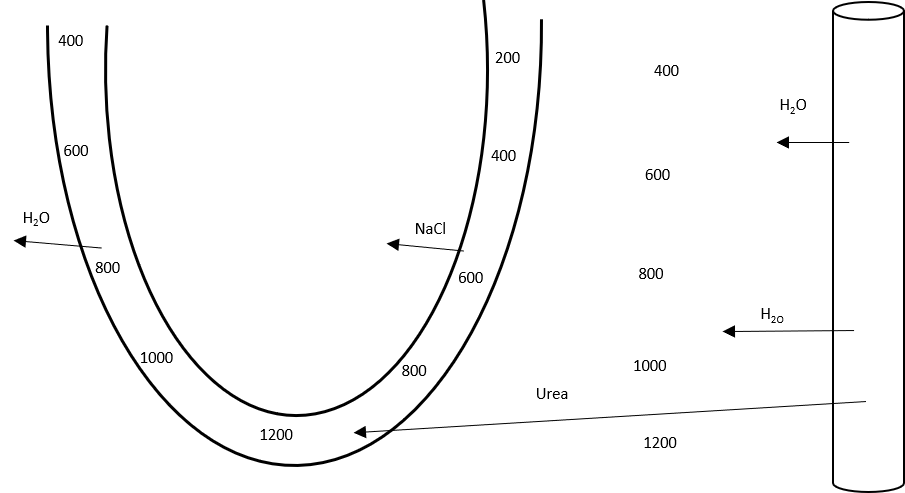
- Ascending limb – active transport of sodium and chloride out of lumen
- Increases osmolality of interstitial fluid
- Descending limb – permeable to water
- Filtrate constantly refreshed
- Leads to concentration gradient, with osmolality increasing toward the medullary interstitial fluid and tubular lumen
- In absence of vasopressin, dilute urine is excreted
- In presence of vasopressin
- Aquaporin 2 channels open in the collecting duct, allowing movement of water out of the lumen and into the interstitial fluid, particularly in medulla where osmolality is high
- Collecting duct becomes permeable to urea
- Urea increases osmolality of interstitum
- Allowing resorption of Na+ and Cl–, while maintaining a high interstitial osmolality
- Urea increases osmolality of interstitum
Mooney 2016
Examiner Comments
2010A: 1 (10%) of candidates passed this question
This question invited candidates to describe the Urea Cycle in the liver. Urea is a waste product derived from deamination of amino acids and the detoxification of NH3. Many candidates did not outline the major steps in the biochemical process.
The kidneys excrete up to 60% of the filtered urea load. The counter-current exchange mechanism in the renal medulla traps urea in the interstitium and generates a concentration gradient, essential for reabsorption of water. The handling of urea by the Loop of Henle and collecting duct, as well as the effect of ADH, should have been discussed.
Many candidates did not include sufficient detail in their answers.
Syllabus: D1 2c,g. I2a.
References: Textbook of Medical Physiology, Guyton p 795, 319.
2015B 16
Describe ammonia metabolism and excretion (70% of marks).
Outline the pharmacology of lactulose (30% of marks).
CICMWrecks Answer
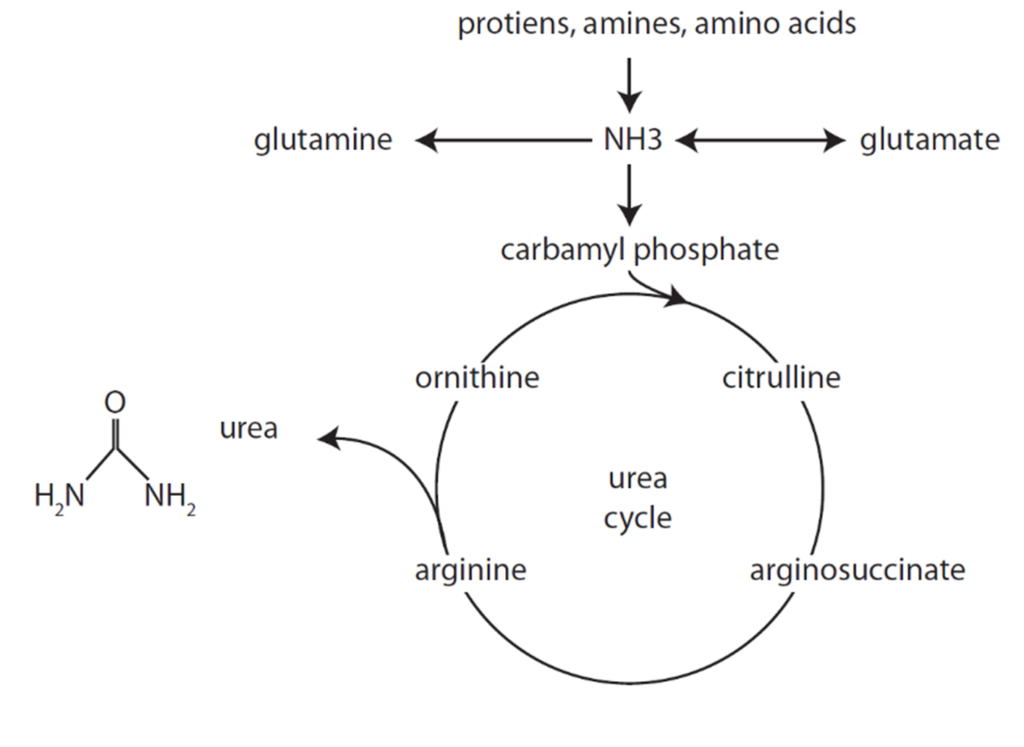
Formation of Urea from Ammonia
- Liberation of ammonia from AAs
- Formation of carbamoyl phosphate from NH3
- Reactions of the urea cycle
Urea Cycle
- Ammonia carried in blood from tissues to liver mitochondria by glutamate as amino groups.
- Hepatocyte Mitochondria
- 2ATP+CO2+NH3 + Carbamoyl Phosphate → citrulline using enzyme Carbamoyl phosphate synthetase 1
- Hepatocyte Cytosol
- Citrulline + ATP + H2O → ornathine + urea
- Urea Fate:
- Enterohepatic circulation (25%)
- Excreted by kidneys (75%).
- Ornathine reenters the mitochondria and is combined with new carbamoyl phosphate to form citrulline
Excretion of ammonia by Kidney
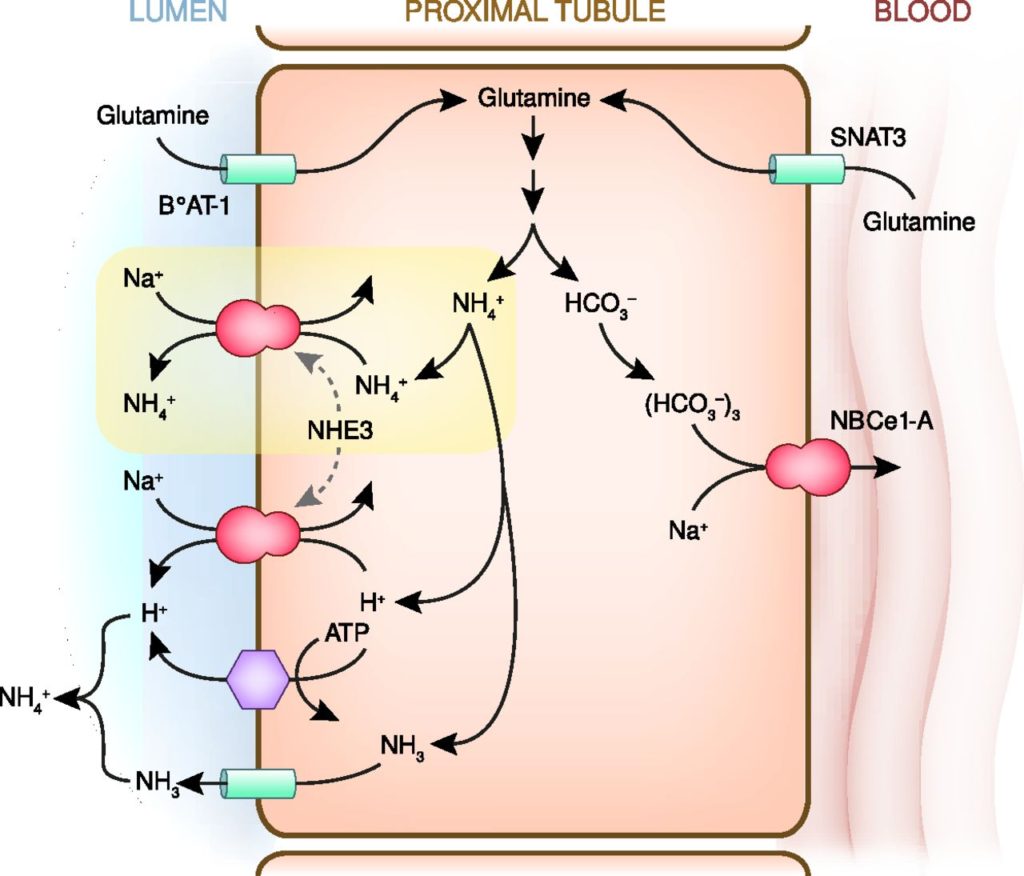
- Ammoniagenesis:
- Glutamine enters the peritubular cells of the PCT
- 80% from the peritubular capillaries
- 20% from the filtrate, reabsorbed by PCT
- Deaminated via Glutaminase (increased activity in acidosis)
- HCO3 reabsorbed into peritubular capillary blood
- NH4 excreted via Na/NH4 antiporter
- Glutamine enters the peritubular cells of the PCT
- Ammonium cycling:
- 80% reabsorbed TAL of LoH via replacing K on Na/K/2Cl cotransporter
- Increased conc in medullary intersitium
- Diffuses down conc grad into CD
- Buffer ability can be utilised to excrete H.
Pharmacology of Lactulose:
PC | Non-absorbable synthetic, non-digestible disaccharide. osmotic laxative |
PD | MOA: ↑ intraluminal osmotic pressure → retention of water in stool Abdminal cramping and flatulance |
PK | A: Not absorbed (PO BA 0%) D: Not applicable M: fermented by gut flora producing metabolites (such as acetate) which have osmotic and peristalsis-stimulating effects E: Faecal |
Gladwin 2016
Examiner Comments
2015B 16: 37.5% of candidates passed this question.
It was expected candidates would identify sources of ammonia (colon from metabolism of proteins, kidney, small amounts from breakdown of red blood cells and metabolism in muscles). The liver converts all circulating ammonia to urea (the urea cycle) (2NH3+CO2 = urea +H2O). Urea then undergoes enterohepatic circulation (25%) or is excreted by kidneys (75%). Ammonia (NH3) is lipid soluble and diffuses into the interstitial cell and tubular fluid by non-ionic diffusion where is buffers H+ to become non diffusible NH4+. No candidate mentioned enterohepatic circulation and most answers had very little detail on the metabolism and excretion and lacked depth.
Lactulose is a non absorbable synthetic, non-digestible disaccharide. It is an osmotic laxative fermented by gut flora producing metabolites (such as acetate) which have osmotic and peristalsis-stimulating effects, and methane causing in flatulence. Few could describe how lactulose decrease absorption of ammonia and a surprising number of people did not even state that lactulose was an osmotic laxative.
2023B 05
Outline the carbohydrate and lipid energy stores of the body (15% marks).
Outline the metabolic responses to starvation under the following headings: <24 hours; 24-72 hours; and >72 hours (85% marks).
CICMWrecks Answer
Body energy substrate reserves and utilisation
Fuel homeostasis principally regulates the needs of brain and muscle (major consumers of fuel).
brain – glucose exclusively (120g/d) until prolonged starvation (~2 days) then switches to ketones (fuel sparing)
Body energy substrate reserves:
- Glycogen stores → source of glucose (sufficient for brief period of fasting <24hrs)
- Liver (100 g; ½ day supply)
- Muscle (400 g)
- Brain (minimal; 4 mins supply)
- Fat stores (as TAG) → source of FFA and glycerol
- liver and adipose tissue
- Protein stores → source of a.a.
- muscle protein, plasma proteins, CT, enzymes
Body energy substrate utilisation:
- Brain/nervous tissue – 1°ly uses glucose for energy, but uses KBs with fasting
- Cardiac muscle – Use FFA, lactate and KB for energy
- RBC – 1°ly uses glucose for energy
- Skeletal muscle
- Uses FFA and ketone bodies for energy
- Glucose can be used for energy (esp with hyperglycaemia or anaerobi conditions (Eg. exercise)) or glycogen storage
- Adipose tissue – Uptake of glucose, glycerol and FFA → forms TAG for storage (lipogenesis); also breaks TAG into FFA/glycerol (lipolysis)
- Liver → integral role in starvation
- Control BSL by controlling glycogen stores and gluconeogenesis
- Takes up all metabolic fuel substrates → can interconvert them (Eg. gluconeogenesis, ketogenesis)
- Vital source of circulating metabolic fuel substrates
Glucose Homeostasis
PHASE I | Well-fed state |
PHASE II | Glycogenolysis |
PHASE III | Gluconeogenesis |
PHASE IV | Glucose, Ketone Body oxidation |
PHASE V | Fatty Acid, Ketone Body oxidation |
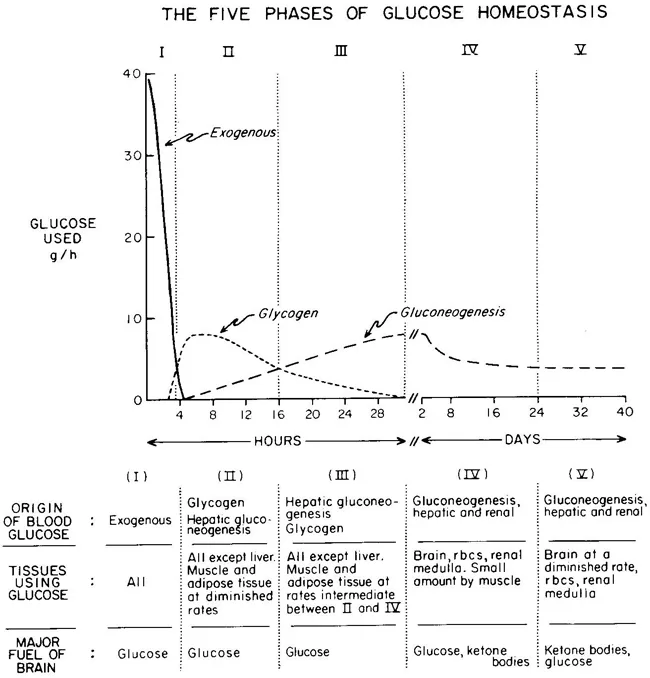
Metabolic responses to starvation
<24 hrs (early fasting)
- Initially Phase I and dietary glucose metabolized
- Phase II starts when dietary glucose exhausted
- ↓ Insulin
- Hepatic glycogenolysis maintains blood glucose
- Transient increase metabolic rate
24-72 hrs (short-term)
- Phase II starts when Glycogen stores exhaust around 20hrs
- depending on: feeding status, hepatic glycogen stores, physical activity
- ↓ Insulin ↑ Glucagon and ↑ catecholamine
- Brain and RBCs: Glucose from Hepatic Gluconeogenesis (from lactate, pyruvate, glycerol and alanine)
- Other (skeletal and cardiac muscle, kidney, liver): FFAs + glycerol (from Lipolysis / Hydrolysis of TGs)
- Metabolic rate begins to decrease after 48hrs
>72 hrs (Prolonged)
- ↓↓↓ Insulin ↑ Glucagon and ↑catecholamine
- Phase IV starts after several days of fasting
- Gluconeogenesis starts to decrease
- Ketone body accumulation increases
- Brain uses both glucose and ketone body for energy
- Further decline in metabolic rate 15-20%
- Phase V starts after prolonged fasting
- Less dependence on gluconeogenesis
- All tissues use Fatty acid and Ketone body oxidation for energy
- High ketone body conc, and glucose levels inhibit proteolysis (conservation of muscle) (protein sparing effect)
- After fat and ketone body exhausted → starvation → proteolysis of muscle
- Reduced energy expenditure
- reduced diet induced thermogenesis
- reduced weight and energy cost of movement
- decline in voluntary physical activity
Examiner Comments
2023B 05: 67% of candidates passed this question.
The first part of this question required the details of carbohydrate and lipid stores with their anatomical locations, biochemical forms, average amount of energy stored.
Under the metabolic responses to starvation, a detailed description was expected of major sources of energy production, associated biochemical processes and their transition from one process to another or one source to another over time, and the hormonal influences that govern this. A more detailed answer would also include organ specific energy utilisation under a starved state. Overall it was expected that a transition of glycogenolysis to gluconeogenesis to ketogenesis would be described. It would also be important to highlight how an initial protein conservation strategy transitions to eventual protein catabolism and how muscle glycogen, an important store of glucose is unavailable to maintain blood glucose concentrations in starvation.
iii. Define basal metabolic rate and outline the factors that influence it.
2023A 07 – 2013B 09 – 2008B 04
Define basal metabolic rate and list the factors that affect it. (60% of marks)
Describe the ways it may be measured. (40% of marks)
CICMWrecks Answer
Basal Metabolic Rate
- Energy expenditure required to maintain the body’s basic homeostatic mechanisms
- Normal BMR = 40kcal/m2/hr or approx. 70kcal/hr for 70kg male
- Relative organ contribution to BMR
- Liver 30%
- Brain 20%
- Muscle 20%
- Kidney 10%
- Heart 10%
- Other 10%
Factors that affect BMR
- Prandial effects
- Metabolic rate increases post-prandially for the digestion and absorption of nutrients
- After prolonged fasting metabolic rate decreases
- Thermoregulatory effects
- Temperatures above and below the thermoneutral zone cause metabolic rate to increase to support extra thermoregulatory mechanisms
- Response to heat
- Sweating
- Vasodilatation
- Response to cold
- Shivering
- Non-shivering thermogenesis
- Vasoconstriction
- Response to heat
- Temperatures above and below the thermoneutral zone cause metabolic rate to increase to support extra thermoregulatory mechanisms
- Physical activity
- Increased muscle activity
- Exercise increases metabolic rate in order to supply ATP to sarcomeres for contraction
- Increased muscle activity
- Inflammation
- Metabolic rate increases
- Chemotaxis
- Increased oxidative bursts
- Cell proliferation and clonal expansion
- Metabolic rate increases
- Psychological stress
- Hormonal factors
- Thyroid hormones → increase metabolic rate
- Maximal thyroid hormone activity increases MR 100%
- Thyroidectomy decreases MR 50%
- Catecholamines → increase metabolic rate
- Growth hormones → increase metabolic rate ~15~20%
- Testosterone → increase metabolic rate ~10~15%
- Thyroid hormones → increase metabolic rate
- Age
- Decreases with age >20
- Sex
- Male BMR > Female BMR possibly due to testosterone effect
- Body mass
- Muscle mass accounts for significant proportion of BMR, therefore increases BMR
- Sleep
- Decreases BMR 10~20%
Measurement of BMR
- Direct Calorimetry
- Atwater Chamber
- Subject placed in insulated chamber surrounded by H2O
- Change in temperature of water proprotional to metabolic rate
- Atwater Chamber
- Indirect calorimetry
- Metabolic carts
- Measurement of O2 consumption (and CO2, Urea production) to determine Metabolic rate
- Respiratory quotient
- Carbohydrates = 1
- Fats = 0.7
- Proteins = 0.8
- Metabolic carts
Conditions required to measure BMR
- Thermoneutral zone
- Free from psychological or physical stimulus
- After a night of restful sleep
- 12 hours after last meal
- 2 hours after last exercise
Sakurai 2016
Examiner Comments
2023A 07: 64% of candidates passed this question.
The first part of the question required candidates to define basal metabolic rate (BMR), identify the standardised conditions under which it is estimated and then elaborate on those conditions which affect it. Most candidates provided reasonable definitions but lost marks for not describing the standard conditions under which it is defined. Many were able to give a comprehensive list of factors that affected BMR however lacked the additional pertinent facts required in an outline question to achieve full marks. Candidates are again referred to the glossary to understand the difference between a list and outline question.
The second part of the question pertained to the measurement of BMR which was not as well answered and understood. Overall, there was a limited ability to discuss direct and indirect calorimetry clearly and some responses confused the two.
2013B 09: 12 candidates passed (44.4%).
Basal metabolic rate is the amount of energy liberated by catabolism of food per unit time, under standardised conditions. Knowledge of those conditions (e.g. after a night’s sleep, at rest and with no strenuous activity for at least 1 hour, a relaxed subject at a comfortable ambient temperature, at least 12 hours after a meal) was weak. Factors affecting (e.g. body temperature, various hormones, malnutrition, pregnancy, drugs, disease, etc.) it was often incomplete.
Knowledge of measurement was very poor. Candidates were expected to describe the direct (e.g. by using a whole body calorimeter) and indirect (e.g. measuring oxygen consumption) methods.
2008B 04: 3 (60%) candidates passed this question
Metabolism is all the chemical reactions in all the cells of the body. Metabolic rate is usually described in terms of rate of heat liberation. Candidates often confused basal with metabolism during activity.
A good answer was expected to outline conditions under which basal metabolic rate is measured (no food for 12 hours, after a night of restful sleep, person is at rest in reclining position for 30 minutes, all psychic or physical factors that cause excitement eliminated, at a controlled comfortable temperature), and the mention of and an explanation of the methods by which metabolic rate is measured (eg direct and indirect calorimetry). Any validated technique of determining the BMR was rewarded with marks, with oxygen consumption methods being the easiest to explain. No candidate mentioned any ‘normal’ value for the BMR.
Candidates were expected to briefly mention and explain how factors affecting metabolic rate (eg exercise, specific dynamic action of protein after a meal is ingested,, age, thyroid hormone, sympathetic stimulation, fever, gender, hormonal, climate, sleep, chronic malnutrition, trauma, inflammatory response, etc).
Questions with more than one part will have an indication of the proportion of the total mark that will be allocated to each part. It is important that candidates apportion their allocated time and content accordingly.
Syllabus: K 2a, b
Reference Text: Guyton Chp72
iv. Describe the measurement of metabolic rate.
2023A 07 – 2013B 09 – 2008B 04
Define basal metabolic rate and list the factors that affect it. (60% of marks)
Describe the ways it may be measured. (40% of marks)
CICMWrecks Answer
Basal Metabolic Rate
- Energy expenditure required to maintain the body’s basic homeostatic mechanisms
- Normal BMR = 40kcal/m2/hr or approx. 70kcal/hr for 70kg male
- Relative organ contribution to BMR
- Liver 30%
- Brain 20%
- Muscle 20%
- Kidney 10%
- Heart 10%
- Other 10%
Factors that affect BMR
- Prandial effects
- Metabolic rate increases post-prandially for the digestion and absorption of nutrients
- After prolonged fasting metabolic rate decreases
- Thermoregulatory effects
- Temperatures above and below the thermoneutral zone cause metabolic rate to increase to support extra thermoregulatory mechanisms
- Response to heat
- Sweating
- Vasodilatation
- Response to cold
- Shivering
- Non-shivering thermogenesis
- Vasoconstriction
- Response to heat
- Temperatures above and below the thermoneutral zone cause metabolic rate to increase to support extra thermoregulatory mechanisms
- Physical activity
- Increased muscle activity
- Exercise increases metabolic rate in order to supply ATP to sarcomeres for contraction
- Increased muscle activity
- Inflammation
- Metabolic rate increases
- Chemotaxis
- Increased oxidative bursts
- Cell proliferation and clonal expansion
- Metabolic rate increases
- Psychological stress
- Hormonal factors
- Thyroid hormones → increase metabolic rate
- Maximal thyroid hormone activity increases MR 100%
- Thyroidectomy decreases MR 50%
- Catecholamines → increase metabolic rate
- Growth hormones → increase metabolic rate ~15~20%
- Testosterone → increase metabolic rate ~10~15%
- Thyroid hormones → increase metabolic rate
- Age
- Decreases with age >20
- Sex
- Male BMR > Female BMR possibly due to testosterone effect
- Body mass
- Muscle mass accounts for significant proportion of BMR, therefore increases BMR
- Sleep
- Decreases BMR 10~20%
Measurement of BMR
- Direct Calorimetry
- Atwater Chamber
- Subject placed in insulated chamber surrounded by H2O
- Change in temperature of water proprotional to metabolic rate
- Atwater Chamber
- Indirect calorimetry
- Metabolic carts
- Measurement of O2 consumption (and CO2, Urea production) to determine Metabolic rate
- Respiratory quotient
- Carbohydrates = 1
- Fats = 0.7
- Proteins = 0.8
- Metabolic carts
Conditions required to measure BMR
- Thermoneutral zone
- Free from psychological or physical stimulus
- After a night of restful sleep
- 12 hours after last meal
- 2 hours after last exercise
Sakurai 2016
Examiner Comments
2023A 07: 64% of candidates passed this question.
The first part of the question required candidates to define basal metabolic rate (BMR), identify the standardised conditions under which it is estimated and then elaborate on those conditions which affect it. Most candidates provided reasonable definitions but lost marks for not describing the standard conditions under which it is defined. Many were able to give a comprehensive list of factors that affected BMR however lacked the additional pertinent facts required in an outline question to achieve full marks. Candidates are again referred to the glossary to understand the difference between a list and outline question.
The second part of the question pertained to the measurement of BMR which was not as well answered and understood. Overall, there was a limited ability to discuss direct and indirect calorimetry clearly and some responses confused the two.
2013B 09: 12 candidates passed (44.4%).
Basal metabolic rate is the amount of energy liberated by catabolism of food per unit time, under standardised conditions. Knowledge of those conditions (e.g. after a night’s sleep, at rest and with no strenuous activity for at least 1 hour, a relaxed subject at a comfortable ambient temperature, at least 12 hours after a meal) was weak. Factors affecting (e.g. body temperature, various hormones, malnutrition, pregnancy, drugs, disease, etc.) it was often incomplete.
Knowledge of measurement was very poor. Candidates were expected to describe the direct (e.g. by using a whole body calorimeter) and indirect (e.g. measuring oxygen consumption) methods.
2008B 04: 3 (60%) candidates passed this question
Metabolism is all the chemical reactions in all the cells of the body. Metabolic rate is usually described in terms of rate of heat liberation. Candidates often confused basal with metabolism during activity.
A good answer was expected to outline conditions under which basal metabolic rate is measured (no food for 12 hours, after a night of restful sleep, person is at rest in reclining position for 30 minutes, all psychic or physical factors that cause excitement eliminated, at a controlled comfortable temperature), and the mention of and an explanation of the methods by which metabolic rate is measured (eg direct and indirect calorimetry). Any validated technique of determining the BMR was rewarded with marks, with oxygen consumption methods being the easiest to explain. No candidate mentioned any ‘normal’ value for the BMR.
Candidates were expected to briefly mention and explain how factors affecting metabolic rate (eg exercise, specific dynamic action of protein after a meal is ingested,, age, thyroid hormone, sympathetic stimulation, fever, gender, hormonal, climate, sleep, chronic malnutrition, trauma, inflammatory response, etc).
Questions with more than one part will have an indication of the proportion of the total mark that will be allocated to each part. It is important that candidates apportion their allocated time and content accordingly.
Syllabus: K 2a, b
Reference Text: Guyton Chp72
v. Describe anaerobic metabolism and ketone production.
2020A 08
Describe the production, metabolism and role of lactate.
2015B 06
Describe the formation and the metabolic fate of lactate. Outline its role in energy production.
2010A 05
Describe the production and metabolism of lactate.
CICMWrecks Answer
Lactate is the conjugate base of lactic acid (an organic 3-C acid)
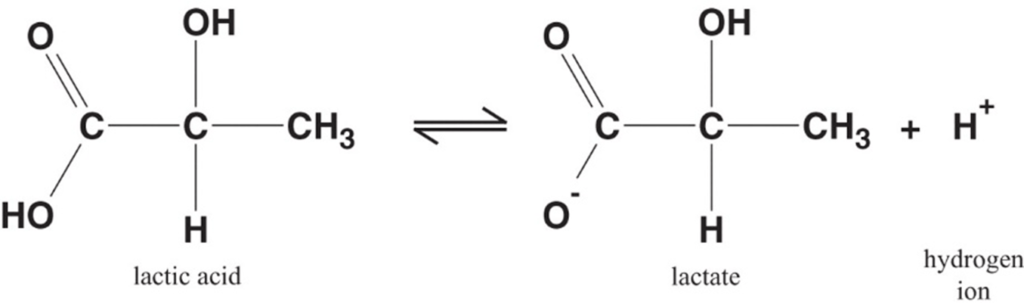
Production:
- It is produced by anaerobic metabolism of pyruvate either:
- Physiologically → in RBC (no mitochondria), renal medulla (↓ PO2), cornea/ lens (↓ PO2) → hence, normal plasma [lactate] is 0.5-2 mmol/L (and NOT zero!)
- Pathologically → reduced tissue perfusion and/or O2 delivery (Eg. shock, hypoxaemia) → thus, plasma [lactate] ↑↑↑ (> 2 mmol/L)
- Plasma [lactate] is 0.5 – 2 mM (and NOT zero) due to physiological production → it can be measured clinically as an indicator of anaerobic metabolism (Ie. ↑ anaerobic metabolism due to pathological situations lead to ↑ [lactate])
Fate of lactate:
- Persistent anaerobic metabolism (Ie. ongoing hypoxia) causes an accumulation of cellular lactate → this diffuses out of the cell into plasma along its [ ] gradient → lactate can then be:
- Used as a fuel source by the heart and brain
- Transported to the liver where it is:
- Converted back to glucose via gluconeogenesis (requires 6x ATP), which is then transported back peripherally for use → “Cori cycle”
- Converted to pyruvate intermediate → utilised locally in TCA cycle for ATP production via oxidative phosphorylation
- Resolution of hypoxia (Ie. tissue O2 tension restored) → intracellular lactate can be oxidised back to pyruvate for use in local tissue aerobic metabolism (Ie. fed into TCA cycle)
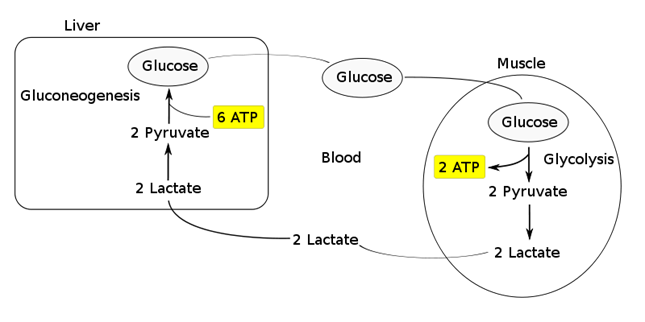
Functions of Lactate:
Lactate Sink:
- Lactate acts as a sink in heart, liver, muscle etc, allowing a period of ongoing ATP production from glycolysis when:
- cells become oxygen deplete
- Kreb’s cycle is inhibited
- Other causes of pyruvate accumulation: circulating catecholamines, exercise, sepsis or lack of mitochondria (RBCs)
Lactate Shuttle:
- Intracellular shuttle:
- Lactate may be shuttle out of:
- mitochoncrial membrane
- peroxisomes
- into cytoplasm of myocytes, neurons, astrocytes
- Oxydised by cytoplasmic LDH to pyruvate, generating NADH for energy use
- Lactate may be shuttle out of:
- Intercellular shuttle:
- Excess lactate, formed within fast-twitch fibres, is transported to other cells within the body with the oxidative capability to metabolize lactate, such as type I (slow-twitch) muscle cells, enhancing their excitability and limiting fatigue
- Furthermore, once in circulation, lactate attaches to red blood cells (RBCs) and is disassociated in the liver where inter-conversion via gluconeogenesis facilitates glucose formation, providing an alternative aerobic energy source.
Lactate as a signaling molecule:
- Redox signaling by intracellular shuttles
- Gene expression
- Increased intracellular levels of lactate can act as a signalling hormone, inducing changes in gene expression that will upregulate genes involved in lactate removal, and stimulates mitochondrial biogenesis.
- Control of lipolysis
- the shuttle regulates FFA mobilization by controlling plasma lactate levels.
- lactate functions to inhibit lipolysis in fat cells through activation of an orphan G-protein couple receptor (GPR81) that acts as a lactate sensor, inhibiting lipolysis in response to lactate
Bianca / JC 2021
Examiner Comments
2020A 08: 16% of candidates passed this question.
Better answers used the categorisation in the question as a structure for their answer. Many candidates gave a good description of lactate production from glycolysis, increasing with accumulation of NADH and pyruvate, when these are unable to enter Krebs cycle. There were however, many vague and incorrect descriptions as to what lactate is and its physiological role. Many candidates suggested that its presence is abnormal or pathological. Most answers demonstrated a superficial understanding and physiological detail of lactate’s role as an energy currency in times of oxygen debt. Higher scoring candidates often mentioned non-hypoxic causes of pyruvate accumulation which include; circulating catecholamines, exercise, sepsis or lack or mitochondria (RBCs). Mention of the relative ATP production of the two fates of pyruvate was also noted in more complete answers. The Cori cycle was generally superficially described. A key role of lactate is the ‘lactate sink’, allowing a period of ongoing ATP production from glycolysis when cells become oxygen deplete or the Kreb’s cycle is inhibited; few candidates detailed or highlighted this.
2015B 06: 21% of candidates passed this question.
It was expected that the answer would include comments on lactate generation from glucose via pyruvate and the metabolic linkage of nicotinamide adenine dinucleotide (NAD). Lactate regenerates NAD+ (pyruvate is reduced to lactate while NADH is oxidized to NAD+ ). The citric acid cycle and the electron transport chain occur in the mitochondria of cells, and will only proceed in the presence of oxygen.
One molecule of glucose produces 2 ATP anaerobically (pyruvate to lactate) vs 26 aerobically (pyruvate enters TCA cycle) .Total production is about 1500 mmols/day with blood levels resting value of 1–1.5 mmol/L to a peak of 10–15 mmol/L.
Lactate can be used in 3 ways:
1. Conversion to glucose via gluconeogenesis in the liver and release back into circulation (Cori cycle). This is the fate of 80 % circulating lactate from tissues low in oxygen (e.g. exercising muscle with low pO2) or red blood cells (no mitochondria). The production from glucose in RBC’s is the Embden-Meyerhoff pathway.
2. Consumed as a fuel e.g. heart (20% of circulating lactate)
3. Mitochondria and oxygen Oxidation back to pyruvate by well-oxygenated muscle cells, heart cells, and brain cells pyruvate is then directly used to fuel the Krebs cycle (generating 28 mmols ATP)
Lactate generation from muscle is increased with B1 mediated stimulation e.g. from adrenalin. This topic is well covered in Power and Kam Principles of Physiology for the Anaesthetist, 3rd Edition, although some of the details are in several different sections.
Most candidates showed some understanding of the role of glucose in the production of pyruvate to lactate. However, the differential ATP production, the role of NADH availability and how oxygen and the role of mitochondria were involved was less well handled. Better answers described the normal generation of lactate in some tissues (e.g. RBC) and role of muscle and liver in metabolism back to glucose (Cori cycle) and the role of lactate as a metabolic substrate in some organs. Marks were awarded for normal production values and blood levels.
2010A 05: 5 (50%) of candidates passed this question
Lactate is constantly produced from pyruvate via the enzyme lactate dehydrogenase (LDH). Lactate is produced during normal metabolism and in increased quantities during anaerobic metabolism. Candidates were expected to further describe this physiological processes.
A good answered required quantification of lactate production during normal aerobic metabolism, during anaeorobic metabolism, the pathways involved (Glucose to Pyruvate, Pyruvate to Citric Acid cycle in presence of Oxygen, Pyruvate + NADH to Lactate + NAD+ without Oxygen) associated ATP production, site of intracellular production, why red blood cells differ and lactate metabolism (eg oxidation to pyruvate by well-oxygenated muscle cells which is then directly used to fuel the citric
acid cycle conversion to glucose via the Cori cycle in the liver through the process of gluconeogenesis). Good answers illustrated the loss of energy potential with the production of lactate and discussed the situations that would lead to an inbalance between production and metabolism of lactate.
Candidates who did poorly in this question did so due to a lack of depth and breadth for this topic. For example, even though the Cori Cycle was often mentioned, it was poorly described in relation to lactate metabolism.
Syllabus: K2g
References: Textbook of Medical Physiology, Guyton Chp 67
2018B 17
Describe ketone bodies including their synthesis and metabolism.
CICMWrecks Answer
Ketone bodies
Introduction:
- Ketone bodies are three water-soluble molecules (acetoacetate, beta-hydroxybutyrate, and their spontaneous breakdown product, acetone) containing the ketone group
- provide an alternative source of substrate for energy production
- Ketones can only be produced by the liver, and only used as a substrate by the kidney, as well as skeletal and cardiac muscle
- Production of ketones is accelerated by glucagon and adrenaline
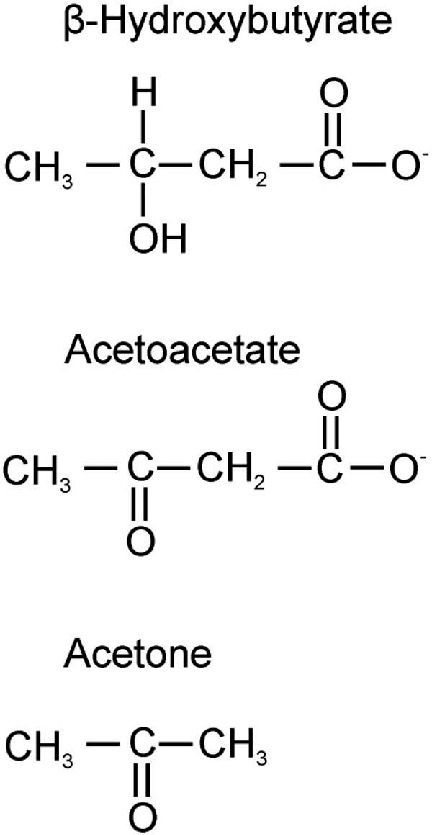
Ketogenesis:
- They are produced by the mitochondria in liver cells from fatty acids
- β-oxidation of fatty acids in the liver produces acetyl-CoA
- Acetyl-CoA usually enters the citric acid cycle to produce ATP
- When large amounts of acetyl CoA are produced, they may instead condense to form acetoacetate, which can then be reduced to β-hydroxybutyrate (These three molecules are called ketone bodies)
Ketone bodies
- Acetoacetate: When two acetyl-CoA molecules lose their -CoAs, (or Co-enzyme A groups) they can form a (covalent) dimer called acetoacetate.
- Beta-hydroxybutyrate: Beta-hydroxybutyrate is a reduced form of acetoacetate, in which the ketone group is converted into an alcohol (or hydroxyl) group. Both are 4-carbon molecules, that can readily be converted back into acetyl-CoA by most tissues of the body, with the notable exception of the liver.
- Acetone: Acetone is the decarboxylated form of acetoacetate which cannot be converted back into acetyl-CoA except via detoxification in the liver where it is converted into lactic acid, which can, in turn, be oxidized into pyruvic acid, and only then into acetyl-CoA.
Circumstances:
- Ketogenesis takes place in the setting of low glucose levels in the blood, after exhaustion of other cellular carbohydrate stores, such as glycogen.
- low food intake (fasting), carbohydrate restrictive diets, starvation, prolonged intense exercise, alcoholism
- State of Low insulin and high glucagon is what stimulates the liver
- It can also take place when there is insufficient insulin (e.g. in type 1 (but not 2) diabetes), particularly during periods of “ketogenic stress” such as intercurrent illness.
- untreated (or inadequately treated) type 1 diabetes mellitus.
Transport:
- Ketone bodies are readily transported into tissues outside the liver and converted into acetyl-CoA, which then enters the citric acid cycle and is oxidized in the mitochondria for energy.
- In the brain, ketone bodies are also used to make acetyl-CoA into long-chain fatty acids.
Metabolism
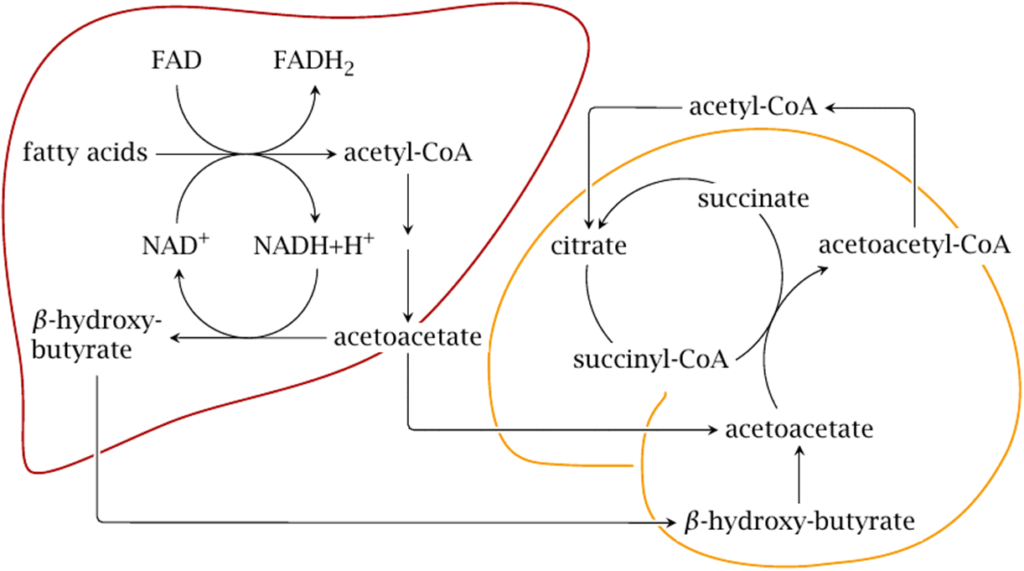
- Ketone bodies can be utilized as fuel in the heart, brain and muscle, but not the liver. They yield 2 GTP and 22 ATP molecules per acetoacetate molecule when oxidized in the mitochondria.
- Liver: cannot use them for energy because it lacks the enzyme thiophorase (β-ketoacyl-CoA transferase). Acetone is taken up by the liver in low concentrations and undergoes detoxification through the methylglyoxal pathway which ends with lactate. Acetone in high concentrations, as can occur with prolonged fasting or a ketogenic diet, is absorbed by cells outside the liver and metabolized through a different pathway via 1,2-propanediol. Though the pathway follows a different series of steps requiring ATP, 1,2-propanediol can eventually be turned into pyruvate
- Heart: Preferentially utilizes fatty acids as fuel under normal physiologic conditions. However, under ketotic conditions, the heart can effectively utilize ketone bodies for this purpose
- Brain:
- The brain gets a portion of its fuel requirements from ketone bodies when glucose is less available than normal (e.g., during fasting, strenuous exercise, low carbohydrate, ketogenic diet and in neonates).
- In the event of a low glucose concentration in the blood, the brain has an obligatory requirement for some glucose.
- After the diet has been changed to lower blood glucose utilization for 3 days, the brain gets 25% of its energy from ketone bodies.
- After about 24 days, ketone bodies become the major fuel of the brain, making up to two-thirds of brain fuel consumption.
Examiner Comments
2018B 17: 35% of candidates passed this question.
Whilst most candidates understood that ketones provided an alternative source of substrate for energy production, many lacked a basic understanding of their synthesis and metabolism.
Important facts included what ketone bodies are, where they were synthesised, where they were taken up and used as fuel, under what circumstances they are used and the integral role of insulin. Many candidates accurately reproduced the glycolytic and/or the TCA cycle, but this was not being examined, and did not score additional marks. Many candidates incorrectly stated that ketone production was the result of anaerobic metabolism.
vi. Describe the pharmacology of enteral and parenteral nutrition.
2022B 06
Outline the dose (10% marks), composition (75% marks) and side effects (15% marks) of enteral feeds.
CICMWrecks Answer
Enteral feeding in critically ill
- Primary goal to alter course and outcome of critical illness
- Major goals determined by following principles:
- Catabolism > anabolism. Calorie consumption reduced with better management of critical illness
- Carbohydrates preferred as fat mobilization impaired
- Protein: mitigate breakdown of muscle protein into AA
- Recovery phase: Anabolism>catabolism. Nutritional support provides substrate for anabolic state
Dose
- Mainly observational evidence and clinical experience
- Adjustments can be made based upon an estimate of individuals’s Resting Energy Expenditure (REE) or their body weight
- Adjustment based on body weight, variations based on age, using equations or indirect calorimetry
- Indirect calorimetry
- better than REE equations
- not routinely accessible, not adequately tested
- Predictive equations significant inaccuracy
- Dosing weight is hence routinely based on body weight / BMI with specific adjustments
Dosing weight
- Estimate current or pre-admission dry body weight
- Choose appropriate body weight based on BMI from which to calculate caloric and protein intake
- Underweight (BMI <18.5 kg/m2): current weight as initial dosing weight. Ideal body weight could lead to excess initial calories and induce refeeding syndrome
- Normal weight (BMI 18.5-24.9 kg/m2): current weight as dosing weight
- Overweight (BMI 24.9-29.9 kg/m2): current weight as dosing weight
- Obese (BMI >= 20 kg/m2): Adjusted body weight
- Ideal Body Weight (Devine formula):
- IBW (men) = 50 kg + 2.3 kg x (height, in – 60)
- IBW (women) = 45.5 kg + 2.3 kg x (height, in – 60)
- Adjusted Body Weight
- AdjBW = IBW + (0.4 * [Actual weight – IBW])
- or AdjBW = 1.1 x IBW
- AdjBW = IBW + (0.4 * [Actual weight – IBW])
DAILY NUTRITIONAL REQUIREMENTS
Nutrient | Requirement |
---|---|
H2O | 30-40 mL/kg/day |
Energy | 25-30 kcal/kg/day (can ↑ up to 1.5-1.75x with disease/stress) |
Nitrogen | 0.5 g/kg/day (can ↑ to 1-1.5 g/kg/day with disease/stress) |
Glucose | 3 g/kg/day |
Lipid | 2 g/kg/day |
Na+ | 1-2 mmol/kg/day |
K+ | 0.7-1 mmol/kg/day |
Cl- | 1 mmol/kg/day |
Ca2+ | 0.1 mmol/kg/day |
Mg2+ | 0.1 mmol/kg/day |
PO4 | 0.1 mmol/kg/day |
BUT these vary according to:
- Physiological factors:
- Age, gender, body size
- Pregnancy
- Activity level
- Hydration status
- Pathological factors
- Burns and sepsis → ↑ protein and caloric intake
- Renal failure → ↓ volume, protein and electrolyte (esp K+) content
- CCF → fluid reduction
- Hepatic failure → ↓ a.a/protein content to prevent encephalopathy
- Respiratory failure → ↓ glucose to minimise CO2 production
- Calories
- Fewer calories in the first week of critical illness: approx 8-10 kcal/kg/day
- 25-30 kcal/kg/day in stable patient
- upto 35 kcal/kg/day if weight gain is desired in stable patient and in a lower inflammatory state
- 25kcal/kg/day or less if extubation is imminent
- Protein
- Mild-mod Critical illness: 0.8-1.2 g/kg/day
- Severe Critical Illness: 1.2-1.5kg/day
- Severe burns: upto 2g/kg/day
Composition
FORMULATIONS:
- Common differences between formulas include osmolarity, caloric density, and amount of protein per calorie, as well as electrolyte, vitamin, and mineral content.
- Most are formulated to provide 100 percent of recommended daily vitamin and mineral dose when a minimum of approximately 1000 or more kilocalories are delivered per day.
STANDARD
- Isotonic to serum
- Caloric density of approximately 1 kcal/mL
- Lactose-free
- Intact (nonhydrolyzed) protein content of about 40 g/1000 mL (40 g/1000 kcal)
- Nonprotein calorie to nitrogen ratio of approximately 130
- Mixture of simple and complex carbohydrates
- Long-chain fatty acids (although some are now including medium-chain and omega-3 fats)
- Essential vitamins, minerals, and micronutrients (usually RDA provided in ~ 1000ml of feed)
OTHERS
- CONCENTRATED
- useful in fluid restriction
- mildly hyperosmolar
- caloric density of 1.2,1.5 or 2.0 kcal/ml
- no mortality or morbidity benefit
- PREDIGESTED
- protein hydrolyzed to short-chain peptides, less complex carbohydrates
- reduced total fat, increased MCT or altered TGs
- weakly supported by data
- Potential benefit in: Thoracic duct leak/chylothorax, digestive defects/malabsorbtion syndromes, failure to tolerate standard EN
- Caloric density of 1 or 1.5 kcal/ml
COMPOSITION
- CARBOHYDRATE/FAT
- Standard 49-53% / 29-30%
- Other (not recommended for routine EN):
- Low carb/high fat 28-40%/40-55%
- high carb/low fat 85%/15%
- PROTEIN
- High protein EN (1.2-2g/kg/day) – improved mortality
- Acute/chronic renal disease: Low-protein EN not recommended for routine use. Renal formulas only if difficult to manage volume and electrolytes
- Standard feeds for patients on CRRT
- Peptides – unlikely to be beneficial
- Omega-3 fatty acids and antioxidants, glutamine, probiotics/prebiotics – unlikely to be beneficial, and may be harmful
- Fiber
- no benefit in diarrhoea prevention.
- Mixed fibre feeds may help in persistent diarrhoea
- avoid in pressors/ reduced mobility due to bezoars
- Vitamins and trace elements
- included in feed upto RDA amounts
- Routine supplementation usually not necessary. Definitely donot provide more than RDA
Side Effects
Complication | Mechanism | Management | |
---|---|---|---|
Aspiration | higher risk of pneumonia – causality poorly established | – backrest elevation, post-pyloric feeding – PEG – motility agents (poor evidence in solely reducing aspiration) | |
Diarrhoea | – 15-18% (vs 6% without EN) – alteration of intestinal transit or microflora – commonly associated with medications that can cause diarrhoea (eg antibiotics, PPIs) or suspensions in non-absorbable sugars like sorbitol | – Removal of potential culprit agents – Fiber (use with caution in impaired peristalsis, pressors) | |
Metabolic | Hyperglycaemia | glucose content, insulin resistance | – correction |
Micronutrient deficiencies | usually in malnutrition or GI/renal losses | – supplementation | |
Refeeding syndrome | – rapid changes in fluids and electrolytes – glucose causes release of insulin and intracellular shift of electrolytes – insulin also causes ATP and 2,3-DPG production which utilises phosphate stores – predominantly hypophosphatemia (incl. CV collapse, resp failure, rhabdomyolysis, seizures, delirium) – hypokalemia, hypomagnesemia – Higher risk in acute weight loss, and rapid weight restoration | – reduce nutritional support – aggressive correction of electrolytes | |
Fluid/water | – ~20ml/kg water – potential for water deficit | – assess hydration status – water flushes/ replacement as necessary | |
Mechanical | Constipation | – in feeds with fiber – esp when peristalsis is impaired – impaction, distention, perforation, death | – Careful monitoring and appropriate management |
Fecal impaction | |||
Fiber bezoars | |||
Other | Device related complications | PEG/NG complications related to insertion, dislodgement, mechanical complications |
Examiner Comments
2022B 06: 60% of candidates passed this question.
Generally, most candidates had a reasonable approach to structuring their answers using the headings provided in the question. To score well candidates were required to outline a method of dosing (per body weight, variations based on age, using equations or indirect calorimetry) and describe the composition in terms of the macronutrient daily requirements and energy content as well as other included components (micronutrients). Very few candidates discussed the variations in formulations beyond concentration change nor the reasons for such variations in sufficient detail. Candidates that did not score well were often lacking in detail or missing sections such as side effects or significant elements of the composition. No marks were awarded for content related to the presence of delivery tubes, administration details (e.g. definitions of gastric tolerance, trophic feeding, commencement rate) or for details that related specifically to parenteral nutrition.
2021A 04
Outline the dose (10% marks), composition (60% marks) and side effects (30% marks) of total parenteral nutrition (TPN).
CICMWrecks Answer
Overview of parental nutrition
- Parental nutrition is the delivery of nutrients into venous circulation (peripherally or centrally) instead of the enteral route → it can be used to supplement nutrient delivery via enteral route, or supplement nutrient delivery in its entirety (total parental nutrition)
- It is prepared by sterile technique → typically a 3L bag of hyperosmolar solution (containing glucose, amino acids, lipids, H2O, electrolytes, vitamins and trace elements)
- It can only be administered by CVC due to its ↑ tonicity (Nb. issues with irritation and thrombosis if given via PIVC) → infused over 24 hrs
- It also requires multidisciplinary team involvement
Indications for parental nutrition
- Indicated for those unable to ingest or digest nutrients or to absorb them from GI tract for a prolonged period of time (> 3-4 days) → includes those who have:
- A failed trial of enteral feeding
- Contraindications to enteral nutrition:
- GI obstruction
- Upper GI strictures and fistulae (Eg. enterocutaneous fistulae)
- Severe pancreatitis
- Prolonged ileus (Eg. major abdominal surgery where feeding not anticipated for days, paralytic ileus)
- Inflammatory conditions (Eg. IBD or mucositis due to chemotherapy)
- Short gut syndromes (Eg. major SB resection)
Daily nutritional requirements for parental nutrition
Nutrient | Requirement |
---|---|
H2O | 30-40 mL/kg/day |
Energy | 25-30 kcal/kg/day (can ↑ up to 1.5-1.75x with disease/stress) |
Nitrogen | 0.5 g/kg/day (can ↑ to 1-1.5 g/kg/day with disease/stress) |
Glucose | 3 g/kg/day |
Lipid | 2 g/kg/day |
Na+ | 1-2 mmol/kg/day |
K+ | 0.7-1 mmol/kg/day |
Ca2+ | 0.1 mmol/kg/day |
BUT these vary according to:
- Physiological factors:
- Age, gender, body size
- Pregnancy
- Activity level
- Hydration status
- Pathological factors
- Burns and sepsis → ↑ protein and caloric intake
- Renal failure → ↓ volume, protein and electrolyte (esp K+) content
- CCF → fluid reduction
- Hepatic failure → ↓ a.a/protein content to prevent encephalopathy
- Respiratory failure → ↓ glucose to minimise CO2 production
Components of parental nutrition
- (1) Energy source → glucose:lipid mixture (60:40 or 50:50)
- Glucose (as 50% dextrose)
- Used as an energy substrate → provides 50% of daily caloric needs
- Glucose has ↓ energy density → 3.4 kcal/g only (cf. 4 kcal/g for carbohydrates) → 50% dextrose needs to be given (1 L = 940 kcal) → BUT this is hypertonic (1900 mosm/L) and requires delivery via CVC
- Insulin can be given to aid glucose utilisation (esp in stressed patients)
- Need to avoid excess glucose delivery → risk of ↑ BGL, lipogenesis (liver disease), ↑ MR and ↑ CO2 production (delayed ventilatory wean)
- Lipid (as 10% or 20% intralipid)
- Used as (i) an energy substrate (provides 30-40% daily caloric needs), and (ii) provision of essential FAs (vital for cell membranes and PG synthesis)
- ↑ energy density cf. glucose → 1.1 kcal/mL (10%) and 2 kcal/mL (20%) → thus 1000 kcal can be achieved by 500 mL of 20% or 1L of 10%
- Can be infused separately (Ie. not mixed with glucose/a.a. solution) → can be given 1x/week BUT usually given daily to aid caloric delivery in the smallest volume of solution –
- Glucose (as 50% dextrose)
- Nitrogen-source (as symthamin 17)
- Used for (i) tissue protein synthesis (Eg. enzymes) and (ii) caloric needs
- Includes > 50% essential a.a.’s (isoleucine, leucine, valine, lysine, methionine, phenylalanine, threonine, tryptophane) and > 25% branched chain a.a
- Nb. A.a. are all L-isomers (as the body cannot use D-isomers)
- H2O → to maintain body H2O balance and replace losses (Eg. dehydration, bleeding)
- Electrolytes (Na+ /K+ /Cl- /PO4 3-/Mg2+, Ca2+) → to maintain and replace losses
- Vitamin/trace elements → vital to enzyme systems and metabolic pathways in body
- Vitamin solutions – both H2O and fat-soluble vitamins (esp folic, thiamine, vitamin K)
- Trace elements (Zn, Fe, Cu, Mn, Co, Se, I, Cr, Mb)
Monitoring of parental nutrition
- Needed to assess for (i) progress of nutritional state and (ii) complications of therapy
- Involves:
- Clinical r/v (Eg. fluid balance, weight, nutritional assessment, infections)
- Frequent ward glucose testing (until BGL stabilises)
- Investigations → daily EUC, CMP, BGL; weekly FBC, LFT (incl albumin), Coags, Lipid studies, and plasma/urine osmolality
Complications of parental nutrition
- Catheter-related (MOST common) → PTX, chylothorax, embolism (air/thrombus), infection, Etc.
- Fluid/electrolyte disturbances
- Fluid overload or hyperosmolar dehydration
- Electrolyte disturbances
- Normal AG metabolic acidosis (due to ↑ Cl- content)
- Metabolic disturbances
- Hyperglycaemia (initially) → delay in ↑ endogenous insulin (thus supplemental insulin required)
- Rebound hypoglycaemia (with abrupt cessation of TPN) → due to ↑ levels of endogenous insulin
- Metabolic bone disease
- Others:
- Immune suppression (due to fat component)
- Liver disease → initially deranged LFTs → later fatty liver/steatohepatitis
- ↑ PaCO2 (due to excessive glucose metabolism)
Bianca
Examiner Comments
2021A 04: 59% of candidates passed this question.
The pharmacology of enteral and parenteral nutrition is a level 1 topic in the first part syllabus. The TPN dose in terms of daily calorie and other nutritional requirements were key expectations in first part of the question. A detailed list of all macro and micronutrients was required under TPN composition. Expected information about macronutrients were their forms in the TPN solution (e.g., carbohydrate in the form of glucose, protein in the form amino acids), their relative calorie contributions and their essential components (e.g., the names of the essential amino acids). Identification of potential variability in composition and dose based on specific patient factors scored extra marks. Side effects included metabolic derangements (refeeding syndrome, over or under-feeding, hyperglycaemia, hyperlipemia), biochemical disturbances (fluid and electrolyte imbalances), organ injury (liver, pancreas) and vascular access related complications. Limited breadth and depth of information as well as incorrect facts were prevalent in the answers that scored lower marks.
VIVAs
2023B | Daily nutritional requirement for healthy aduly, nutrition, endocrine pharmacology |
2023A | |
2022B | Carbohydrate metabolism, factors that increase insulin secretion Carbohydrate metabolism, factors that increase insulin secretion |
2022A | |
2021B | Carbohydrate breakdown, absorption, glucose metabolism |
2021A | |
2020B | |
2019B | Basal metabolic rate, thyroid hormone |
2019A | Caloric requirement for 65 yo female |
2018B | |
2018A | |
2017B | |
2017A | |
2016B | |
2016A | |
2015B | |
2015A | nutrition and metabolism |
2014B | |
2014A | |
2013B | |
2013A | |
2012B | |
2012A | |
2011B | |
2011A | |
2010B | |
2010A | |
2009B | |
2009A | |
2008B | |
2008A | |
2007B |
Recent Comments