Syllabus (Fourth Edition, 2023)
Topics
H1: Renal Physiology
i. Describe the functional anatomy of the kidneys.
ii. Describe renal blood flow and its regulation.
iii. Describe glomerular filtration and tubular function.
iv. Explain the counter-current mechanisms in the kidney.
v. Describe the functions of the kidney.
vi. Describe the physiological effects of renal dysfunction.
H2: Applied Renal Physiology
i. Describe the principles of dialysis and filtration.
H3: Renal Pharmacology
i. Understand the pharmacology of diuretics.
ii. Understand the pharmacology of renal replacement fluid.
H4: Renal Measurement
i. Describe the principles of measurement of glomerular filtration rate and renal blood flow.
ii. Describe the utility of biochemical estimates of renal function (including but not limited to the measurement of serum creatinine, cystatin C and estimates of Creatinine Clearance such as eGFR).
Topics not covered in previous SAQs
H4: Renal Measurement
i. Describe the principles of measurement of glomerular filtration rate and renal blood flow.
Learning Objectives for the First Part Examination in Intensive Care Medicine
- This will ensure that trainees, tutors, and examiners can work from a common base.
- All examination questions are based around this Syllabus.
- These learning objectives are designed to outline the minimum level of understanding required for each topic.
- The accompanying texts are recommended on the basis that the material contained within them provides sufficient information for trainees to meet the learning objectives.
- Trainees are strongly encouraged to explore the existing and evolving body of knowledge of the Basic Sciences as they apply to Intensive Care Medicine by reading widely.
- For all sections of the syllabus an understanding of normal physiology and physiology at extremes of age, obesity, pregnancy (including foetal) and disease (particularly critical illness) is expected.
- Similarly, for pharmacology, trainees are expected to understand a drug’s pharmacology in these contexts.
- An understanding of potential toxicity and relevant antidotes is also expected.
Definitions
Throughout the document specific wording has been used under the required abilities to indicate the level of knowledge and understanding expected and a glossary of these terms is provided.
Definitions
Calculate | Work out or estimate using mathematical principles. |
Classify | Divide into categories; organise, arrange. |
Compare and contrast | Examine similarities and differences. |
Define | Give the precise meaning. |
Describe | Give a detailed account of. |
Explain | Make plain. |
Interpret | Explain the meaning or significance. |
Outline | Provide a summary of the important points. |
Relate | Show a connection between. |
Understand | Appreciate the details of; comprehend. |
SAQs
H1. Renal Physiology
i. Describe the functional anatomy of the kidneys.
2019A 04 – 2017A 18
Outline the functional anatomy of the kidney (40% of marks).
Outline the regulation of renal blood flow (60% of marks).
CICMWrecks Answer – Anatomy
Kidney
- Lie in the posterior wall of the abdomen – outside peritoneum (retroperitoneal)
- Each kidney approx 150g
- Medial side of each kidney = hilum
- Renal artery and vein
- Lymphatic supply
- Nerves
- Ureter
- Kidney surrounded by tough fibrous capsule
- Kidney organized into outer cortex and inner medulla
- Medulla
- Organised into mutliple pyramids with the base at the corticomedullary junction and apices – the papilla – at the hilum draining into the ureters
- Nephrons = functional unit of the kidney (1 million / kidney)
Nephron
The functional unit of the kidney (1 million / kidney)
Parts:
- Afferent Arteriole: Brings blood to glomerulus. Regulate BP and supply blood to kidneys. Diameter bigger than Efferent Arterioles
- Efferent arteriole: Carries blood away. Regulates GFR
- Glomerulus: Provides driving force for solutes and water
- Bowman’s Capsule: Inner visceral layer, outer parietal layer. Filters bloods
- Renal Tubule: Filled with tubular fluid: aids in excretion and reabsorption
- Proximal convoluted tubule
- Loop of Henle:
- Descending Limb
- Lower thin Ascending limb
- Thick Ascending Limb
- Distal convoluted tubule
- Connecting tubule
Types by length:
- Cortical nephrons
- High in cortex, short loops of Henle.
- Juxtamedullary nephrons
- Low in cortex near medulla, long loops of Henle which penetrate medulla
- Only nephrons with vasa recta
Renal Blood Supply
- Renal blood flow ~1.25L/min (24% of cardiac output or 500ml/100g tissue/min) via renal artery
- 95% blood flow to cortex, ~5% to Medulla
- Renal artery enters through hilum it divides
- → interlobar arteries
- → arcuate arteries
- → interlobular arteries
- → afferent arterioles
- → glomerular capillaries
- → efferent arterioles
- → peritubular capillaries
- Peritubular capillaries supply blood to the renal tubules
- Juxtaglomerular capillaries have specialized vasa recta which supply the LoH and is important in renal concentration of urine
- → venous system → interlobular veins → arcuate veins → interlobar veins → renal vein
Juxtaglomerular Apparatus
Formed where the TAL of LoH and DCT passes between the afferent and efferent
arterioles at the vascular pole of the glomerulus.
Comprises of:
- Macula Densa
- Modified tubular epithelial wall of the DCT (located at the angle between
afferent and efferent arterioles) - Contains specialised cells that sense changes in the filtrate NaCl levels →
involved in intrinsic regulation of GFR and RBF by “Tubuloglomerular
feedback” and control of renin secretion
- Modified tubular epithelial wall of the DCT (located at the angle between
- Juxtaglomerular (Granular) cells
- Specialised smooth muscle cells found in the afferent arteriolar wall (esp
“media”) that possess:- Renin-containing granules → secreted to invoke RAAS
- Intrarenal-baroreceptors → involved in intrinsic autoregulation of RBF/GFR by a myogenic mechanism
- Specialised smooth muscle cells found in the afferent arteriolar wall (esp
- Extraglomerular mesangial (Lacis) cells
- Participate in a signalling pathway of the Tubuloglomerular feedback
- Possess contractile elements (actin and myosin) → contract in response to SNS stimulation → thereby ↓ glomerular surface area and GFR
JC / Sakurai 2019
CICMWrecks Answer – Physiology of Blood flow
Regulation of Renal Blood Flow
- Renal blood flow Autoregulated between 80~170mmHg
- Blood flow maintained by modulating resistance based on pressure
- Renal vascular resistance maintained by interlobular arteries, afferent arterioles and efferent arterioles, amenable to external regulation
- GFR approx 180l/day – Autoregulated by tubuloglomerular feedback – relatively constant in response to fluctuating renal blood flow
INTRINSIC Regulation (Autoregulation) of GFR and RBF:
- Renal blood flow (and consequently GFR) Autoregulated between MAP range of 75~170mmHg
- Blood flow maintained by modulating resistance of AFFERENT based on pressure
- Efferent arteriole is NOT involved in autoregulation!
- Autoregulation of GFR and RBF can be overridden by external influences (Eg. hormones and SNS neurons), even when renal perfusion pressure is between MAP 75-170 mmHg!
Mechanisms of Autoregulation:
- Myogenic autoregulation (Myogenic stretch response):
- In response to vascular wall stretch (due to increased intraluminal pressures), stretch dependent Ca influx occurs causing vasoconstricion of arterioles → increased resistance according to Poisuille-Hagen Equation → Decreased flow
- In response to shear stress (due to increased flow), Endothelial derived relaxation factors released (such as NO) → NO acts on guanylyl cyclase → increased cGMP → smooth muscle relaxation → arteriolar vasodilation
- Tubuloglomerular feedback (TGF)
- Negative feedback – Links the rate of GFR to concentration of salt in tubular fluid at macula densa
- Macula densa in wall of Ascending limb of loop of Henle Detects change in tubular flow (by the changing salt concentrations)
- As a consequence of decreased blood flow → GFR decreases → Tubular flow rate decreases → Increased uptake of [Na] in Ascending LoH → Reduced [Na+] and [Cl-] reaching the DCT and macula densa → Juxtaglomerular apparatus releases prostaglandins (PGE2) → vasodilation of afferent arteriole → increased resistance to glomerular blood flow
- With increased GFR → Increased tubular flow rate → Decreased [Na] uptake by the LoH → Increased [Na+] in macula densa → Juxtaglomerular apparatus secretes adenosine → Vasoconstriction of the afferent arteriole → Decreased blood flow
Note: Flow to Juxtamedullary nephrons is not autoregulated. High blood pressure increases juxtamedullary flow, increasing GFR and impairing renal concentration, resulting in a pressure diuresis.
Extrinsic Control of GFR and RBF:
- Hormonal regulation of blood flow
- Afferent arterioles:
- Dilation → PGE-2, PGI-2, DA, ANP, NO, kinins
- Constriction → High dose AII, NAd, ET-1, Adenosine, ADH, Thirst
- Efferent arterioles:
- Dilation → Inhibition of AT-II
- Constriction → Low dose AT-II
- Mesangial cell → Contracts due to AT-II, ADH and NAd (contraction response inhibited by ANP, DA, PGE2, PGI2)
- Note: Angiotensin II:
- At physiological (low) doses → it maintains GFR by efferent arteriolar vasoconstriction (at expense of RBF)
- With ↑ AT-II levels → causes:
- BOTH afferent and efferent arterioles constriction→ ↓ GFR
and RBF - Mesangial cell contraction in renal corpuscle → ↓ KF → ↓
GFR
- BOTH afferent and efferent arterioles constriction→ ↓ GFR
- Afferent arterioles:
- Neural regulation of renal blood flow (SNS, noradrenergic)
- All renal vescles richly innervated by sympathetic nerves
- 2 Mechanisms:
- Constricts BOTH afferent and efferent arterioles → ↓ RBF >>> GFR
- Stimulates renin secretion (via β1 receptors on JG cells) → ↑ Angiotensin II production → afferent and efferent arteriolar vasoconstriction → ↓ RBF and GFR
- Starling resistors
- Increased intra-abdominal pressure will decrease blood flow in starling resistor model
- Increased intra-capsular pressure will decrease renal blood flow
- High blood amino acid/ glucose level
- High filtered AA/ glucose load → reabsorption in PT with Na → ↓NaCl reaches distal tubules → macula densa → ↓adenosine → vasodilation → ↑RBF
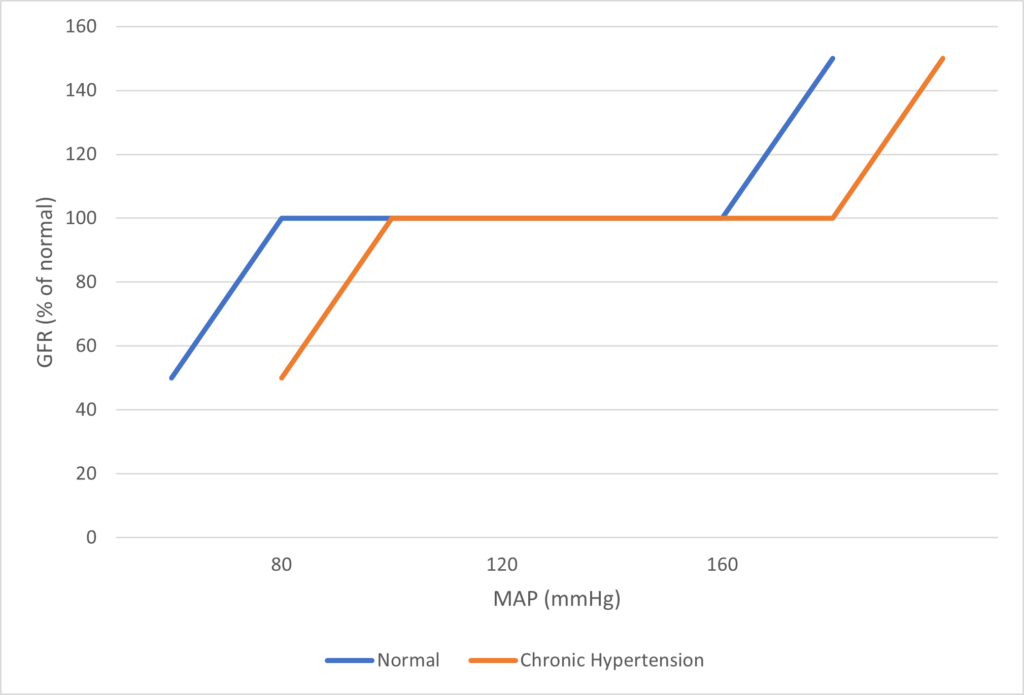
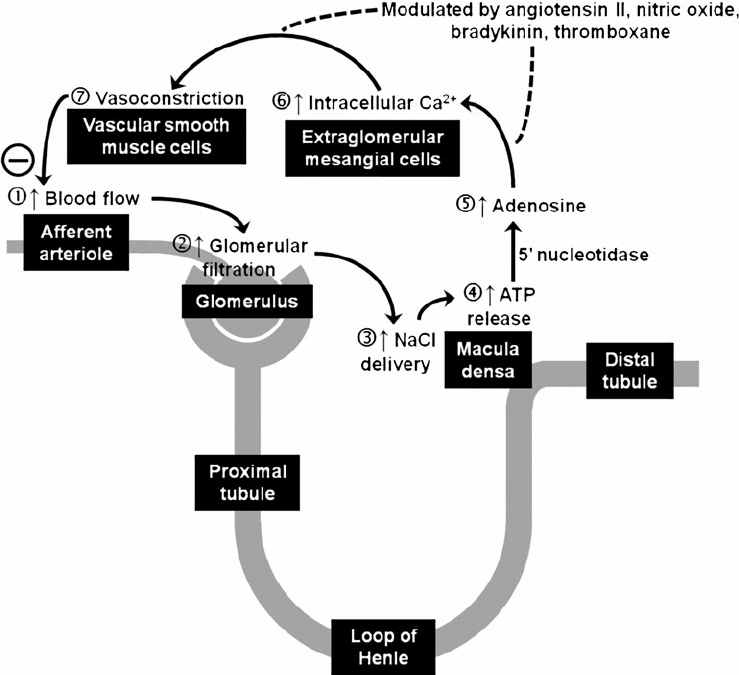
Image Source
JC / Mooney / Sakurai 2019
Examiner Comments
2019A 04: 71% of candidates passed this question.
It was expected that answers include sections on the blood supply, the nephron (including the difference between the cortical and juxta-medullary nephrons) and innervation. A number of candidates failed to quantify renal blood flow and to define autoregulation. The concept that it’s the flow that’s regulated was not described by some. Tubuloglomerular feedback was generally described correctly but a reasonable number had the blood flow increasing when an increased sodium was sensed at the macula densa.
2017A 18: 67% of candidates passed this question.
Candidates who scored well weighted their answers according to the marks allocation outlined in the question and adopted a good structure. A number of candidates confused the roles of tubuloglomerular feedback and the renin angiotensin aldosterone pathway.
ii. Describe renal blood flow and its regulation.
2019A 04 – 2017A 18
Outline the functional anatomy of the kidney (40% of marks).
Outline the regulation of renal blood flow (60% of marks).
CICMWrecks Answer – Anatomy
Kidney
- Lie in the posterior wall of the abdomen – outside peritoneum (retroperitoneal)
- Each kidney approx 150g
- Medial side of each kidney = hilum
- Renal artery and vein
- Lymphatic supply
- Nerves
- Ureter
- Kidney surrounded by tough fibrous capsule
- Kidney organized into outer cortex and inner medulla
- Medulla
- Organised into mutliple pyramids with the base at the corticomedullary junction and apices – the papilla – at the hilum draining into the ureters
- Nephrons = functional unit of the kidney (1 million / kidney)
Nephron
The functional unit of the kidney (1 million / kidney)
Parts:
- Afferent Arteriole: Brings blood to glomerulus. Regulate BP and supply blood to kidneys. Diameter bigger than Efferent Arterioles
- Efferent arteriole: Carries blood away. Regulates GFR
- Glomerulus: Provides driving force for solutes and water
- Bowman’s Capsule: Inner visceral layer, outer parietal layer. Filters bloods
- Renal Tubule: Filled with tubular fluid: aids in excretion and reabsorption
- Proximal convoluted tubule
- Loop of Henle:
- Descending Limb
- Lower thin Ascending limb
- Thick Ascending Limb
- Distal convoluted tubule
- Connecting tubule
Types by length:
- Cortical nephrons
- High in cortex, short loops of Henle.
- Juxtamedullary nephrons
- Low in cortex near medulla, long loops of Henle which penetrate medulla
- Only nephrons with vasa recta
Renal Blood Supply
- Renal blood flow ~1.25L/min (24% of cardiac output or 500ml/100g tissue/min) via renal artery
- 95% blood flow to cortex, ~5% to Medulla
- Renal artery enters through hilum it divides
- → interlobar arteries
- → arcuate arteries
- → interlobular arteries
- → afferent arterioles
- → glomerular capillaries
- → efferent arterioles
- → peritubular capillaries
- Peritubular capillaries supply blood to the renal tubules
- Juxtaglomerular capillaries have specialized vasa recta which supply the LoH and is important in renal concentration of urine
- → venous system → interlobular veins → arcuate veins → interlobar veins → renal vein
Juxtaglomerular Apparatus
Formed where the TAL of LoH and DCT passes between the afferent and efferent
arterioles at the vascular pole of the glomerulus.
Comprises of:
- Macula Densa
- Modified tubular epithelial wall of the DCT (located at the angle between
afferent and efferent arterioles) - Contains specialised cells that sense changes in the filtrate NaCl levels →
involved in intrinsic regulation of GFR and RBF by “Tubuloglomerular
feedback” and control of renin secretion
- Modified tubular epithelial wall of the DCT (located at the angle between
- Juxtaglomerular (Granular) cells
- Specialised smooth muscle cells found in the afferent arteriolar wall (esp
“media”) that possess:- Renin-containing granules → secreted to invoke RAAS
- Intrarenal-baroreceptors → involved in intrinsic autoregulation of RBF/GFR by a myogenic mechanism
- Specialised smooth muscle cells found in the afferent arteriolar wall (esp
- Extraglomerular mesangial (Lacis) cells
- Participate in a signalling pathway of the Tubuloglomerular feedback
- Possess contractile elements (actin and myosin) → contract in response to SNS stimulation → thereby ↓ glomerular surface area and GFR
JC / Sakurai 2019
CICMWrecks Answer – Physiology of Blood flow
Regulation of Renal Blood Flow
- Renal blood flow Autoregulated between 80~170mmHg
- Blood flow maintained by modulating resistance based on pressure
- Renal vascular resistance maintained by interlobular arteries, afferent arterioles and efferent arterioles, amenable to external regulation
- GFR approx 180l/day – Autoregulated by tubuloglomerular feedback – relatively constant in response to fluctuating renal blood flow
INTRINSIC Regulation (Autoregulation) of GFR and RBF:
- Renal blood flow (and consequently GFR) Autoregulated between MAP range of 75~170mmHg
- Blood flow maintained by modulating resistance of AFFERENT based on pressure
- Efferent arteriole is NOT involved in autoregulation!
- Autoregulation of GFR and RBF can be overridden by external influences (Eg. hormones and SNS neurons), even when renal perfusion pressure is between MAP 75-170 mmHg!
Mechanisms of Autoregulation:
- Myogenic autoregulation (Myogenic stretch response):
- In response to vascular wall stretch (due to increased intraluminal pressures), stretch dependent Ca influx occurs causing vasoconstricion of arterioles → increased resistance according to Poisuille-Hagen Equation → Decreased flow
- In response to shear stress (due to increased flow), Endothelial derived relaxation factors released (such as NO) → NO acts on guanylyl cyclase → increased cGMP → smooth muscle relaxation → arteriolar vasodilation
- Tubuloglomerular feedback (TGF)
- Negative feedback – Links the rate of GFR to concentration of salt in tubular fluid at macula densa
- Macula densa in wall of Ascending limb of loop of Henle Detects change in tubular flow (by the changing salt concentrations)
- As a consequence of decreased blood flow → GFR decreases → Tubular flow rate decreases → Increased uptake of [Na] in Ascending LoH → Reduced [Na+] and [Cl-] reaching the DCT and macula densa → Juxtaglomerular apparatus releases prostaglandins (PGE2) → vasodilation of afferent arteriole → increased resistance to glomerular blood flow
- With increased GFR → Increased tubular flow rate → Decreased [Na] uptake by the LoH → Increased [Na+] in macula densa → Juxtaglomerular apparatus secretes adenosine → Vasoconstriction of the afferent arteriole → Decreased blood flow
Note: Flow to Juxtamedullary nephrons is not autoregulated. High blood pressure increases juxtamedullary flow, increasing GFR and impairing renal concentration, resulting in a pressure diuresis.
Extrinsic Control of GFR and RBF:
- Hormonal regulation of blood flow
- Afferent arterioles:
- Dilation → PGE-2, PGI-2, DA, ANP, NO, kinins
- Constriction → High dose AII, NAd, ET-1, Adenosine, ADH, Thirst
- Efferent arterioles:
- Dilation → Inhibition of AT-II
- Constriction → Low dose AT-II
- Mesangial cell → Contracts due to AT-II, ADH and NAd (contraction response inhibited by ANP, DA, PGE2, PGI2)
- Note: Angiotensin II:
- At physiological (low) doses → it maintains GFR by efferent arteriolar vasoconstriction (at expense of RBF)
- With ↑ AT-II levels → causes:
- BOTH afferent and efferent arterioles constriction→ ↓ GFR
and RBF - Mesangial cell contraction in renal corpuscle → ↓ KF → ↓
GFR
- BOTH afferent and efferent arterioles constriction→ ↓ GFR
- Afferent arterioles:
- Neural regulation of renal blood flow (SNS, noradrenergic)
- All renal vescles richly innervated by sympathetic nerves
- 2 Mechanisms:
- Constricts BOTH afferent and efferent arterioles → ↓ RBF >>> GFR
- Stimulates renin secretion (via β1 receptors on JG cells) → ↑ Angiotensin II production → afferent and efferent arteriolar vasoconstriction → ↓ RBF and GFR
- Starling resistors
- Increased intra-abdominal pressure will decrease blood flow in starling resistor model
- Increased intra-capsular pressure will decrease renal blood flow
- High blood amino acid/ glucose level
- High filtered AA/ glucose load → reabsorption in PT with Na → ↓NaCl reaches distal tubules → macula densa → ↓adenosine → vasodilation → ↑RBF
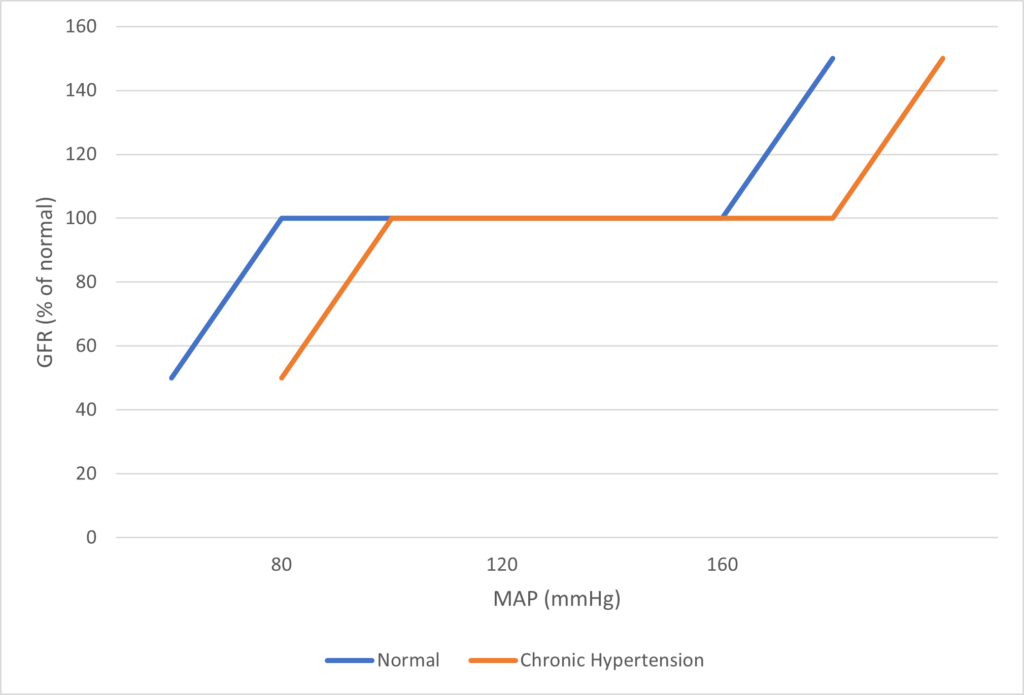
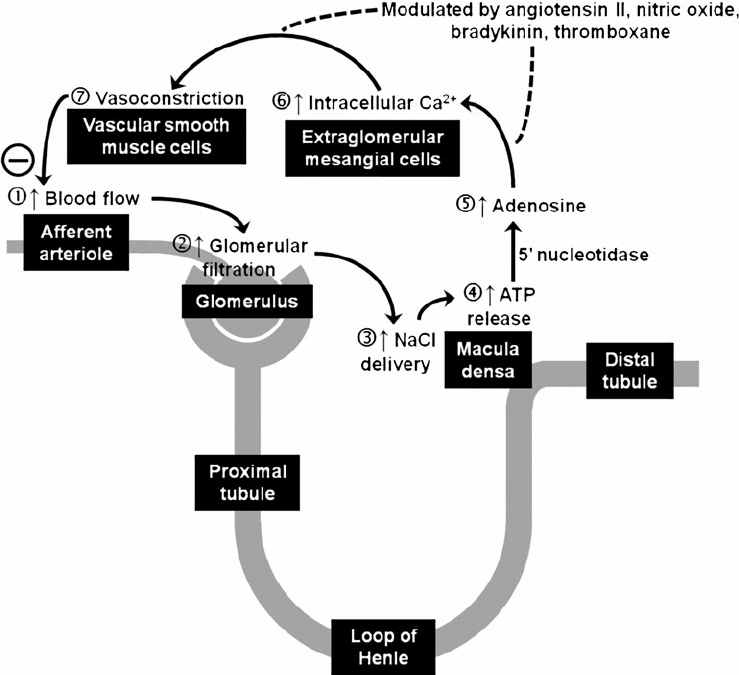
Image Source
JC / Mooney / Sakurai 2019
Examiner Comments
2019A 04: 71% of candidates passed this question.
It was expected that answers include sections on the blood supply, the nephron (including the difference between the cortical and juxta-medullary nephrons) and innervation. A number of candidates failed to quantify renal blood flow and to define autoregulation. The concept that it’s the flow that’s regulated was not described by some. Tubuloglomerular feedback was generally described correctly but a reasonable number had the blood flow increasing when an increased sodium was sensed at the macula densa.
2017A 18: 67% of candidates passed this question.
Candidates who scored well weighted their answers according to the marks allocation outlined in the question and adopted a good structure. A number of candidates confused the roles of tubuloglomerular feedback and the renin angiotensin aldosterone pathway.
2019B 02
Describe renal blood flow and its regulation (80% of marks).
Outline the impact of adrenoreceptor agonists on renal blood flow (20% of marks).
CICMWrecks Answer
Regulation of Renal Blood Flow
- Renal blood flow Autoregulated between 80~170mmHg
- Blood flow maintained by modulating resistance based on pressure
- Renal vascular resistance maintained by interlobular arteries, afferent arterioles and efferent arterioles, amenable to external regulation
- GFR approx 180l/day – Autoregulated by tubuloglomerular feedback – relatively constant in response to fluctuating renal blood flow
INTRINSIC Regulation (Autoregulation) of GFR and RBF:
- Renal blood flow (and consequently GFR) Autoregulated between MAP range of 75~170mmHg
- Blood flow maintained by modulating resistance of AFFERENT based on pressure
- Efferent arteriole is NOT involved in autoregulation!
- Autoregulation of GFR and RBF can be overridden by external influences (Eg. hormones and SNS neurons), even when renal perfusion pressure is between MAP 75-170 mmHg!
Mechanisms of Autoregulation:
- Myogenic autoregulation (Myogenic stretch response):
- In response to vascular wall stretch (due to increased intraluminal pressures), stretch dependent Ca influx occurs causing vasoconstricion of arterioles → increased resistance according to Poisuille-Hagen Equation → Decreased flow
- In response to shear stress (due to increased flow), Endothelial derived relaxation factors released (such as NO) → NO acts on guanylyl cyclase → increased cGMP → smooth muscle relaxation → arteriolar vasodilation
- Tubuloglomerular feedback (TGF)
- Negative feedback – Links the rate of GFR to concentration of salt in tubular fluid at macula densa
- Macula densa in wall of Ascending limb of loop of Henle Detects change in tubular flow (by the changing salt concentrations)
- As a consequence of decreased blood flow → GFR decreases → Tubular flow rate decreases → Increased uptake of [Na] in Ascending LoH → Reduced [Na+] and [Cl-] reaching the DCT and macula densa → Juxtaglomerular apparatus releases prostaglandins (PGE2) → vasodilation of afferent arteriole → increased resistance to glomerular blood flow
- With increased GFR → Increased tubular flow rate → Decreased [Na] uptake by the LoH → Increased [Na+] in macula densa → Juxtaglomerular apparatus secretes adenosine → Vasoconstriction of the afferent arteriole → Decreased blood flow
Note: Flow to Juxtamedullary nephrons is not autoregulated. High blood pressure increases juxtamedullary flow, increasing GFR and impairing renal concentration, resulting in a pressure diuresis.
Extrinsic Control of GFR and RBF:
- Hormonal regulation of blood flow
- Afferent arterioles:
- Dilation → PGE-2, PGI-2, DA, ANP, NO, kinins
- Constriction → High dose AII, NAd, ET-1, Adenosine, ADH, Thirst
- Efferent arterioles:
- Dilation → Inhibition of AT-II
- Constriction → Low dose AT-II
- Mesangial cell → Contracts due to AT-II, ADH and NAd (contraction response inhibited by ANP, DA, PGE2, PGI2)
- Note: Angiotensin II:
- At physiological (low) doses → it maintains GFR by efferent arteriolar vasoconstriction (at expense of RBF)
- With ↑ AT-II levels → causes:
- BOTH afferent and efferent arterioles constriction→ ↓ GFR
and RBF - Mesangial cell contraction in renal corpuscle → ↓ KF → ↓
GFR
- BOTH afferent and efferent arterioles constriction→ ↓ GFR
- Afferent arterioles:
- Neural regulation of renal blood flow (SNS, noradrenergic)
- All renal vescles richly innervated by sympathetic nerves
- 2 Mechanisms:
- Constricts BOTH afferent and efferent arterioles → ↓ RBF >>> GFR
- Stimulates renin secretion (via β1 receptors on JG cells) → ↑ Angiotensin II production → afferent and efferent arteriolar vasoconstriction → ↓ RBF and GFR
- Starling resistors
- Increased intra-abdominal pressure will decrease blood flow in starling resistor model
- Increased intra-capsular pressure will decrease renal blood flow
- High blood amino acid/ glucose level
- High filtered AA/ glucose load → reabsorption in PT with Na → ↓NaCl reaches distal tubules → macula densa → ↓adenosine → vasodilation → ↑RBF
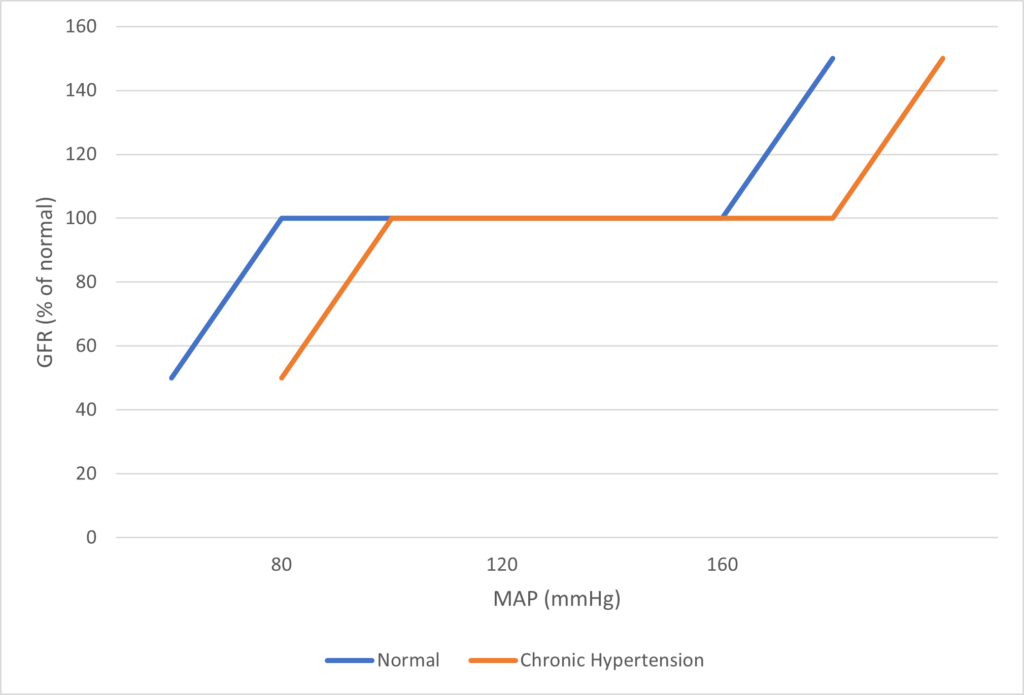
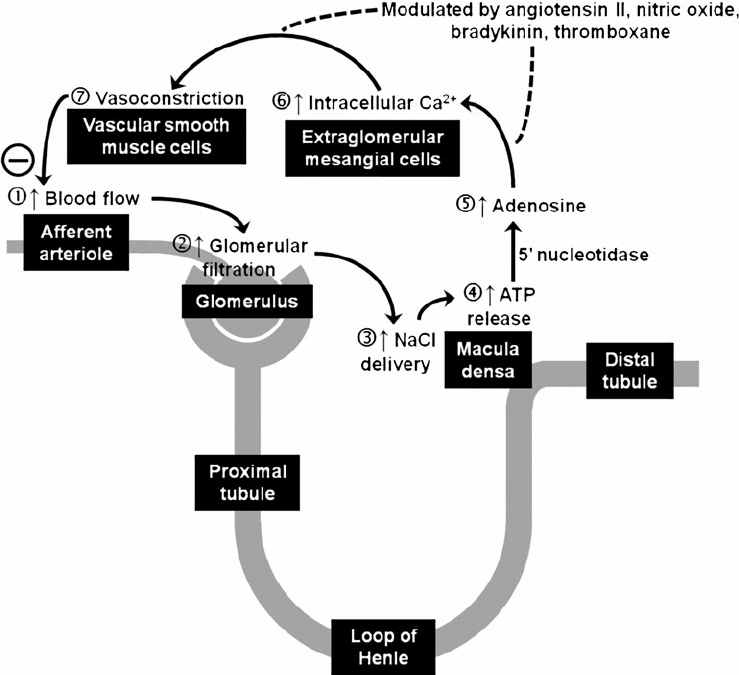
Image Source
Effect of Adrenoreceptor agonists on Renal Blood Flow
The impact of adrenoreceptor agonists is varied based on the amount of direct action in the kidney, indirect effect of altered cardiac output on Renal blood flow.
- Generally sympathomimetic agents will vasoconstrict and therefore increase renovascular resistance and result in a decrease renal blood flow.
- The relative impact on afferent vs efferent arteriolar tone may alter glomerular perfusion pressure.
Receptor stimulation effects:
- Alpha1 and Alpha2 stimulation → renal vasoconstriction → Decreased renal blood flow and GFR
- Beta1 stimulation:
- Renin release → Angiotensinogen to Angiotensin I → AT1 to AT2 by ACE → constriction of the afferent and efferent arterioles → reduces medullary blood supply
- Can cause positive inotropic effect which increases Cardiac output and can increase renal blood flow
*Note: Dopamine can cause renal vasodilatation in low doses to improve renal blood flow and increase GFR, but this action is via Dopamine DA1 receptors, not adrenergic receptors
JC / Mooney / Sakurai 2019
Examiner Comments
2019B 02: 64% of candidates passed this question.
This question was well answered by most candidates. The description of renal flow involves a brief comment of the anatomy including interlobar, arcuate, interlobular arteries, then afferent and efferent arterioles – 2 sets of capillaries and then corresponding veins and better answers made the distinction better cortical and medullary flow and went on to detail the consequence of this. Renal blood flow is autoregulated and most candidates describe well the various mechanisms around myogenic and tubuloglomerular feedback.
Additional marks were gained with by discussing renal vascular resistance and how this may be varied. The impact of adrenoreceptor agonists is varied but generally sympathomimetic agents will vasoconstrict and therefore increase renovascular resistance and result in a decrease renal blood flow. The relative impact on afferent vs efferent arteriolar tone may alter glomerular perfusion pressure.
2008B 12 : 4 (80%) candidates passed this question
This question was concerned with a very important physiological principle and was generally well answered. A good answer included a definition of autoregulation. In relation to the renal circulation, the kidneys extract only 10% of the available O2 supply and therefore the renal blood flow is high for the purpose of filtration and not metabolic demand, renal blood flow is autoregulated to remain constant against arterial blood pressures from 75 – 160 mmHg (an illustration helps explain this concept), Tubuloglomerular Feedback (including a description of the mechanism). A discussion about other mechanisms thought to play a role is important – eg intrinsic contractile response of smooth muscle to stretch (myogenic theory of autoregulation). Vasodilator substances tend to accumulate in active tissues, and these “metabolites” (decreases in O2 tension, increased CO2 tension and decreased pH) also contribute to autoregulation (metabolic theory of autoregulation). The sympathetic nerves innervate afferent and efferent arterioles. Renal autoregulation usually overrides mild to moderate degrees of sympathetic stimulation. Strong sympathetic stimulation however will constrict renal arterioles reducing flow to 10% of normal. GFR falls to a lesser extent than renal blood flow owing to a differential effect of sympathetic stimulation constricting the efferent arteriole to a greater degree than the afferent arteriole.
Syllabus: C1f 2a, d D2i
Reference Text: Guyton Chp 26
2007B 18: 3 candidates (43%) passed this question.
To obtain a pass candidates needed to briefly define autoregulation and state the range of MAP over which this occurs, and why it occurs, then provide a more detailed discussion about the mechanisms thought to be responsible for this.
The main site of autoregulation in the kidney is the afferent arteriole. There are two main factors that affect vascular tone in the afferent arteriloe, these are stretch-activated constriction of vessels (myogenic mechanism) and tubuloglomerular feedback (TGF).
Both of the above mechanisms are important to maintenance of near-constant blood flow. Stretch results in membrane depolarisation, increased intra-cellular concentrations of Ca and ultimately, vasoconstriction.
In tubulo-glomerular feedback, complex signals pass from the macula densa to the afferent arteriole, regulating its tone. The fundamental theme of TGF is that increased delivery of fluid and/or NaCl to the distal tubule causes vasoconstriction, thus limiting the flow (negative feedback).
The major weakness in answes was again the failure to include sufficient information to acheive a pass mark.
2008B 12
Describe the concept of autoregulation as it relates to the renal circulation.
2007B 18
Describe the autoregulation of renal blood flow.
CICMWrecks Answer
Regulation of Renal Blood Flow
- Renal blood flow Autoregulated between 80~170mmHg
- Blood flow maintained by modulating resistance based on pressure
- Renal vascular resistance maintained by interlobular arteries, afferent arterioles and efferent arterioles, amenable to external regulation
- GFR approx 180l/day – Autoregulated by tubuloglomerular feedback – relatively constant in response to fluctuating renal blood flow
INTRINSIC Regulation (Autoregulation) of GFR and RBF:
- Renal blood flow (and consequently GFR) Autoregulated between MAP range of 75~170mmHg
- Blood flow maintained by modulating resistance of AFFERENT based on pressure
- Efferent arteriole is NOT involved in autoregulation!
- Autoregulation of GFR and RBF can be overridden by external influences (Eg. hormones and SNS neurons), even when renal perfusion pressure is between MAP 75-170 mmHg!
Mechanisms of Autoregulation:
- Myogenic autoregulation (Myogenic stretch response):
- In response to vascular wall stretch (due to increased intraluminal pressures), stretch dependent Ca influx occurs causing vasoconstricion of arterioles → increased resistance according to Poisuille-Hagen Equation → Decreased flow
- In response to shear stress (due to increased flow), Endothelial derived relaxation factors released (such as NO) → NO acts on guanylyl cyclase → increased cGMP → smooth muscle relaxation → arteriolar vasodilation
- Tubuloglomerular feedback (TGF)
- Negative feedback – Links the rate of GFR to concentration of salt in tubular fluid at macula densa
- Macula densa in wall of Ascending limb of loop of Henle Detects change in tubular flow (by the changing salt concentrations)
- As a consequence of decreased blood flow → GFR decreases → Tubular flow rate decreases → Increased uptake of [Na] in Ascending LoH → Reduced [Na+] and [Cl-] reaching the DCT and macula densa → Juxtaglomerular apparatus releases prostaglandins (PGE2) → vasodilation of afferent arteriole → increased resistance to glomerular blood flow
- With increased GFR → Increased tubular flow rate → Decreased [Na] uptake by the LoH → Increased [Na+] in macula densa → Juxtaglomerular apparatus secretes adenosine → Vasoconstriction of the afferent arteriole → Decreased blood flow
Note: Flow to Juxtamedullary nephrons is not autoregulated. High blood pressure increases juxtamedullary flow, increasing GFR and impairing renal concentration, resulting in a pressure diuresis.
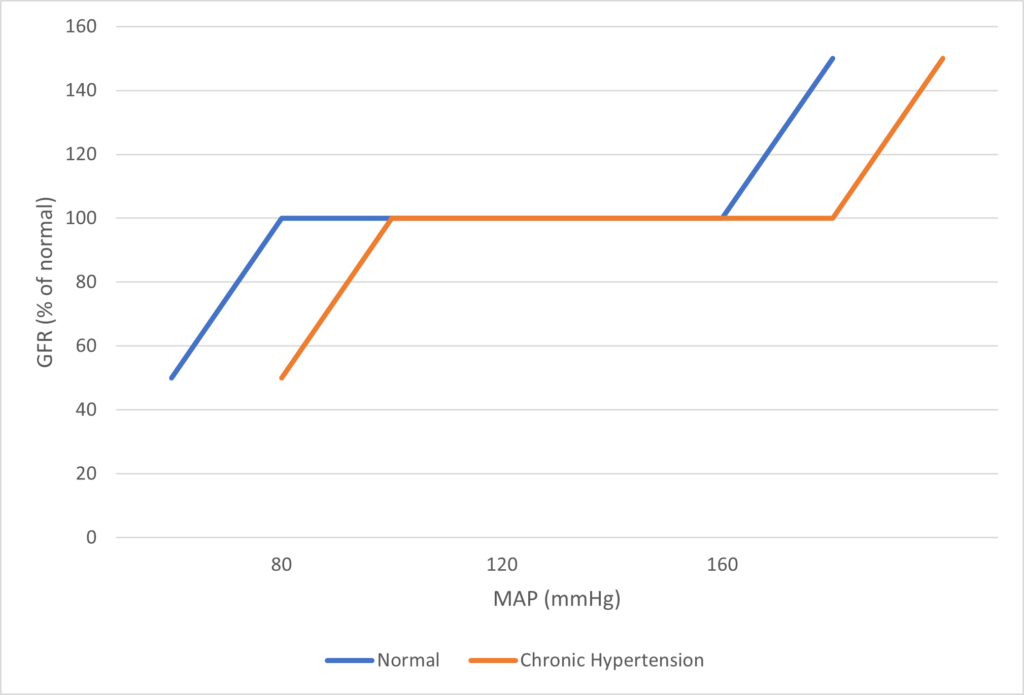
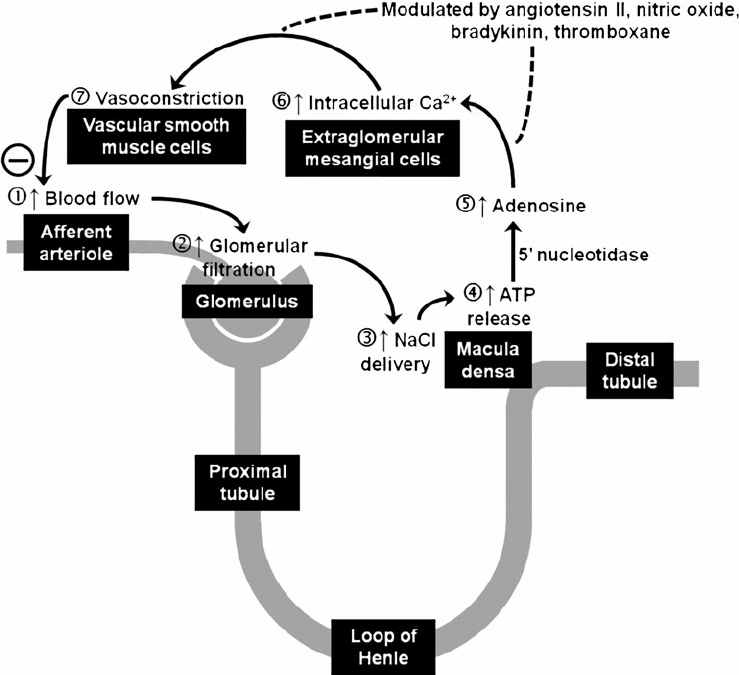
Image Source
JC / Mooney / Sakurai 2019
Examiner Comments
2008B 12 : 4 (80%) candidates passed this question
This question was concerned with a very important physiological principle and was generally well answered. A good answer included a definition of autoregulation. In relation to the renal circulation, the kidneys extract only 10% of the available O2 supply and therefore the renal blood flow is high for the purpose of filtration and not metabolic demand, renal blood flow is autoregulated to remain constant against arterial blood pressures from 75 – 160 mmHg (an illustration helps explain this concept), Tubuloglomerular Feedback (including a description of the mechanism). A discussion about other mechanisms thought to play a role is important – eg intrinsic contractile response of smooth muscle to stretch (myogenic theory of autoregulation). Vasodilator substances tend to accumulate in active tissues, and these “metabolites” (decreases in O2 tension, increased CO2 tension and decreased pH) also contribute to autoregulation (metabolic theory of autoregulation). The sympathetic nerves innervate afferent and efferent arterioles. Renal autoregulation usually overrides mild to moderate degrees of sympathetic stimulation. Strong sympathetic stimulation however will constrict renal arterioles reducing flow to 10% of normal. GFR falls to a lesser extent than renal blood flow owing to a differential effect of sympathetic stimulation constricting the efferent arteriole to a greater degree than the afferent arteriole.
Syllabus: C1f 2a, d D2i
Reference Text: Guyton Chp 26
2007B 18: 3 candidates (43%) passed this question.
To obtain a pass candidates needed to briefly define autoregulation and state the range of MAP over which this occurs, and why it occurs, then provide a more detailed discussion about the mechanisms thought to be responsible for this.
The main site of autoregulation in the kidney is the afferent arteriole. There are two main factors that affect vascular tone in the afferent arteriloe, these are stretch-activated constriction of vessels (myogenic mechanism) and tubuloglomerular feedback (TGF).
Both of the above mechanisms are important to maintenance of near-constant blood flow. Stretch results in membrane depolarisation, increased intra-cellular concentrations of Ca and ultimately, vasoconstriction.
In tubulo-glomerular feedback, complex signals pass from the macula densa to the afferent arteriole, regulating its tone. The fundamental theme of TGF is that increased delivery of fluid and/or NaCl to the distal tubule causes vasoconstriction, thus limiting the flow (negative feedback).
The major weakness in answes was again the failure to include sufficient information to acheive a pass mark.
2011B 21
Outline the functional anatomy, and the physiological factors, that determine oxygen delivery to the renal medulla.
CICMWrecks Answer
Anatomy
- Lie in the posterior wall of the abdomen – outside peritoneum (retroperitoneal)
- Each kidney approx 150g
- Medial side of each kidney = hilum
- Renal artery and vein
- Lymphatic supply
- Nerves
- Ureter
- Kidney surrounded by tough fibrous capsule
- Kidney organized into outer cortex and inner medulla
- Nephrons = functional unit of the kidney (1 million / kidney)
- Medulla
- Organised into mutliple pyramids with the base at the corticomedullary junction and apices – the papilla – at the hilum draining into the ureters
- Renal blood flow
- ~1.25L/min (24% of cardiac output or 500ml/100g tissue/min) via renal artery
- 95% blood flow to cortex, ~5% to Medulla
- Renal artery enters through hilum it divides
- → interlobar arteries
- → arcuate arteries
- → interlobular arteries
- → afferent arterioles
- → glomerular capillaries
- → efferent arterioles
- → peritubular capillaries
- Peritubular capillaries supply blood to the renal tubules
- Juxtaglomerular capillaries have specialized vasa recta which supply the LoH and is important in renal concentration of urine
- → venous system → interlobular veins → arcuate veins → interlobar veins → renal vein
Renal Medulla
- Organised into mutliple pyramids with the base at the corticomedullary junction and apices – the papilla – at the hilum draining into the ureters
- Medullary blood flow: 1~2% of total renal blood flow
- 0.6ml/g/min in inner medulla
- 2.5ml/g/min in outer medullar
- 5ml/g/min in cortex
- 20~30% nephrons are juxtaglomerular with specialized vasa recta
- Blood from post-glomerulus, therefore decreased plasma (filtered) proportion and increased viscosity
- Decreased flow
- Factors that increase filtration fraction decrease medullary flow (e.g. angiotensin II)
- All extrinsic factors which affect afferent arterioles (humoral, neural) affect medullary blood flow similar to RBF
- Oxygen supply determined by state of oxyHb dissociation curve
- Right shift favours oxygen supply
- Renal medullary pO2 = 15mmHg (compared with 50mmHg in cortex)
- Increased O2 offloading
- Renal oxygen consumption
- Increases with sodium resorption (happens mostly in medulla)
Physiology
Oxygen Delivery
CaO2 is determined by:
- CaO2 – Arterial oxygen content
- Hb – Haemoglobin
- SaO2 – Arterial Oxygen saturation
- paO2 – Arterial partial pressure of oxygen
Renal Blood Flow
- Determined by:
- Regulation:
- Renal vascular resistance maintained by interlobular arteries, afferent arterioles and efferent arterioles
- Autoregulated between 80~170mmHg
Neural | Sympathetic | All renal vesicles richly innervated by sympathetic nerves |
Minimal influence on GFR except in severe acute disturbances (severe haemorrhage) | ||
Decrease blood flow due to vasoconstriction | ||
Hormonal | Adrenaline and noradrenaline | Vasoconstrict → decrease blood flow |
Endothelin | Vasoconstrict → decrease blood flow | |
Angiotensin II | Constricts efferent arterioles → decrease blood flow | |
Nitric oxide | Vasodilate → increase blood flow | |
Prostaglandins | Vasodilate → increase blood flow |
- Starling resistors
- Increased intra-abdominal pressure will decrease blood flow in starling resistor model
- Increased intra-capsular pressure will decrease renal blood flow
Sakurai 2016
Examiner Comments
2011B 21: 1 (4%) of candidates passed this question.
A good answer required mentioning factors that affect systemic oxygen capacity and delivery (eg Hb, cardiac output, PaO2, etc), Hb-HBO2 dissociation and a description of the anatomy and regulation of renal medullary blood flow. 20 – 30% of nephrons have glomeruli deep in the cortex and long Loop of Henle that go deep into medulla. Here blood supply differs – long efferent arterioles from glomeruli into outer medulla and inner cortex that then divide into vasa recta deep in medulla. These vessels carry post glomerular blood so have less serum, mostly plasma, ie more viscous and concentrated. Factors that influence medullary blood flow include: Sympathetic stimulation – decrease (via efferent arteriolar constriction), Angiotensin II (via tubuloglomerular feedback) – decrease (via efferent arteriolar constriction), Endothelin – decrease (via efferent arteriolar constriction), Prostaglandins – increase, Bradykinin – increase, high protein meal – increase, high glucose levels – increase. Candidates performed poorly due to a lack of knowledge of the topic and/or failure to logically present their answer. Common mistakes were to give no value for renal blood flow, to discuss the function of the medulla which was not required and to not describe the factors that influence medullary blood flow.
Syllabus: B1h 2a,b; C1f 2d
Recommended sources: Guyton Medical Physiology Chp 26; Vander Renal Physiology Chps1, 5
2015B 03 – 2012A 11
Compare and contrast renal and hepatic blood flow, and their regulation.
CICMWrecks Answer
Blood flow
Renal | Hepatic | |
---|---|---|
Blood Flow | 1.25l/min Highest blood flow per tissue mass | 1.5l/min Highest blood flow per organ |
Afferent | Renal artery (from aorta) | – Hepatic artery (30%) from coeliac trunk – Portal vein (70%) from SMV, IMV and splenic veins – Hepatic artery supplies 50% of O¬2 |
Course | Portal system → Afferent arteriole → glomerulus → efferent arteriole → peritubular capillary (inc. vasa recta) → renal vein | Via portal triad → hepatic sinusoids → central vein → hepatic vein |
Efferent | Renal vein to IVC | Hepatic vein to IVC |
Liver has blood reservoir function – 400ml |
Regulation
Renal | Hepatic | |
---|---|---|
Hepatic arterial tone above sBP >80mmHg | ||
Local | Myogenic mechanism – Stretch of afferent arterioles causes contraction decreasing blood flow Tubuloglomerular feedback – Na and Cl are sensed by the macula densa causing adenosine release – Adenosine constricts the afferent arteriole reducing renal blood flow | Myogenic autoregulation – Stretch of arterioles causes contraction decreasing blood flow Semi-reciprocal relationship between portal venous and hepatic arterial blood flow – Decreased blood flow via portal vein increases hepatic arterial blood flow |
External – Hormonal | RAAS – Juxtaglomerular apparatus secretes renin in response to decreased tubular [Na] and [Cl], as well as to β stimulation – Angiotensin II constricts both afferent and efferent arterioles reducing renal blood flow | Adrenaline – Constricts portal vein and dilates hepatic artery – increasing hepatic artery contribution to blood flow Angiotensin II – Vasoconstricts both portal vein and hepatic artery decreasing hepatic artery blood flow Vasoactive intestinal peptide and secretin – Increase hepatic artery blood flow Glucagon – Increased hepatic blood flow |
External -Neural | – Sympathetic stimulation constricts the renal afferent and efferent arterioles reducing renal blood flow | – Sympathetic (α adrenergic) stimulation by noradrenaline causes portal venous constriction and decreased hepatic blood flow |
External -Starling resistors | – Increased intra-abdominal pressure will decrease blood flow in starling resistor model – Increased intra-capsular pressure will decrease renal blood flow | Respiration – Inspiration decreases hepatic venous blood flow |
External – dietary | High blood amino acid/ glucose level – vasodilation and increased renal blood flow | Post-prandial – Increased splanchnic blood flow – increased portal venous blood flow |
Examiner Comments
2015B 03: 54% of candidates passed this question.
It was expected candidates would describe the salient features of the anatomy, distribution and content of blood flow and influences on each circulation. Answers with a clear organisation and context for the normal influences of blood flow on the functioning of each organ system scored highly. Anatomy was often sufficiently covered, but candidates often did not take advantage of that by linking the anatomical features to the functional concepts. Figures should be clearly and accurately labelled to score well. Many answers failed to demonstrate a depth of understanding of key concepts. For example tubuloglomerular feedback, relationship between hepatic arterial and portal venous flows and autoregulation within both those systems was often poorly described.
2012A 11: 7 (70%) of candidates passed.
Another fundamental physiology topic that required candidates to understand, and synthesize knowledge from multiple areas. Generally well done with some very good answers. A tabular format worked well. Candidates were expected to mention values for renal and hepatic flow (total flow, % of cardiac output and oxygen consumption), basic anatomical comparisons, distribution (e.g. renal cortex 95% , renal medulla 5%,), two capillary beds (glomerular and peritubular) for renal, and hepatic triad and sinusoids for the liver, function (filtration for renal blood flow and metabolic activity for hepatic) and regulatory mechanisms for each (e.g. myogenic, autonomic, metabolic and humoral for both and tubuloglomerular feedback for renal).
iii. Describe glomerular filtration and tubular function.
2016A 04
Discuss the factors that influence filtration across the glomerular basement membrane.
2014A 23
What is the Glomerular Filtration Rate (GFR)? Discuss the physiological factors that can influence it.
2009A 13
Outline the factors that determine the composition and volume of glomerular filtrate in a normal person.
CICMWrecks Answer
GFR
GFR:
- “The amount of glomerular ultrafiltrate formed divided by the time of filtration”
- Normal value is approx 125 mL/min or 180 L/day
- Renal blood flow 1.25l/min in 70kg male → Filtration fraction 0.2
Functional anatomy:
3 Distinct Layers:
- glomerular capillary endothelium
- highly specialised endothelium with fenestrations to ↓ filter thickness
- prevents cellular components of blood from coming into contact with BM
- glomerular BM
- made of CT; -vely charged
- acts as filter
- bowmans epithelial cells (podocytes)
- epithelial cells with foot processes → large SA
- negatively charged
- maintain BM + phagocytic functions
Direct GFR Determinants
The GFR (Net flux across the membrane) is the balance of hydrostatic pressure and oncotic pressure, as defined by the Classic Starling Equation.
where
Kf = Filtration coefficient
P = hydrostatic pressure
π = oncotic pressure
σ = Staverman’s reflection coefficient ie. Permeability of membrane to protein
- Normally NFP = 17mmHg
- Tubular oncotic pressure = zero throughout
- GC oncotic pressure varies from 21 to 33 mmHg as filtrate is removed.
- Thus less GFR produced at distal end of tubule.
Afferent end of Glomerular Capillary (mmHg) | Efferent end of Glomerular Capillary (mmHg) | |
---|---|---|
PGC | 60 | 58 |
PT | 15 | 15 |
πGC | 21 | 33 |
NFP | 24 | 10 |
Kf | Filtration coefficient | = LpS = Hydraulic conductivity x Surface Area Glomerular surface area = 0.8m2 • Altered by Mesangial cell contraction (see circulating factors e.g. Angiotensin II → ↓SA → ↓GFR) Patency of the normal capillary wall structure (ie in tubular dysfunction Kf ↑’s ↑GFR) |
PGC | hydrostatic pressure in capillary | relates to • RBF which is autoregulated for MAP 70-170mmHg • relative afferent/efferent arteriolar tone Affected by • Catecholamines • Local autoregulation -> Myogenic -> Tubuloglomerular feedback -> Hormones (see below) |
PT | hydrostatic pressure in tubule | relates to • obstruction to urinary flow (usually pathological) • ↑PT → ↓ GFR (ie post renal obstructiion causing renal failure) |
πGC | oncotic pressure in capillary | relates to • plasma protein concentration (incr in dehydration, decreased in heart failure) • ↑Systemic plasma oncotic pressure → ↑πGC → ↓GFR • ↓Renal plasma flow → ↑πGC → ↓GFR |
πT | oncotic pressure in tubule | • usually zero, but can increase in renal failure/proteinuria • ↑’d πT → ↑GFR |
σ | Staverman’s reflection coefficient | • Permeability of membrane to protein usually 1 (no protein leak) • can decrease with nephritis/proteinuria |
Factors influencing GFR
Circulating Factors
- Prostaglandins
- PGI2 and PGE2 → vasodilates → ↑ renal blood flow → ↑ GFR
- ↓ PGE2, PGI2 and NO levels → inhibits afferent arteriolar vasodilation → ↓ GFR
- Long term → ↓ renin release → ↓ ATII-induced efferent arteriolar vasoconstriction → ↓ GFR
- Noradrenaline / Adrenaline
- constrict renal afferent and efferent arterioles → ↓ renal blood flow and reducing filtration → ↓GFR
- Constrict mesangial cells to → ↓ GFR.
- Mesangial cell tone:
- Angiotensin 2, Endothelin, vasopressin → ↓ glomerular surface area and GFR
- ANP, PGE2, Dopamine and cAMP → all increase GFR
Solute factors
- Size
- < 7 kDa (Eg. glucose, ions, urea, H2O) filtered freely,
- > 70 kDa (Eg. albumin) are not filtered;
- neutral particles < 4 mm diameter filtered freely, > 8 mm are excluded
- Charge
- Negatively charge (Anionic) particles repulsed
- Cationic substances filtered more readily
- Protein binding
- Albumin excluded
Disease Factors
- Filtration decreases
- Shock → decreased glomerular pressure
- Obstruction → increased bowman’s capsule hydrostatic pressure
- Hypoproteinaemia → hepatic failure, nephrotic syndrome
JC / Gladwin / Sakurai / Kerr 2020
Examiner Comments
2016A 04: 38% of candidates passed this question.
It was expected this answer would involve discussion about membrane structure, the unique blood vessel structure (afferent and efferent arterioles allowing a high net pressure to be maintained) and Starlings forces all influencing ultrafiltation. Better answers included comment on mesangial cells contraction to decrease surface area ( caused by angiotensin 2). Details regarding molecular weight cut offs (> 7000 Da are not filtered freely) gained additional credit
2014A 23: 40% of candidates passed this question.
Generally this question was well answered. Almost all answers gave the correct value for GFR and the correct formula for Starling’s forces across the glomerular membrane. Better answers discussed the physiological factors affecting each force (hydrostatic and osmotic) across the membrane in a stepwise logical manner. Some answers discussed the control of renal blood flow; this was not expected and therefore was not rewarded
2009A 13: Pass rate: 30%
The volume and composition of the glomerular filtrate are best explained by referring to the equation: Glomerular Filtration = Kf x net filtration pressure. Then describing the factors that affect each part of the equation e.g. hydrostatic pressure, oncotic pressure, factors that influence the filtration coefficient e.g. surface area
Glomerular filtrate is an ultrafiltrate of plasma and factors that affect the passage of proteins and other molecules should be discussed Extra marks were given for an explanation of filtration fraction and short facts about GFR in paediatric patients and the elderly.
No marks were given for any discussion of drugs.
Syllabus D2b
Reference; Power and Kam 1st edition p 197-199
2016B 03
Describe the factors that determine glomerular filtration rate (GFR) in the kidney (70% of marks). Outline methods by which GFR can be measured (30% of marks).
CICMWrecks Answer – GFR, Determinants, Factors
GFR
GFR:
- “The amount of glomerular ultrafiltrate formed divided by the time of filtration”
- Normal value is approx 125 mL/min or 180 L/day
- Renal blood flow 1.25l/min in 70kg male → Filtration fraction 0.2
Functional anatomy:
3 Distinct Layers:
- glomerular capillary endothelium
- highly specialised endothelium with fenestrations to ↓ filter thickness
- prevents cellular components of blood from coming into contact with BM
- glomerular BM
- made of CT; -vely charged
- acts as filter
- bowmans epithelial cells (podocytes)
- epithelial cells with foot processes → large SA
- negatively charged
- maintain BM + phagocytic functions
Direct GFR Determinants
The GFR (Net flux across the membrane) is the balance of hydrostatic pressure and oncotic pressure, as defined by the Classic Starling Equation.
where
Kf = Filtration coefficient
P = hydrostatic pressure
π = oncotic pressure
σ = Staverman’s reflection coefficient ie. Permeability of membrane to protein
- Normally NFP = 17mmHg
- Tubular oncotic pressure = zero throughout
- GC oncotic pressure varies from 21 to 33 mmHg as filtrate is removed.
- Thus less GFR produced at distal end of tubule.
Afferent end of Glomerular Capillary (mmHg) | Efferent end of Glomerular Capillary (mmHg) | |
---|---|---|
PGC | 60 | 58 |
PT | 15 | 15 |
πGC | 21 | 33 |
NFP | 24 | 10 |
Kf | Filtration coefficient | = LpS = Hydraulic conductivity x Surface Area Glomerular surface area = 0.8m2 • Altered by Mesangial cell contraction (see circulating factors e.g. Angiotensin II → ↓SA → ↓GFR) Patency of the normal capillary wall structure (ie in tubular dysfunction Kf ↑’s ↑GFR) |
PGC | hydrostatic pressure in capillary | relates to • RBF which is autoregulated for MAP 70-170mmHg • relative afferent/efferent arteriolar tone Affected by • Catecholamines • Local autoregulation -> Myogenic -> Tubuloglomerular feedback -> Hormones (see below) |
PT | hydrostatic pressure in tubule | relates to • obstruction to urinary flow (usually pathological) • ↑PT → ↓ GFR (ie post renal obstructiion causing renal failure) |
πGC | oncotic pressure in capillary | relates to • plasma protein concentration (incr in dehydration, decreased in heart failure) • ↑Systemic plasma oncotic pressure → ↑πGC → ↓GFR • ↓Renal plasma flow → ↑πGC → ↓GFR |
πT | oncotic pressure in tubule | • usually zero, but can increase in renal failure/proteinuria • ↑’d πT → ↑GFR |
σ | Staverman’s reflection coefficient | • Permeability of membrane to protein usually 1 (no protein leak) • can decrease with nephritis/proteinuria |
Factors influencing GFR
Circulating Factors
- Prostaglandins
- PGI2 and PGE2 → vasodilates → ↑ renal blood flow → ↑ GFR
- ↓ PGE2, PGI2 and NO levels → inhibits afferent arteriolar vasodilation → ↓ GFR
- Long term → ↓ renin release → ↓ ATII-induced efferent arteriolar vasoconstriction → ↓ GFR
- Noradrenaline / Adrenaline
- constrict renal afferent and efferent arterioles → ↓ renal blood flow and reducing filtration → ↓GFR
- Constrict mesangial cells to → ↓ GFR.
- Mesangial cell tone:
- Angiotensin 2, Endothelin, vasopressin → ↓ glomerular surface area and GFR
- ANP, PGE2, Dopamine and cAMP → all increase GFR
Solute factors
- Size
- < 7 kDa (Eg. glucose, ions, urea, H2O) filtered freely,
- > 70 kDa (Eg. albumin) are not filtered;
- neutral particles < 4 mm diameter filtered freely, > 8 mm are excluded
- Charge
- Negatively charge (Anionic) particles repulsed
- Cationic substances filtered more readily
- Protein binding
- Albumin excluded
Disease Factors
- Filtration decreases
- Shock → decreased glomerular pressure
- Obstruction → increased bowman’s capsule hydrostatic pressure
- Hypoproteinaemia → hepatic failure, nephrotic syndrome
JC / Gladwin / Sakurai / Kerr 2020
CICMWrecks Answer – GFR Measurement
GFR Measurement
Clearance:
- Renal clearance = vol of plasma completely cleared of a given substance by the kidneys per unit time (ml/min)
- Involves: glomerular filtration, secretion, reabsorption, and rarely tubular metabolism
- Renal clearance = V x [U]/[P]
- V = volume of urine or urine flow rate in ml/min
- [U] = urinary concentration of substance in mg/ml
- [P] = plasma concentration of substance in mg/ml
Estimating GFR:
- GFR = renal clearance of a substance if it is:
- Freely filtered at glomerulus
- Not secreted
- Not reabsorbed
- Not synthesized
- Not metabolised
- The amount excreted in the urine = amount filtered
- i.e. [plasma] x GFR = [urine] x urine vol
- Rearrange: GFR = urine vol x [urine] / [plasma]
Substances used to estimate GFR:
- Criteria
- easily measured in urine + plasma
- non-toxic
- easily administered
- Inulin
- Protein; polymer of fructose
- MW 5800 Da
- freely filtered
- Not reabsorbed, secreted, metabolised, or synthesized by the kidney
- Therefore clearance of inulin = GFR
- Requires continuous infusion for several hours to achieve steady state
- Creatinine
- used to approximate GFR as is more practical
- Released at a steady state from skeletal muscle cells (phosphocreatine)
- Freely filtered + not reabsorbed
- *Small amount secreted → overestimates GFR by small amount
- Cockcroft Gault formula used
JC / Gladwin / Sakurai / Kerr 2020
Examiner Comments
2016B 03: 57% of candidates passed this question.
Good answers included a description of Starling forces acting at the glomerular basement membrane. A description of the local and systemic factors influencing each component was expected.
It was expected candidates would discuss autoregulation of GFR & RBF, tubuloglomerular feedback, and integrated responses the body uses to keep GFR steady. Confusion about the nature of induced effects on afferent or efferent arteriolar dilation and constriction limited marks for some candidates. Many failed to mention the effects of mesangial surface area, Bowmans space pressure or serum protein content.
Candidates were expected to outline the methods of GFR estimation. Better responses described the rationale behind the use and limitations. Creatinine clearance, inulin and nuclear medicine techniques all scored marks. Some candidates made no attempt at this section and missed the opportunity to score marks. Estimates of CrCl/GFR [eGFR by formulae such as Cockcroft Gault, and serum Cr] are not measurement of GFR.
2017B 03
Describe the factors that determine the filtered load of a substance at the renal glomerulus
CICMWrecks Answer
Filtered Load
- It is the amount of substance that is filtered per unit time.
- For freely filtered substances, The filtered load is equal to:
- E.g: Na: Filtered load = GFR x Px = 125ml/min x 0.14mEq/ml(or 140mEq/L) = 17.5 mEq/min.
- The filtered load is what is presented to the rest of the nephron to handle.
- For substances that are freely filtered:
- The filtered load varies only with plasma concentration and GFR.
- A rise in GFR, at constant plasma concentration increases the filtered load, as does a rise in plasma concentration at constant GFR.
- For substances which are not freely filtered, filtration also depends on
- protein binding
- molecular size
- charge
GFR
Direct Determinants
The GFR (Net flux across the membrane) is the balance of hydrostatic pressure and oncotic pressure, as defined by the Classic Starling Equation.
where
Kf = Filtration coefficient
P = hydrostatic pressure
π = oncotic pressure
σ = Staverman’s reflection coefficient ie. Permeability of membrane to protein
Kf | Filtration coefficient | = LpS = Hydraulic conductivity x Surface Area Glomerular surface area = 0.8m2 • Altered by Mesangial cell contraction (see circulating factors e.g. Angiotensin II → ↓SA → ↓GFR) Patency of the normal capillary wall structure (ie in tubular dysfunction Kf ↑’s ↑GFR) |
PGC | hydrostatic pressure in capillary | relates to • RBF which is autoregulated for MAP 70-170mmHg • relative afferent/efferent arteriolar tone Affected by • Catecholamines • Local autoregulation -> Myogenic -> Tubuloglomerular feedback -> Hormones (see below) |
PT | hydrostatic pressure in tubule | relates to • obstruction to urinary flow (usually pathological) • ↑PT → ↓ GFR (ie post renal obstructiion causing renal failure) |
πGC | oncotic pressure in capillary | relates to • plasma protein concentration (incr in dehydration, decreased in heart failure) • ↑Systemic plasma oncotic pressure → ↑πGC → ↓GFR • ↓Renal plasma flow → ↑πGC → ↓GFR |
πT | oncotic pressure in tubule | • usually zero, but can increase in renal failure/proteinuria • ↑’d πT → ↑GFR |
σ | Staverman’s reflection coefficient | • Permeability of membrane to protein usually 1 (no protein leak) • can decrease with nephritis/proteinuria |
Circulating Factors
- Prostaglandins
- PGI2 and PGE2 → vasodilates → ↑ renal blood flow → ↑ GFR
- ↓ PGE2, PGI2 and NO levels → inhibits afferent arteriolar vasodilation → ↓ GFR
- Long term → ↓ renin release → ↓ ATII-induced efferent arteriolar vasoconstriction → ↓ GFR
- Noradrenaline / Adrenaline
- constrict renal afferent and efferent arterioles → ↓ renal blood flow and reducing filtration → ↓GFR
- Constrict mesangial cells to → ↓ GFR.
- Mesangial cell tone:
- Angiotensin 2, Endothelin, vasopressin → ↓ glomerular surface area and GFR
- ANP, PGE2, Dopamine and cAMP → all increase GFR
Disease Factors
- Filtration decreases
- Shock → decreased glomerular pressure
- Obstruction → increased bowman’s capsule hydrostatic pressure
- Hypoproteinaemia → hepatic failure, nephrotic syndrome
Plasma Concentration
Plasma concentration is a complex interplay of factors:
- Extrinsic substances like drugs: dosage, time and route of administration, the bioavailability of the drug
- Absorption / Production of substance
- Distribution
- Metabolism
- Excretion
- Inter-relationships with other substances or drugs
- Concurrent drug administration may profoundly affect plasma drug concentrations
Additional Factors for Substances not Freely filtered
- Molecular Size
- < 7 kDa (Eg. glucose, ions, urea, H2O) filtered freely,
- > 70 kDa (Eg. albumin) are not filtered;
- neutral particles < 4 mm diameter filtered freely, > 8 mm are excluded
- Molecular Charge
- Negatively charge (Anionic) particles repulsed
- Cationic substances filtered more readily
- Protein binding
- Albumin excluded
JC / Gladwin / Sakurai / Kerr 2020
Examiner Comments
2017B 03: 67% of candidates passed this question.
A good place to start was with the correct equation for a filtered load and a description of the components. Better answers described the components and how they differ and change over the glomerulus. Many candidates usefully based answers around the Starling forces.
A summary of factors including the role of plasma concentration, protein binding, molecular size and charge was required to pass. Many answers gave examples for the effects of size and charge and relate endocrine responses to specific alterations in arteriolar tone and how this affected filtration. A detailed discussion of cardiovascular and endocrine responses to hypovolaemia was not required.
Some candidates confused clearance with filtered load. Candidates are reminded to write legibly – especially where subscripts and Greek letters are used. Directional arrows (if used) should correlate with text.
2018B 09
Describe the renal handling of water including the modulation of water excretion.
2008A 08
Outline the role of the kidney in body water homeostasis.
CICMWrecks Answer
- Total body water = 60% body weight (approx. 42L in 70kg male)
- Intracellular fluid = 2/3 Total body water (approx. 28L)
- Extracellular fluid = 1/3 Total body water (approx. 14L)
- Plasma compartment = 1/4 Extracellular fluid (approx. 3L)
- Renal blood flow
- 1.25l/min
- Filtration fraction
- 0.2
- Glomerular filtration rate
- 180l/day
- >4x total body water
- Therefore renal water reabsorption important in H2O homeostasis
Water reabsorption
Mechanisms:
- difference in osmolality lumen : interstitial fluid. This created by reabsorption solutes (Na, Cl, glucose, AA)
- solute reabsorb actively ⇒ ↓luminal osmolality & ↑interstitial osmolality ⇒ gradient to drive water reabsorb across cells +/- tight junctions
Locations of Water Reabsorption:
PCT
- Highly permeable to water
- 65% H2O reabsorption → follows Na+ reabsorption via osmosis
LoH
- Thin descending limb
- Highly permeable to water
- 20% H2O reabsorbed
- Follows ions via osmosis
- Thick ascending limb
- Impermeable to water
- Countercurrent mechanism to increase papillary osmolarity to ~1400mosm/l
- Important for further H2O reabsorption
DCT/CD
- Variable permeability
- Controlled by aldosterone and vasopressin
If decreased total body water
- Decreased renal blood flow (beyond autoregulatory capabilities)
- Decreased glomerular filtration
- Decreased Na reaching DCT macula dense
- Release of Renin from Juxtaglomerular apparatus → Angiotensin II → Aldosterone
Angiotensin II
- Direct upregulation of proximal tubular Na reabsorption
- Efferent arteriolar vasoconstriction (as well as weak afferent arteriolar constriction) → increased filtration
- Increased thirst sensation
- Stimulates aldosterone release
- Stimulates vasopressin release
- Potentiates post-ganglionic sympathetic stimulation
Aldosterone
- Upregulates CD Na/K ATPase and inserts ENaC channels to increase Na reabsorption
- H2O follows Na via osmosis
Vasopressin (ADH)
- Stimulated by Angiotensin II, Osmoreceptors and low-volume baroreceptors
- Causes insertion of aquaporin 2 via V2 receptor stimulation
- Increased permeability of CD to H2O
- H2O reabsorbed due to high interstitial osmolarity created by counter-current mechanism and reabsorption of urea
If increased total body water
- Atrial stretch → production of natriuretic peptides (ANP, BNP)
- Leads to decreased reabsorption of Na in PCT
- Leads to decreased reabsorption of H2O
Sakurai 2016
Examiner Comments
2018B 09: 37% of candidates passed this question.
This question required a brief introduction of the role the kidney plays in water balance; a more detailed description of how water is handled as it passes through the various segments of the nephron (glomerulus, PCT, Loop of Henle, DCT and Collecting Duct); the modulation of water excretion by the kidney due to ADH (vasopressin) and how this operates; and the stimuli (osmotic and non-osmotic) for ADH secretion. Although worth mentioning in the context of the effect they have on water movement through the kidney, detailed explanations of Starling’s forces in the glomerulus, and of the operation and maintenance of the counter-current mechanism, were not required. More important was describing the control of water reabsorption in the collecting ducts (and thus modulation of water excretion by the kidney) under the influence of ADH.
2008A 08: 1 candidate (33%) passed this question
The main concept required was that the renal excretion of water is basic to the maintenance of constant body water conditions. This renal water excretion is controlled by multiple factors that influence glomerular filtration and tubular reabsorption. Also the kidney has mechanisms that allow it to eliminate excess water by excreting a dilute urine or to conserve water by excreting a concentrated urine.
Better answers provided details of the large GFR and the renal tubular handling of water. Also the creation of the hyperosmolar medullary interstitium by the counter current multiplier system, the special characteristics of the Loop of Henle that cause solutes to be trapped in the renal medulla and the resulting delivery of a hypoosmolar tubular fluid to the collecting ducts. Finally the variation in water permeability of the collecting ducts under the influence of ADH.
Extra marks were awarded for details on ADH including its origin, secretion, regulation and mechanism of action and the concept of electrolyte free water clearance. Some candidates confused aquaporins with vasopressin receptors. Other candidates produced long and irrelevant descriptions of the renin angiotensin system which gained no extra marks.
Syllabus D1 2f
2015A 04
Describe the mechanisms by which water and electrolytes are reabsorbed across the renal tubules.
CICMWrecks Answer
This is a list of all the mechanisms by which the renal tubules reabsorb water and electrolytes.
Most substances involve multiple mechanisms, the answer is structured to describe the different transport “mechanisms” and hence the examples are not extensive.
Mechanism | Energy Expenditure | Electrochemical gradient | Example | ||||
---|---|---|---|---|---|---|---|
Diffusion | Passive diffusion | Molecule crosses a membrane to which it is permeable by diffusion | No | With | Most electrolytes and molecules at different levels of kidney (along with other mechanisms) - H+, NH4+, HCO3-, Urea | ||
Facilitated diffusion | Molecule crosses a membrane via a channel, without energy expenditure | No | With | Amino acid movement from tubules to blood | |||
Active transport | Primary | Primary active transport | Molecule crosses a membrane via a channel, with energy expenditure (ATP) | Yes | Against | 3Na+ /2K+ ATPase pump - Na from cell into interstitium, in exhange for K | |
Secondary | Symport | Molecule crosses membrane against its electrochemical gradient, with the energy being provided by the transport of another molecule (Co-transported) | Not directly | May be Passive or Active based on gradient of 2nd | sodium and an amino acid | ||
Antiport | Molecule crosses membrane against its electrochemical gradient, with the energy being provided by the transport of another molecule (Anti-transported) | Not directly | May be Passive or Active based on gradient of 2nd | Na+ / H+ antiporter in proximal convoluted tubule | |||
Ligand-gated ion channel | Binding of a ligand causes conformational change in membrane channel, allowing movement of ion across membrane | No | With | ENaC channel - Control resorption of Na Located in collecting duct | |||
Exocytosis | Substance packed in vesicle, moves to cell membrane, two membranes merge -> substance exits cell | Yes | Usually against | Exocytosis of Water by Aquaporin2 channel (regulated by vasopressin) | |||
Endocytosis | Cell membrane extends to engulf a substance or object, which is then contained in the cell within a vesicle | Yes | Can be with, against or refer to a macroscopic object | Reabsorption of filtered proteins by receptor-mediated endocytosis in the proximal tubule | |||
Reabsorption | Multi-mechanism: Starling forces, diffusion, and active transport. | Depending on mechanism | Depending on mechanism | Resorption of glucose, water | |||
Osmosis | Spontaneous net movement of solvent molecules through a selectively permeable membrane into a region of higher solute concentration, in the direction that tends to equalize the solute concentrations on the two sides (Diffusion of water across semi-permeable membrane) | No | Yes | Water | |||
Solvent Drag | solutes in the ultrafiltrate that are transported back from the renal tubule by the flow of water rather than specifically by ion pumps or other membrane transport proteins. Occurs in paracellular pathways between tubular cells | Usually No | NA | Renal Na and Cl resorption, Renal urea handling |
JC 2019
Examiner Comments
2015A 04: 33 % of candidates passed this question.
The intent of the question was to have the candidate describe in the context of a classification the mechanisms by which water and electrolytes may cross a cell membrane and use the renal tubule to provide an example of each mechanism.
It was expected the answer would talk about transport “mechanisms” across membranes. These would include processes such as reabsorption, diffusion, facilitated diffusion, primary and secondary active transport, endocytosis, osmosis and solvent drag. Many candidates used colloquial and vague language to describe precise concepts.
Some candidates structured their answer as an outline of the principal mechanisms at each segment of the tubule. Thus there was repetitive reference to mechanisms without a description as requested in the question. This approach also resulted in some candidates omitting some mechanisms altogether.
2018A 04
Describe the renal handling of sodium.
2014B 23
Describe the regulation of sodium in the body.
2009B 22
Describe how the kidney handles sodium. (50 marks).
What factors influence urinary sodium excretion (50 marks)
CICMWrecks Answer
NORMAL SODIUM
- Total Sodium (Na) = 4000 mmol (60 mmol/kg)
- Distributed:
- Bone (45%)
- ECF (50%)
- 1°ly found in ECF (major EC cation)
- ICF (5%)
- 10-15 mmol/L maintained by
- Na+/K+ ATPase
- low gNa → prevents influx of Na
- Role of Na
- Main determinant of ECF osmolality and tonicityNa/Cl ~ 90% ECF osmotic solute load
- Main determinant of ECFV
- Depolarisation in action potential 2° to ↑Na conductance
- Co-transport of substances across membranes (Eg. Glucose)
- Involved in Na+/K+ ATPase in cell membranes
- Total output =Total input
- 1-1.4 mmol/kg/day
- ~100-300 mmol/day in 70 kg adult
- 10.5 g/day
- Sodium Control – Lost via:
- Kidneys (main) ~ lose 150 mmol/day
- Filters 25000 mmol Na+/day
- 99.5% reabsorbed
- 65% PCT, 25% TAL of LoH, 5% EDCT, 4-5% LDCT and CD
- Sweat and GIT loss in faeces ~ lose 10 mmol/day (0.25 g/day each)
- Kidneys (main) ~ lose 150 mmol/day
Sodium Reabsorption in Kidney
Location | Contribution | Mechanism |
---|---|---|
PCT | 65% | Secondary active transport: – Luminal Na/organic cotransporters with glucose and AAs – Luminal Na/K (NHE-3) exchanger with H from Henderson-Hasselbach intracellularly Passive transcellular – Via solvent drag passively – Down electrical gradient from positive lumenal charge |
TAL of LoH | 25% | Secondary Active transport: – NKCCT on luminal surface – Dominant mechanism Small amount continues via Secondary active transport as per PCT Paracellular movement driven by net positive charge in lumen |
Early DCT | 6-10% | 2° active means – Apical Na/K ATPase generates Na gradient – Basal Na/Cl symporter – No alteration in the luminal charge as electrically neutral |
Late DCT and CD | 5-10% | – Facilitated diffusion across principle cells – Basolateral Na/K ATPase → intracellular Na deficit – Na reabsorbed from lumen via ENaC channels in principle cells up regulated by Aldosterone |
Overview of renal Na+ regulation:
- Thus, renal Na+ regulation depends on:
- Degree of glomerular filtration of Na+ → GFR (minor)
- Changes in GFR due to hyper or hypovolaemia will (indirectly) adjust sodium elimination. Increased plasma volume increases GFR, and vice versa.
- Degree of tubular reabsorption of Na+ (major)
This is the main mechanism for controlling sodium in euvolaemia.
In terms of long term Na excretion; Na reabsorbed is more impt than GFR because:- (i) GFR is heavily autoregulated
- (ii) Glomerulotubular balance blunts any major changes in Na+ excretion that would have resulted from minor changes in GFR changes that actually occurs
- Na reabsorption through GIT/sweat/salivary glands:
- Varies with diet and exercise
- Mainly action of Aldosterone via Na/K ATPase
- Degree of glomerular filtration of Na+ → GFR (minor)
- Normal values
- Na+ filtration: 140mmol/L x 180L/day = 25000mmol Na/day
- Na+ excretion: 140mmol excreted
- rest reabsorbed
- Fractional excretion = 0.5%
Na+ regulation: Control of GFR
- Intrinsic autoregulatory factors (tubuloglomerular feedback and myogenic mechanism)
- MAP has minor effect on GFR over MAP range 70-175 mmHg → BUT changes
in BP that invoke baroreceptor reflexes (BRR) can override these autoregulatory
mechanisms → alter GFR and amount of Na+ filtered
- MAP has minor effect on GFR over MAP range 70-175 mmHg → BUT changes
- Extrinsic factors: Body Na+ content (via ECFV)
- Direct renal effects – ↓ [Na+] (or ↓ ECFV) → results in ↓ GFR due to a ↓ glomerular capillary P(HYDROSTATIC) and ↑ glomerular capillary P(ONCOTIC) → ↓ GFR and Na+ filtered
- Indirect renal effects – ↓ [Na+] (or ↓ ECFV) → stimulates arterial, venous and cardiac BRR → neurohormonal response → to ↓ GFR and Na+ filtered via:
- (i) ↑ SNS and RAAS activity → cause afferent and efferent arteriolar constriction and mesangial cell contraction
- (ii) ↑ ADH → cause afferent arteriolar constriction and mesangial cell contraction
- (iii) ↓ ANP → inhibit afferent arteriolar dilation and mesangial cell relaxation
Na+ Regulation: Control of Reabsorption
- Glomerulotubular balance:
- Intrinsic autoregulatory mechanism that minimises the effect of changes in GFR on Na+ and H2O excretion
- It functions on the basis that the PCT reabsorbs a constant proportion of glomerular filtrate (65% of filtered Na+ /H2O), rather than a constant amount
- In effect – ↑ GFR = ↑ filtration of Na+/H2O = ↑ Na+/H2O reabsorption
- Mechanism:
- With ↑ GFR → large amount of plasma is filtered at the glomerulus → leads to ↑ π(ONCOTIC) of plasma in peritubular capillaries
- This results in an ↑ gradient that –
- (i) Favours tubular reabsorption, and
- (ii) Counteracts the effect of ↑ GFR on fluid leaving the PCT
- Renal interstitial hydrostatic pressure (Intrarenal physical factors)
- ↓ ECFV (and ↓ Na+) results in ↓ MAP → leads to (i) ↓ PHYDROSTATIC and (ii) ↑ πONCOTIC of peritubular capillaries → thus, ↑ Na+ (and ↑ H2O) reabsorption from tubular interstitium into peritubular capillaries
- Hormonal Influences:
- Renin
- Released by ↓ Na delevery to macula densa or β1 stimulation secondary to volume underload
- Tubular effects:
- increased PCT Na/Cl reabsorption, increased tubular K secretion
- Direct PCT effect
- Aldosterone release
- Aldosterone
- Most important regulator of Na+ reabsorption
- Alters protein translation (inducing production of tubular basolateral Na+/K+ATPase and luminal ENaC and K+channels) → causes ↑ Na+ reabsorption by DCT and Principal cells of CCD
- Increased Na reabsorption throughout the GIT/sweat and salivary glands via Na/K ATPase
- Increased H2O reabsorption and increased Na via solvent drag
- Angiotensin II
- Negative feedback on renin release
- Increased aldosterone release
- Decreased RBF and GFR
- Direct renal arteriole constriction (efferent = afferent)
- Mesangial cell contraction thus decreased Kf and GFR
- Direct stimulation of Na+ reabsorption at PCT, and
- Indirect stimulation of Na+ reabsorption via SNS, AII, and aldosterone
- SNS
- Direct stimulation of Na+ reabsorption at the PCT (α1 and β1 receptors), and
- Indirect stimulation of Na+ reabsorption via RAAS
- ADH
- → ↑ Na+ reabsorption at the CCD (principal cells) → acts synergistically with aldosterone here
- ANP
- Inhibition of Na+ reabsorption (blockage of ENaC) in the CDs
- ↓ RAAS and ↓ ADH activity
- Renin
- Other causes ↑Na reabsorb:
- Cortisol
- Oestrogen
- GH
- Thyroid hormone
- Insulin
- Dopamine
- Other cause ↓Na reabsorb:
- PGE2 inhibits NaK ATPase to reduce Na reabsorption
- Glucagon
- Progesterone
- PTH
- Renal vasoDilators:
- PGs
- Kinins
- Pressure natriuresis & diuretics
- renal compensatory mechanism that maintains long-term regulation of arterial BP by controlling the kidney’s excretory ability of Na+and H2O
- Pharmacological agents:
- Ouabain (a cardiac glycoside) inhibits NaK ATPase decreasing excretion
- Loop Diuretics → ↑ Na loss
Gladwin / Bianca / JC 2019
Examiner Comments
2018A 04: 6% of candidates passed this question.
A description of filtration and reabsorption, including amounts was required. Better answers described sodium handling in a logical sequence as it progressed through the nephron including the percentages reabsorbed in each segment. In addition to the amounts reabsorbed, the mechanisms of transport across the tubular luminal and basolateral membranes into interstitial space should have been described.
2014B 23: 19% of candidates passed this question.
This question was generally poorly answered. Total body sodium is regulated within 2% in normal individuals. The vast majority is contained in the extracellular compartment. While any physiological regulation involves a balance of input and output, sodium intake is essentially unregulated in humans. Output is regulated via renal, gastrointestinal and skin losses. Candidates needed to present the renal handling of Na including hormonal control and present factual knowledge about the level of absorption and GFR effects to attain a pass mark. Many candidates focused on osmolality and tonicity and some on the use of diuretics thereby not gaining marks
on regulation of sodium. Most candidates didn’t mention either the skin and GIT role in sodium balance.
2009B 22: 2 (22%) of candidates passed this question.
Candidates were essentially expected to describe the fate of sodium as t passes through the kidney from filtration at the glomerulus to ending up in the urine. Essentially, most Na+ is reabsorbed at the proximal tubule (65%), reabsorption being down an electrochemical gradient (inside cell negative and low Na+ concentration) which is maintained by active Na+/K+ ATPase activity at the basolateral membrane. Then Loop of Henle reabsorbs a further 15% of filtered Na+. At descending limb – no Na+ reabsorption. At ascending limb, thick segment – active process as per proximal tubule, but mostly coupled to K+ and Cl- and paracellular diffusion through tight junctions. Finally the distal convoluted tubule and collecting ducts, a further almost 20% reabsorbed – leaving < 1%
Factors influencing loss are
- aldosterone = stimulates Na+ reabsorption at the collecting tubules
- intra renal factors such as interstitial pressure which is lowered during hypovolaemia and reduced renal perfusion, thus promoting Na+ reabsorption gradient
- sympathetic nervous system – influences interstitial pressure and increases renin production
- angiotensin II – stimulates reabsorption at proximal tubule
- Atrial Naturetic Peptide/Factor – inhibits Na+ reabsorption
- Others – dopamine, cortisol, insulin => increase Na+ reabsorption, but minor factors
Syllabus – D1, 2f
Reference – Textbook of medical Physiology, Guyton, Chp 28
2023A 05
Describe renal handling of potassium (60% of Marks), including physiological factors that may influence it (40% of Marks).
2021A 08
Describe renal handling of potassium (60% marks),including factors that may influence it (40% marks).
2010B 16
Discuss the regulation of body potassium by the kidney.
CICMWrecks Answer
Potassium
- Predominany intracellular cation
- Total Stores: Approx 3200mmol (50mmol/kg)
- Key Functions:
- Main determinant of ICF osmolality and tonicity
- Responsible for RMP of excitable cells via Goldmann-Hodgkin-Katz due to ↑↑gK relative to other species
- Role in action potential → repolarisation phase
- Secretion of insulin and multiple other KATP dependant processes
- Regulation of IC processes (protein/glycogen synthesis)
- Involved in Na+/K+ ATPase in cell membranes
Potassium Elimination
- Faecal – 8mmol/day
- Renal – 92mmol/day (See next heading for details)
- Urinary K+ excretion = [K+ filtered by glomerulus] + [K+ secreted by CCD/LDCT] – [K+ reabsorbed by renal tubules]
- Glomerular filtration: Freely filtered = 756mmol/day (180L x 4.2mmol/L)
- PCT: 65% reabsorption
- LoH: 25~30% reabsorption
- DCT/Collecting Ducts – variable
- Determined by Aldosterone, Plasma [K+], Tubular Flow rate
- Secreted by principal cells
- Reabsorbed by intercalating cells
K+ handling within the tubular system:
- PCT (55% of filtered K+ is reabsorbed):
- K+ is reabsorbed passively via the paracellular route due to:
- Solvent drag → coupled to flow of Na+ and H2O
- [ ] gradient → created by reabsorption of H2O
- Electrical gradient → due to +ve luminal charge in late PCT
- K+ is reabsorbed passively via the paracellular route due to:
- TAL of LoH (30% of filtered K+ is reabsorbed):
- K+ is reabsorbed by 2° active transport via paracellular route → +ve charged lumen generated by ion flux created by NKCCT transporter causes K+ to be reabsorbed by diffusion down its electrical gradient
- LDCT and CCD (0 to > 15% K+ is secreted):
- Principal cells of CCD and LDCT → 2° active secretion K+ → via:
- Basolateral Na+ /K+ ATPase → (i) generates an electrochemical gradient that draws Na+ intracellularly from the tubular lumen (via the ENaC channel), and (ii) pumps K+ from peritubular capillaries into tubular cell
- The –vely charged lumen generated by influx of Na+ across ENaC channel → favours tubular secretion of K+
- o Type A intercalated cells of CCD and LDCT → 2° active reabsorption of K+ :
- H+ is produced within tubular cell by hydration of CO2 (using CA) → H+ is then exchanged for tubular K+
- Principal cells of CCD and LDCT → 2° active secretion K+ → via:
- MCD (5% of filtered K+ is reabsorbed)
Renal K+ regulation:
- Renal K+ regulation occurs MAINLY via control of K+ secretion at the distal nephron (CCD and LDCT) via the following factors:
- Circulating factors:
- Plasma [K+ ]
- ↑ [K+ ] causes ↑ K+ secretion by → (i) Directly stimulating Na+ /K+ ATPase in principal cells, and (ii) Directly stimulating Aldosterone release from adrenal cortex
- Aldosterone
- ↑ aldosterone causes ↑ K+ secretion by → ↑ production of Na+ /K+ ATPase, K+ channels and ENaC Na+ channels in the principal cells
- Plasma pH
- Alkalosis via ↓ plasma [H+ ] causes ↑ K+ secretion by → stimulating Na+ /K+ ATPase in principal cells
- Plasma [K+ ]
- Luminal factors:
- Flow of tubular fluid in DCT and CCD
- ↑ tubular fluid flow causes ↑ K+ secretion by → maintaining ↓ luminal [K+ ] (Ie. continuously washing it away) → permits passive diffusion of K+ ↓ its [ ] gradient into tubular lumen
- ↑ Na+ delivery rate to DCT and CCD → ↑ K+ secretion
- -ve lumen potential difference → ↑ K+ secretion
- Flow of tubular fluid in DCT and CCD
- Circulating factors:
JC / Gladwin / Sakurai / Bianca 2020
Examiner Comments
2023A 05: 33% of candidates passed this question.
This question related to the renal handling of potassium, the physiology of potassium in the rest of the body was not relevant. Ultimately net potassium flux is a function of filtration, reabsorption, secretion and excretion. Good candidates divided the nephron into relevant sections and described how potassium was handled in each section. They correctly described the percentage reabsorption along each section, as well as the relevant active and passive pathways for reabsorption and/or secretion including the cells and channels/pumps involved. The physiological factors regulating each of these mechanisms were then described in correct detail.
2013A 12
Potassium is the second most common cation in the body and the main intracellular cation. It is widely distributed and has many important roles. Maintenance of potassium balance depends mainly on secretion by the kidneys in the distal and collecting tubules. Candidates were expected to mention the influence of aldosterone, and other hormones such as glucocorticoids, catecholamines and vasopressin have as well as factors such as acidosis/alkalosis. Candidates who had a systematic and structured approach performed better.
2010B 16: 4 (27%) of candidates passed this question
A number of candidates discussed the distribution of potassium in the body and its role in membrane potentials. This was not asked for. Common omissions were a lack of comment on glomerular filtration, a lack of detail regards mechanisms of potassium transport in various parts of the glomeruli and failing to discuss control mechanisms other than aldosterone.
Syllabus: E1,2b, D1,2f
References: Guyton and Hall Textbook of Medical Physiology, Chp 29
2020B 19
Explain how the kidney handles an acid load.
2014B 12
Describe the role of the kidneys in the excretion of non-volatile acid.
CICMWrecks Answer
Non-volatile acid
” An acid produced from sources other than carbon dioxide, which cannot be excreted in the lungs. “
- e.g. lactate, sulphate, phosphate and ketone bodies
- 70-100mmol non-volatile acid produced per day
- 4000-5000mmol HCO3– filtered by glomerulus per day
- Renal acid-base is largely determined by handling of bicarbonate ions
- 80% of filtered bicarbonate resorbed in proximal tubule
- 20% resorbed in distal tubule and collecting duct
- Urinary Buffers (Dibasic Phosphate and ammonia) play a role
Bicarbonate Resorption
Proximal tubule
In the tubular cell:
- H+ leaves the tubular cell through the apical membrane via
- H+/Na+ antiporter (secondary active transport via Na+/K+ ATPase on basolateral membrane)
- The HCO3– re-enters the circulation via the Na/HCO3– symporter
- H+ combines with HCO3– in tubule to form H2CO3
- H2CO3 switches back to CO2 and H2O, CO2 is resorbed to turn back into the tubular cell to generate more HCO3 for resorption
More bicarbonate resorbed when:
- Higher filtered HCO3–
- Higher PaCO2
- Lower luminal flow rate
- Angiotensin 2
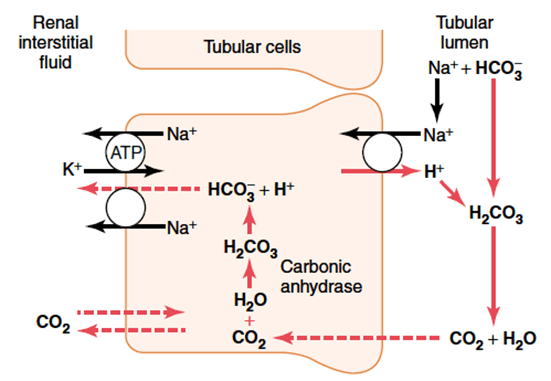
Distal convoluted tubule and collecting duct
- Similar mechanism with regards to resorption of HCO3– via secretion of H+
- Difference is that H+ is transported across the apical membrane via an H+ ATPase (active transport)
Role of Buffers
- The maximum urinary pH is 4.5
- To eliminate more protons, they must be buffered in the tubular fluid
- H+ is bound preferentially to buffers only in the presence of low tubular HCO3–
- Buffering, and so elimination of H+ without its conjugate HCO3–, requires resorption of HCO3– in the proximal convoluted tubule
Phosphate (HPO42-):
- Becomes concentrated in tubules
- pKa = 6.8, so operates effectively as a buffer at tubular pH
Ammonia
- Glutamine broken down in tubular epithelia cells to two NH4+ and two HCO3–
- Comes from hepatic amino acid metabolism
- The new HCO3– is transported back to the circulation via the Na/HCO3– symporter to re-enter the circulation
- NH4+ becomes ion trapped in the tubular fluid
- Proximal tubular fluid is not acidic enough to keep NH4+ in the tubule
- It is resorbed in the ascending limb, moves through the medulla to re-enter the collecting duct
- Here urine has a low pH, and so NH4+ can be eliminated with its proton attached
Mooney 2016
Examiner Comments
2020B 19: 51% of candidates passed this question.
This question required candidates to understand the renal response to an acid load. It was expected that candidates would answer with regard to recycling of bicarbonate in the proximal tubule, excretion of titratable acid via the phosphate buffer system and generation of ammonium and its role in acid secretion. Many candidates had a good understanding of the bicarbonate system but used this to explain the secretion of new acid.
2014B 12: 27% of candidates passed this question.
This is a complex but essential area of physiology for intensive care practice. It was expected candidates would indicate that non-volatile acids are those not able to eliminated by the lungs (lactate, sulphate, phosphate and ketone bodies). The kidney plays a central role via bicarbonate (resorbing filtered bicarbonate and generating “new” bicarbonate = acid excretion). It was expected candidates could detail the processes in various parts of the renal tubules and the role or urinary buffers (dibasic phosphate and ammonia). While a number of candidates were able to provide some of the details of ion transports in the kidney, few showed understanding of the overarching concepts of the proximal bicarbonate reabsorption being necessary to allow the distal acid excretion.
2010B 12
Describe the renal handling of bicarbonate and the changes in urine pH along the nephron. (80% of marks)
How is this affected by hypoventilation? (20% of marks)
CICMWrecks Answer
Total filtered (24×180) = 4320 mmol/day
Completely reabsorbed
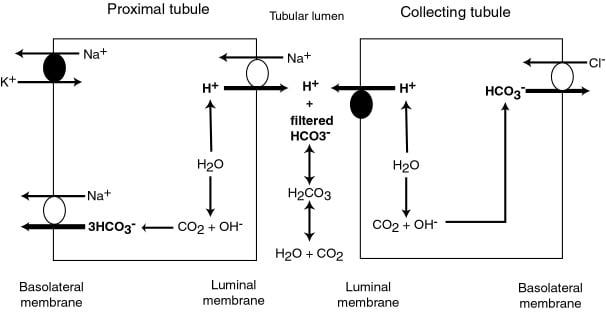
PCT (85%) / TAL (10%)
- Absorbed as CO2 after conversion by C.A. 4 on brush border
- Converted back via CA2
- Reabsorbed to peritubular capilaries via Na/HCO3 cotransporter
DCT/CCD (5%)
- Type A intercalated cells
- Secrete H via H-ATPase or H/K ATPase
- ↑ by aldosterone
- HCO3 is absorbed into peritubular capilary
- Secrete H via H-ATPase or H/K ATPase
- Type B intercalated cels
- Secrete HCO3 via luminal Cl/HCO3 exchanger
- Secrete HCO3 via luminal Cl/HCO3 exchanger
pH changes
- PCT pH = 7.4.
- Reabsorption of Bicarb → pH ~ 6.8 at descending limb of LoH
- End of DCT → pH ~ 4.4
Hypoventilation:
- ↓RR → ↑PaCO ₂ → acidosis
- Chronic Acidosis (traditional)
- → ↑ secretion of titratable acids (phosphate, Ammonia)
- → Binding and secretion of additional H
- → net ↑[HCO3] reabsorption in tubular cells (traditional model)
- Chronic Acidosis (stewart)
- ↑ ammonigenesis → ↑DCT ammonia assoc with ↑Cl secretion via luminal Cl/HCO3 exchanger → ↑SID → defense of acidosis.
Mooney 2016
Examiner Comments
2010B 12: 4 (27%) of candidates passed this question.
This question sought knowledge of an important and basic area of physiology that applies to many circumstances encountered in daily intensive care practice. For a good answer candidates were expected to mention that bicarbonate is freely filtered, its fate along the nephron and that it is not normally found in the urine. Mechanisms by how it is reabsorbed and generated along the different segments of the nephron were expected to be described in some detail. The last part of this question relating to hypoventilation was poorly answered. For a good answer candidates were expected to mention that Hypoventilation results in an increase in arterial PCO2 that readily diffuses into tubular cells resulting in increased intracellular H2CO3 and subsequently bicarbonate, that is reabsorbed, and H+ that is secreted.
Syllabus: D1 Renal Physiology, 2e, 2k
References: Ganong, Review of Medical Physiology, Ch 39 and 40
2016A 08
Describe the characteristics of a drug that influence its excretion by the kidneys.
CICMWrecks Answer
Excretion by kidneys can be influenced by drug characteristics
1) Which affect filtration at glomerulus
2) Which affect secretion into tubules
3) Which affect reabsorption in the tubules
1) Which affect filtration at glomerulus
Filtration at the glomerulus – Dependent on Filtration fraction [GFR / RBF] and net starling force.
Normal filtration is 180 L/day (20% of renal plasma flow)
- Protein binding:
- Only free drug present in filtered plasma will be excreted.
- Concentration of filtered drug will be the same as in unfiltered plasma
- Highly protein bound drugs are poorly filtered
There is only a weak concentration gradient favouring dissociation from plasma proteins.
- Molecule size:
- Size:
- Molecules larger than 30 Angstrom is not filtered
- Weight:
- Substances less than 7,000 Da are freely filtered
- Substances greater than 70,000 Da are essentially impermeable
- Size:
- Hydrophilic/lipophobic
Lipophilic drugs may be filtered at the glomerulus but will be freely reabsorbed during their passage down the tubule, such that only trivial amounts are eliminated in urine. - Charge:
- Positive ions are filtered (Glomerulus is slightly negative charged)
2) Which affect secretion into tubules
Active process, allows secretion against concentration gradients
- Acid/Base:
- Saturable process – Saturation may occur of a basic transporter whilst still allowing excretion of acidic drugs, and vice versa.
- Size:
- Molecules which are too large to be filtered in the glomerulus may still be cleared renally by these mechanisms
- Protein binding:
- Protein-bound drugs are not cleared this way; only the unbound fraction is available for active secretion.
3) Which affect reabsorption in the tubules
Passive diffusion down a concentration gradient.
- Hydrophilic molecules can only be reabsorbed by a specialised transport mechanism
- Drug ionization:
- Only non-ionised drug can be reabsorbed passively
- Acidic drugs will become ionised in an alkaline urine (and vice versa), reducing their solubility
This is the physiological justification for urinary alkalinisation.
JC 2019
Examiner Comments
2016A 08: 29% of candidates passed this question.
Drug characteristics that might influence the renal excretion processes include charge, size, solubility, and binding to specific structures or protein. Whether the drug is unchanged versus metabolised can influence these factors.
This question tests core knowledge of pharmacology principles and should be answered with equations, graphs or simple clear descriptions of physical and chemical principles. Extended examples and hedged statements about “influencing” without the direction, magnitude and necessary conditions for the influence did not score marks
2011B 03 – 2010A 08
Describe the role of the kidney in drug excretion and the factors affecting this (80% marks).
Briefly outline how you would alter the dosing of a drug with high renal excretion in a patient with renal impairment (20% marks)
2008A 17
Describe the role of the kidney in drug excretion, and the factors affecting this.
Briefly outline how you would alter the dosing of gentamicin in a patient with renal impairment.
CICMWrecks Answer
Renal Drug Excretion
Due to glomerular filtration and balance of secretion and reabsorption
Glomerular Filtration
- Physiological factors
- Starling forces
- Filtration pressure ∝ (PG – PBC) – ( πG – πBC)
Where- PG = Glomerular hydrostatic pressure (60mmHg)
- PBC = Bowman’s Capsule hydrostatic pressure (18mmHg)
- πG = Glomerular oncotic pressure (32mmHg)
- πBC = Bowman’s Capsule oncotic pressure (Negligible)
- Filtration decreases
- Shock → decreased glomerular pressure
- Obstruction → increased bowman’s capsule hydrostatic pressure
- Hypoproteinaemia → hepatic failure, nephrotic syndrome
- Fick’s Law (see eqn)
- Filtration increases with decreased MW, increased concentration gradient
- Filtration decreases with increased GBM thickness (glomerulosclerosis, deposition) and loss of glomerular surface area (usually 0.8m2. may be lost with renal failure)
Fick’s Law:
- Drug factors
- Size (Due to filtration slits and podocyte foot processes)
- Particles <4nm freely filtered
- Particles >8nm excluded
- Charge
- GBM has negative charge, therefore negatively charged molecules repulsed → decreased filtration
- Protein binding
- Highly protein bound drugs inhibited from filtration due to large size and charge of proteins
- Size (Due to filtration slits and podocyte foot processes)
Secretion
- Due to specific transporters
- P-glycoprotein (Amphipathic anions)
- MPR-2 (Conjugated metabolites)
- ATP-Binding Cassette Transporters (Organic cations)
Reabsorption
- Passive reabsorption occurs in PCT and DCT
- Membrane transporters for facilitated transport are present in DCT
- Ionization
- pH of urine may lead to drug “trapping”
- Acids ionized with pH > pKa
- Bases ionized when pH < pKa
- pH of urine may lead to drug “trapping”
- Lipophilicity
- Allows permeation through phospholipid bilayers of cell membrane → reabsorption
Dose adjustment of renally excreted drugs
- According to clearance and GFR
- Loading dose = Target concentration x Vd
- Usually no alteration of loading dose required
- In ESRF, volume of distribution may be increased → loading dose may need to be increased
- Dosing rate = Target concentration x Cl
- As clearance is reduced in renal failure dosing rate requires reduction
- Maintenance dose = Dosing rate x Dosing interval
- Maintenance dose and/or the dosing interval may need to be reduced (depending on whether AUC or peak concentration of drug is required for drug effect)
- Plasma monitoring of [drug] concentration required for drugs with low therapeutic index
Dose adjustment of Gentamicin
MoA: Inhibition of bacterial protein synthesis through irreversible binding to the 30s bacterial ribosome
Has a narrow therapeutic window, hence dose optimization and therapeutic drug monitoring are crucial.
Concentration dependant killing | Active concentration needs to be acheived (~8-10 times Minimim Inhibitory Concentration/MIC) Time above MIC does not need to be maintained | Loading dose should not be altered even in renal failure (5-7mg/kg) |
Significant postantibiotic effect Distribution: Hydrophilic, so Distribute mainly to extra-cellular fluid. Redistribution can occurs upto 16-24 hours. | Once daily dosing sufficient without renal failure | |
ESRF – Volume of distribution theoretically larger (but in case of Aminoglycosides, might be lower – suspected due to tissue displacement by other molecules like urea) | Loading dose adjustment in ESRF 4mg/kg | |
Metabolism: Nil Excretion: Rapidly excreted by glomerular filtration. Hence accumulate in renal failure (t1/2 upto 30-60hrs) | Subsequent Dosing interval based on renal clearance (GFR / CrCl) Frequent dosing only increases toxicity with no improvement in efficacy. | Maintenance dosing less frequent in renal failure Dose interval based on pre-existing guidelines, and with monitoring of pre-administration levels CrCl 40 to 59 mL/minute: Administer every 36 hours CrCl 20 to 39 mL/minute: Administer every 48 hours CrCl <20 mL/minute: Monitor serum levels and redose when gentamicin level is less than 1 mg/L |
End stage renal disease / Dialysis (t1/2 upto 100hrs) | Check level prior to dialysis, and only administer dose after dialysis (based on expert advice) | |
Low Therapeutic index | Frequent monitoring of levels required | |
Sakurai / JC 2019
Examiner Comments
2011B 03: 13 (52%) of candidates passed this question.
It was expected candidates would expand on the role of the kidney in the excretion of drugs and metabolites. A statement that referred to the classical features of drugs that undergo renal excretion (eg polarity, lipid solubility, size and protein binding, drug metabolites) and a definition of renal clearance was expected and would have provided an excellent introduction to any answer. It was then expected that candidates would mention, in some detail, the processes of filtration and secretion (both active and passive and both proximal and distal along the renal tubules). The question also asked for factors that affect renal drug excretion, for example, a reduction in GFR or alteration in protein binding. An approach to alterations of dosing would require some consideration of assessing degree of dysfunction (GFR estimation / calculation) then an understanding that it would not impact on loading doses but would influence subsequent dosing of renal cleared drugs. Plasma monitoring provides useful information for some drugs, particularly those with a narrow therapeutic index.
Syllabus: II2d, D12h
Recommended sources: Goodman and Gilman’s the Pharmacological Basis of Therapeutics pgs10-14; Foundations of Anaesthesia Basic and Clinical Science, Hemmings pg107; Basic and Clinical Pharmacology, Katzung pgs 35, 48-49.
2010A 08: 0 (0%) of candidates passed this question
The preponderance of marks was allocated to a discussion of renal drug excretion and factors altering this function. Detail was expected including a definition of clearance, and the balance between filtration / secretion / reabsorption in the tubules. Specific mention of GFR, molecular weight of filterable compounds, protein binding, and charge effects determining filtration at the glomerular level was anticipated. Tubular transport mechanisms for secretion and reabsorption in the proximal and distal tubule should have been included in the discussion.
Candidates needed to cover factors which alter GFR, competition for transport proteins, changes in pH on drug elimination, and disease states in answering the question.
An understanding that drug dosing should be based on estimating creatinine clearance and plasma concentration monitoring was essential. Loading dose is usually unaltered. However, maintenance dose and dosing interval need to be adjusted owing to an increased half-life. Many candidates did not emphasise the need to increase dosing interval as well as reduce maintenance dose in renal impairment.
Syllabus: II2d, D12h
References: Goodman and Gilman’s the Pharmacological Basis of Therapeutics p10-14. Foundations of Anaesthesia Basic and Clinical Science, Hemmings p107.
Basic and Clinical Pharmacology, Katzung p35, 48-49.
2008A 17: No candidates (0%) passed this question.
The main points candidates were expected to cover included a brief definition of renal clearance followed by a description of the drug factors that affect this (filtration, secretion and reabsorption), recognition that GFR and protein binding was important in the answer. A brief description of gentamicin kinetics that affect dosing regimens and a statement that dosing would be guided by calculated GFR and measured drug levels wold round out a good answer. Correct elaboration of the above factors were rewarded with additional marks.
Candidates failing this question submitted answers where concepts were randomly mentioned with no attempt to integrate these into a cohesive answer that demonstrated an understanding of the topic. Writing random words without examples or explanations did not demonstrate sufficient understanding to be rewarded with marks. Again, many answers lacked sufficient detail in the answer.
Syllabus – Pharmacokinetics
iv. Explain the counter-current mechanisms in the kidney.
2023B 03 Describe the physiological mechanisms by which the kidney is able to concentrate urine.
2022B 07 Describe the physiological mechanisms by which the kidney is able to maximally concentrate urine.
2020A 04 Explain the counter-current mechanism in the kidney.
2015B 22 Describe the counter-current mechanisms in the kidney (60% of marks) and in the skin (40% of marks).
2011A 09 Describe how the kidney maintains the medullary concentration gradient.
CICMWrecks Answer – Kidney counter-current
Medullary concentrating gradient
- physiological process which sets up a concentration gradient from cortex through to medulla
- allows formation of concentrated urine
- Mechanisms:
- Counter current Multiplier: creates concentrated medullary interstitium
- Counter current Exchange (vasa recta): maintains intersisital osmotic gradient
- Recycling of urea: contributes to high osmolarity of medullary interstitium
- Normal cortico-medullary gradient 300-1400mOsmol.
Counter-current Mechanism in Kidney
Counter Current Multiplier:
Set-up
- Descending limb LoH Permeable to water only
- Ascending limb LoH Permeable to NaCl only
- Thin passive NaCl reabs
- Thick NaCl via active NKCCT cotransport and paracellular
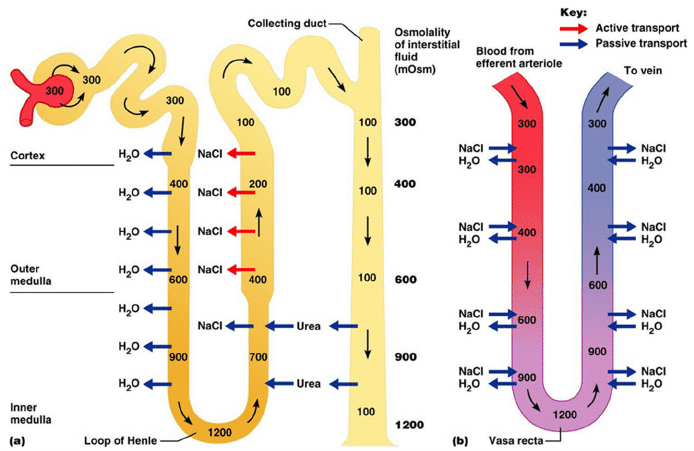
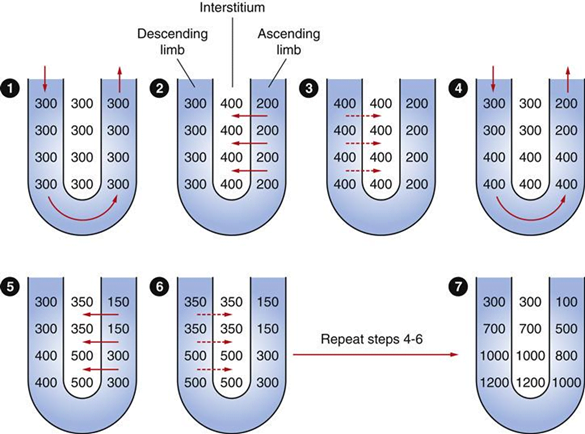
Generation
- Enters LoH osmolarity throughout = 300mOsm
- Max effort of NKCCT = 100mOsm/kg
- Tubular OSM = 200/kg
- Interstitial Osm = 400/kg
- Descending limb diffuses water down gradient
- Descend limb OSM now 400
- Process repeats (flow, pump salt, equilibrate water)
Counter Current Exchange:
- Hairpin loop arrangement of the vasa recta in the juxta-medullary nephrons.
- Provides blood flow to medullary tissue without impact of the cortico-medullary gradient.
- Relies on slow flow of blood.
- Mechanism
- On descent, water is lost from capillaries and NaCl is absorbed into capillaries increasing osmolarity
- On ascent water is reabsorbed and solute is lost
Role of Urea in Medullary Concentration Gradient
- 900mmol/day filtered
- 350-550mmol/day reabsorbed.
- Comprises 50% of osmolality (300-650 mOsm/kg)
- Freely filtered at the glomerulus
- Secreted by thin limbs of LoH down concentration gradient from medullary interstitium to tubular fluid.
- As water and Na are reabsorbed, tubular [Urea] increases
- [Urea] = >500mmol/L at collecting ducts
- Equilibrates with interstium due to slow flow rates.
- ↑ADH
- urea transporters UT1 and UT3 insertion → ↑ CD permeability to urea
- → ↑ medullary gradient (further 10% reabsorption if required)
Gladwin / JC 2020
CICMWrecks Answer – Skin counter-current
Counter-current Mechanism in Skin
- Counter current mechanism in skin
- Unknown significance in man
- In arctic fox
- Deep veins run parralel to and adjacent to arteries
- Heat from arterial blood is reabsorbed by venous blood returning to the body
- minimizes heat transfer to periphery and subsequent heat transfer to the
environment
- Counter-current system within the limbs
- one of the mechanisms to keep core temperature fairly constant
- the warm outbound arterial blood transfers heat to the cold inbound venous blood
- Three types:
- One artery and one vein in parallel
- as exist down the lengths of our arms and legs,
- One artery surrounded by many veins
- as in human fingers
- A net where 20–40, or sometimes several hundred, small arteries and veins run parallel and are intermingled
- Extremely rare in humans – usually pathological
- Extremely rare in humans – usually pathological
- One artery and one vein in parallel
Gladwin / JC 2020
Examiner Comments
2022B 07: 35% of candidates passed this question.
Answering this question well required the demonstration of understanding of the concept of achieving maximum urinary concentrating ability. Answers required a description of the usual concentrating processes and the changes that would occur in circumstances where maximally concentrated urine would be made. A key concept was the creation of a medullary concentration gradient to allow water reabsorption independent of solute reabsorption. This required an explanation of the contribution of the loop of Henle, the vasa recta, and urea cycling in the creation and maintenance of this gradient, along with the impact of ADH. More detailed explanation of each contribution was required as overarching statements were not sufficient to attract all marks for each section. Answers that focused solely on the counter-current exchange and multiplier process were insufficient on their own to achieve a passing mark. The examiners commented that a significant proportion of candidates excluded the role of urea in their answers.
2020A 04: 63% of candidates passed this question.
Higher scoring candidates described the counter-current multiplier mechanism, the countercurrent exchanger and the contribution of urea cycling to the medullary osmotic gradient. Detailing the mechanisms as to how they may be established, maintained and or regulated. Descriptions of the multiplier (LOH) alone did not constitute a passing score. Values for osmolality at the cortex & medulla and within the different parts of the LOH was required.
A description of the countercurrent exchanger system where inflow runs parallel to, counter to and in close proximity to the outflow was expected. This could have been achieved by describing the anatomical layout of the loop of Henle and the vasa recta.
2015B 22: 48% of candidates passed this question.
Most answers gave a good account of counter currents in the kidney but few described the generation of the counter current multiplier arrangement. Many candidates did not discuss counter current mechanisms in the skin but instead, focussed on thermal control by the skin.
2011A 09: 4 (33%) of candidates passed this question.
A useful introduction could include a definition of medullary concentration gradient and its function. The answer was expected to describe the roles of sodium, chloride and urea in the countercurrent multiplier and the features of the vasa recta countercurrent exchange system.
The 3 main areas that needed to be addressed to pass this question included:
[1] The loops of Henle with their water permeable descending limbs and water impermeable ascending limbs, which actively remove solutes from the tubular lumen. The counter current multiplier system.
[2] The vasa recta which run parallel to the loops of Henle and are permeable to water and solute and have low flow. This allows the medullary concentration gradient to be maintained. The counter current exchange mechanism.
[3] The role of urea which is concentrated in the medulla by mechanisms which involve changes in permeability to urea in different regions of the tubules partly influenced by the effects of antidiuretic hormone.
Some candidates elected to draw the loop of Henle and vasa recta together with the movement of various solutes and water and answer the question from it.
Unfortunately mistakes in these diagrams only confused their answers further.
Syllabus: Section D1, 2c
Recommended sources: Principles of Physiology for the Anaesthetist, Power and Kam, page 234
v. Describe the functions of the kidney.
2013A 09
Outline the functions of the kidney.
CICMWrecks Answer
- Excretion of metabolic waste and foreign substances
- Filtration of metabolic waste products
- urea from protein
- uric acid from nucleic acids
- creatinine from muscle creatine
- Filtration of drugs
- Filtration of metabolic waste products
- Regulation of water and electrolyte balance
- Homeostatic mechanism
- 180L of fluid and other substances per day, with 99% reabsorbed along the tubule
- Concentration of urine via the countercurrent mechanism
- max 1400mOsm to reduce renal water loss
- Reabsorb K, Ca, Mg and Cl either passively or actively
- Regulation of plasma osmolality
- Plasma osmolality is altered whenever the inputs and outputs of water and solutes changes disproportionately.
- Input from the hypothalamic osmoreceptors contributes.
- Regulation of acid base balance
- Excretion of H+ ions, fixed acids, phosphate and ammonium
- Resorption of HCO3- to maintain plasma neutrality
- Regulation of blood pressure
- Production of angiotension 2 via the RAAS in response to a reduction in GFR system
- peripheral vasoconstriction
- reabsorption of sodium
- secretion of aldosterone
- → rise in MAP
- Changes in blood volume regulated by the kidney (as mentioned above) will also influence BP
- Production of angiotension 2 via the RAAS in response to a reduction in GFR system
- Endocrine function
- Vitamin D
- EPO to stimulate RBC production (in response to a reduction in pO2 the secretory cells which lie in between the cortex and medulla)
- Aldosterone and angiotensin II as regulated by the RAAS will contribute to BP management
- Prostaglandins esp PGE2 and prostacyclin – potent renal vasodilators
- Bradykinin – potent renal vasodilator
- Gluconeogenesis
- The renal cortex contains glucose-6-phosphatase and thus the ability to release glucose in times of need
Gladwin 2016
Examiner Comments
Outline type questions require a comprehensive list and a brief explanation of each major function of the kidney. This should have included water balance, electrolyte balance, endocrine function, filtration, metabolism, acid-base balance, excretion and blood pressure control.
Adequate breadth was lacking in many answers. Marks were awarded for discussion of water reabsorption at specific points along the length of the nephron and the control mechanism through Aquaporins and ADH. It was expected answers would include calcium, magnesium, glucose and amino acid handling by the kidney (as well as sodium and potassium). Better answers discussed the elimination of fixed as well as volatile acids and outlined the role of ammonia. Discussion of the endocrine functions would include EPO, ,25 dihydroxy-cholecalciferol, prostaglandin E, renin, angiotensin II and kallekrein.
Superficial responses such as, “the kidney is important in regulating volume” (or “waste” or “water” or “sodium”) were not sufficient.
2017A 04
Describe the endocrine functions of the kidney.
CICMWrecks Answer
Non-RAAS Endocrine Functions
Vitamin D
Vitamin D has a complex metabolic pathway which meanders through a number of organ systems:
- Vitamin D2 (ergocalciferol) only in diet
- Vitamin D3 (cholecalciferol) absorbed in diet or produced in skin by the action of UV light
- 7-dehydrocholesterol à 7-dehydroxycholecalciferol à cholecalciferol
- Liver: Cholecalciferol hydrolysed à 25-hydroxycholecalciferol (25-OHD3)
- Enzyme: Vitamin D25-hydroxylase (CYP450 enzyme)
- Released into plasma, binds to Vitamin D-binding protein (a globulin carrier protein)
- Kidney: 25-OHD3 hydrolysed à 1,25-dihydroxycholecalciferol (calcitriol – active form)
- in the proximal tubule, Enzyme: 1alpha hydroxylase
- Released into circulation
- Transported by Vitamin D binding enzyme to intestine, kidney, bone
Erythropoietin
Secretion of EPO to stimulate RBC production.
Erythropoiesis is stimulated by EPO release:
- In adults, EPO is released from the:
- Peritubular capillary fibroblasts (85%)
- Liver (15%)
- EPO is released in response to:
- Hypoxia (near the secretory cells which lie in between the cortex and medulla)
- Hypotension
- Low Hct
- Erythropoiesis is inhibited by:
- High red cell volume
Production of EPO is decreased in renal failure, which is why patients with end-stage renal disease require exogenous EPO.
Prostaglandins:
Production of prostaglandins esp PGE2 and prostacyclin – potent renal vasodilators
Bradykinin:
Production of bradykinin – potent renal vasodilator
RAAS
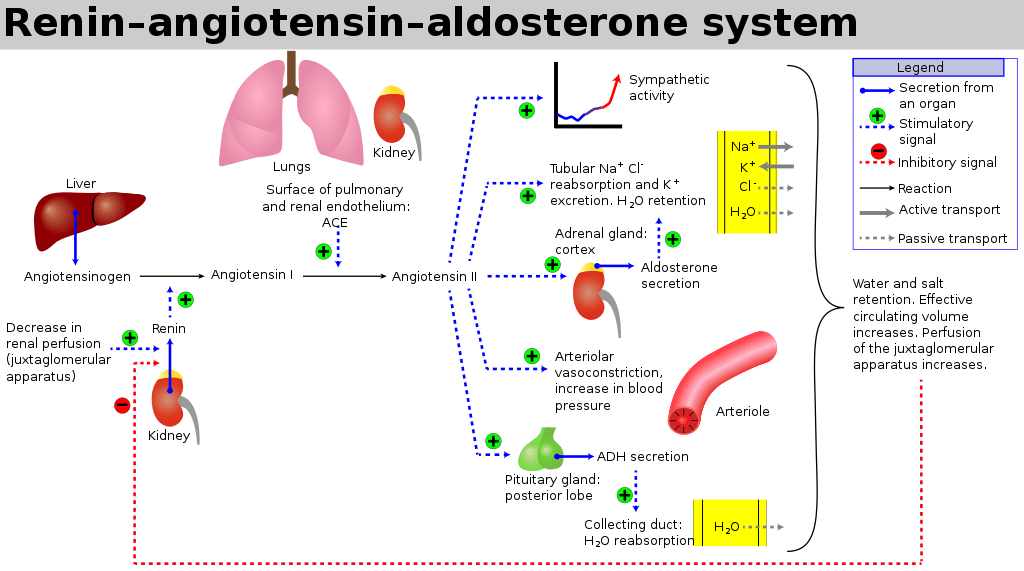
The RAAS is a signaling pathway involved in blood pressure control. It involves a number of hormones:
- Angiotensinogen is produced by the liver
- Renin is a protease enzyme (Half-life 80mins)
- produced by the kidneys
- stored by the granular cells of the juxtaglomerular apparatus, which lies close to the glomerulus and distal tubule
- Secretion is stimulated by:
- Pressure changes in the afferent arteriole
- Stimulation of the macula densa when changes occur in tubular NaCl concentration
- Renal sympathetic nerve activity (a direct β1 effect)
- Baroreceptor reflexes
- Negative feedback from AT II
- Exists to cleave hepatic angiotensinogen to the 10-AA peptide angiotensin I
- Note: Renin is rate-limiting step for activation of RAAS
- Angiotensin I
- has mild vasoconstrictor properties, but not enough to cause significant changes in circulation
- Angiotensin Converting Enzyme (ACE)
- Found mainly in pulmonary vascular endothelial cells – converts angiotensin I to angiotensin II
- Also cleaves bradykinin into inactive metabolites
- Angiotensin II
- Effects include a general reduction in sodium and water excretion, and maintenance of circulating blood volume, GFR and blood pressure.
- Rapidly removed from circulation in 1-2mins, presumably by tissue peptidases (Angiotensinases)
- Aldosterone
- is secreted from the zona glomerulosa of the adrenal cortex
- It acts in the renal CCD to enhance the activity of ENaC and K+ secretion by principal cells.
- increases Na+ (and water) reabsorption in exchange for K+ (kidney, gut, salivary, sweat)
JC 2019
Examiner Comments
2017A 04: 39% of candidates passed this question.
It was expected that candidates would discuss the major hormones produced (or activated) by the kidney. These included erythropoeitin, renin and calcitriol. Good answers included the following: the area where the hormone is produced or modified; stimuli for release; factors which inhibit release; and the subsequent actions / effects. Marks were not awarded for hormones that act on the kidney.
2021B 05
Write detailed notes on angiotensin, including its synthesis, role within the body and regulation.
2009B 18
Outline the physiology of, and factors which regulate levels of angiotensin.
CICMWrecks Answer
Angiotensinogen is a peptide hormone produced by the liver
- Angiotensin 1 (decapeptide)
- ↑ Renin by JGA → cleavage of Angiotensinogen → AT1
- No biological activity
- Angiotensin 2 (octapeptided)
- ACE in pulmonary capil endothelia
- → ↑AT2
- Angiotensin 3 (septapeptide)
- 40% of the pressor activity AT2
- 100% of the aldosterone-producing activity
- Angiotensin 4 (hexapeptide)
- Minimal biological activity
Actions of AT2 include:
- Acts via GPCR (Gq via PLC to increase IP3 and intracellular Ca)
- CVS
- Vasopressor
- Resets baroreceptor control of HR at higher pressure
- Potent mitogen for smooth muscle and cardiac myocytes
- Direct positive inotrope
- CNS
- ↑ sympathetic outflow
- ↑ thirst
- ↑ ADH release from posterior pituitary.
- Renal
- Negative feedback on renin release
- ↑ aldosterone release
- ↓ RBF and GFR
- Direct renal arteriole constriction (efferent = afferent)
- Mesangial cell contraction thus ↓ Kf and GFR
- ↑ sodium/chloride reabsorption in PCT
- Direct effect and via ↑ aldosterone release
Factors which alter renin release cause a corresponding change in Angiotensin 2
- Stimulation of renin secretion (↑ renin → ↑ angiotensin 2)
- β-1 agonism
- ↓ renal perfusion pressure
- ↓ sodium delivery to DCT
- Prostaglandins
- Inhibition or renin secretion (↓ renin → ↓ angiotensin 2):
- ↑ renal perfusion pressure (via afferent arteriolar dilatation)
- ↑ sodium delivery to DCT
- ↑ by angiotensin II (negative feedback) and vasopressin
Gladwin 2016
Examiner Comments
2021B 05: 24% of candidates passed this question.
This question provided headings for the answer template. Good answers integrated the required facts from the appropriate chapters of the major texts. Most answers lacked detail surrounding the factors that increase or decrease angiotensin activity. Few answers provided any detail as to all the mechanisms through which angiotensin exerts it effects. A lot of answers focussed singularly on the vascular effects of angiotensin. Overall, there was often a paucity of detail, with vague statements and incorrect facts.
2009B 18: 4 (44%) of candidates passed this question.
For a good answer it was expected that candidates would mention the relationship of angiotensinogen, angiotensin I, renin and Angiotensin Converting Enzyme to angiotensin II production, the actions and fate of angiotensin II and factors that regulate angiotensin II. It was expected that candidates mention angiotensin acts as a potent vasoconstrictor, stimulates aldosterone secretion, facilitates noradrenaline release, preferentially vasoconstricts Efferent arteriole in nephron, preserving GFR in low perfusion states, increases renal tubular Na + reabsorption and increases secretion of vasopressin and ACTH.
Regulation of Angiotensin could be separated into factors increasing angiotensin levels (eg prostaglandins, low K+ levels, Sympathetic stimulation, ↓ Na+ delivery at distal tubule, any factor contributing to reduced renal blood flow (eg hyovolaemia, cardiac failure, renal artery stenosis) and factors reducing angiotensin levels (eg hypervolaemia, afferent arteriolar dilatation and vasopressin).
Syllabus – N1 (h), C2b (f)
Reference: Textbook of medical Physiology, Guyton, Chp 26, Goodman and Gillman, Chp 30
2011B 06
Describe the physiology of the Renin and Angiotensin system.
CICMWrecks Answer
Renin-Angiotensin-Aldosterone system primarily for hormonal regulation of blood volume.
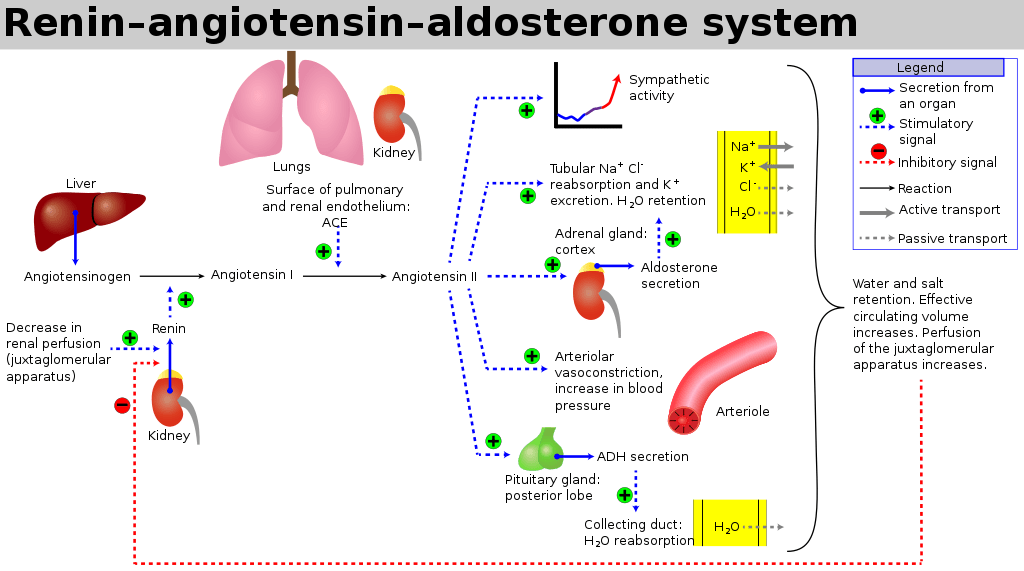
Renin
- Proteolytic enzyme
- Secreted from granula cells in juxtaglomerular apparatus
- Secretion:
- Stimulated by:
- ↓ GFR
- ↓ tubular Na/Cl at macula densa
- β1 SNS stimulation of granular cells
- ↑ prostacyclin levels
- ↓ levels of angiotensin 2 or ADH
- Inhibited by:
- ↑ renal perfusion pressure
- ↑ sodium delivery to DCT
- ↑ by ATII and ADH
- Stimulated by:
- Target:
- circulating angiotensinogen
- Effects:
- Tubular effects:
- ↑’d PCT Na/Cl reabsorption, ↑’d tubular K secretion
- Direct PCT effect
- Aldosterone release
- Volume Status effects:
- ↑’d total body water via
- ↑’d thirst (hypothal)
- ↑’d ADH secretion
- Renal Blood flow effects
- Direct: Reduced RBF and GFR (Aff>Eff)
- ↑’d renin → ↑ AT2 (see AT2 below) → ↑’d Aldosterone
- Vasoconstriction (aff=eff)
- Mesangial cell contraction
- Tubular effects:
Angiotensin 2
- Production:
- Angiotensinogen is the precursor peptide hormone produced by the liver
- Angiotensin 1 (decapeptide)
- ↑ Renin by JGA (above) → cleavage of Angiotensinogen → AT1
- No biological activity
- Angiotensin 2 (octapeptided)
- Angiotensin Converting Enzyme (ACE) in pulmonary capil endothelia
- → ↑AT2
- Angiotensin 3 (septapeptide)
- 40% of the pressor activity AT2
- 100% of the aldosterone-producing activity
- Angiotensin 4 (hexapeptide)
- Minimal biological activity
- Target:
- Mesangial cells, arteriolar SM, CNS, posterior pituitary
- AT1 and AT2 receptors
- Acts via GPCR (Gq via PLC to increase IP3 and intracellular Ca)
- Effect:
- CVS
- Vasopressor
- Resets baroreceptor control of HR at higher pressure
- Potent mitogen for smooth muscle and cardiac myocytes
- Direct positive inotrope
- CNS
- ↑’d sympathetic outflow
- ↑’d thirst
- ↑’d ADH release from posterior pituitary.
- Renal
- Negative feedback on renin release
- ↑’d aldosterone release
- ↓’d RBF and GFR
- Direct renal arteriole constriction (efferent = afferent)
- Mesangial cell contraction thus ↓’d Kf and GFR
- ↑’d sodium/chloride reabsorption in PCT
- Direct effect and via ↑’d aldosterone release
- CVS
Aldosterone
- Steroid Hormone
- Source:
- Adrenal cortex (zona glomerulosa)
- Release Stimuli:
- adrenal cortical hyperkalaemia
- ↑’d AT2
- ↑’d ACTH
- Target:
- Distal collecting duct and CCD principal cells ENaC receptors.
- Na/K ATPase co-transporters in DCT/CCD, GIT and secretory glands
- Effect:
- Sodium (↑’d plasma Na)
- Upregulates basolateral ENaC and Na/K ATPase to increase Na reabsoption
- ↑’d Na reabsorption throughout the GIT/sweat and salivary glands via Na/K ATPase
- ↑’d H2O reabsorption and ↑’d Na via solvent drag
- Potassium (↓’d plasma K)
- ↑’d K secreation at DCT/CCD principal cells (via ROMK)
- ↑’d K secretion throughtout the GIT/Salivary glands
- ↑’d intracellular K uptake
- Chloride (↑’d plasma Cl)
- ↑’d Cl uptake in distal tubule
- Acid (H) Effects
- Upregulation of H and H/K-ATPase in type A intercalated cells of CCD
- Increases H secretion (and HCO3 reabsorption)
- Sodium (↑’d plasma Na)
Gladwin 2016
Examiner Comments
2011B 06: 15 (60%) of candidates passed this question.
Renin and angiotensin are core components in the regulation of plasma volume and blood pressure regulation. It is unfortunate that many candidates presenting to this examination are not able to provide sufficient information for a pass. For a good answer, candidates were expected to mention what renin and angiotensin are and what they do, as well as briefly mention the place of angiotensin converting enzyme (converts Angiotensin I to Angiotensin II and inactivates bradykinin). Renin is a proteolytic enzyme cleaves angiotensinogen to angiotensin I, secreted by the juxtaglomerular cells of the kidney which are located in media of afferent arteriole and in close proximity to the glomerulus and the distal convoluted tubule (macula densa). Angiotensin II acts on cell surface AT1 and AT2 receptors. Major functions being to preserve of GFR & enhanced Na/H2O reabsorption in the setting of reduced renal blood flow (candidates expected to outline the mechanism by which this occurs), vasoconstriction, stimulate aldosterone secretion and increase thirst and ADH secretion. The better candidates also mentioned that it decreases sensitivity of baroreceptor reflex, increases secretion of ACTH and facilitates noradrenaline release from sympathetic nervous system as well as its fate (metabolized by blood/tissue peptidases). A good response for regulation would have been mentioning principally regulated via renin release (which in itself is influenced by renal sympathetic nervous system activity, intrarenal baroreceptors and macula densa sodium chloride delivery, ADH and intra-renal prostaglandins), negative feedback from angiotensin II.
Syllabus: N2h
Recommended sources: Textbook of medical Physiology, Guyton, Chp 26.
vi. Describe the physiological effects of renal dysfunction.
2012B 19
Describe the changes that occur in the plasma with renal dysfunction.
CICMWrecks Answer
Water
- Hypervolaemia may occur if oliguric renal failure
Electrolytes
- Renal failure can retard the kidneys ability to eliminate excess potassium
- Hyperkalaemia
- Sodium relatively preserved
- Acid-Base balance
- Approx. 80mEq/day of non-volatile acids require elimination daily
- Renal failure leads to progressive acidosis due to loss of buffering ability and limiting urinary pH of 4.5
- Loss of renal compensation of respiratory acid/base disturbances
- Approx. 80mEq/day of non-volatile acids require elimination daily
- Renal conversion of 25-hydroxy Vit.D to 1,25,-dihydroxy Vit D promotes GI reabsorption of Ca à lost in renal dysfunction leading to hypocalcaemia and hypophosphataemia
Proteins
- Damage to the glomerular basement membrane (Goodpasture’s Disease, other causes of nephrotic syndrome) can lead to renal elimination of protein and loss of plasma proteins due to increased glomerular filtration
- The kidney’s eliminate nitrogenous waste products of protein metabolism (urea), and uricaemia occurs in renal failure
Other
- Creatinine
- Metabolite of creatine phosphate, generated at constant rate, and eliminated renally. Increases in renal failure
- Only after 75% loss of functional nephrons
- Decreased production of Erythropoietin
- Parathyroid hormone increased due to hypocalcaemia
Sakurai 2016
Examiner Comments
2012B 19: 9 (41%) of candidates passed.
A good answer required an integrated knowledge of various aspects of basic physiology. Most often there was a lack of breadth and/or depth of knowledge (e.g. mention that plasma creatinine increases, but failure to mention that it only increases after substantial (>75%) loss of nephron function). It was expected that some mention of changes in electrolytes (e.g. Na+, K+, Ca2+), HCO3, PO4, hormones (1, 25 vitamin D, erythropoietin), proteins, etc. be included.
H2. Applied Renal Physiology
i. Describe the principles of dialysis and filtration.
2011A 24
Describe the PHYSICAL PRINCIPLES that are involved in the flow of blood through a dialysis circuit, and, in the movement of solutes across a dialysis membrane.
CICMWrecks Answer
Dialysis
- “process whereby the composition of a solution (patient’s blood) is altered by exposure to a second solution (dialysate) through a semi-permeable membrane”
- involves transfer of H2O and LMWT solutes across the membrane between the solutions via diffusion, osmosis and solvent drag
- Indications:
Failure of normal renal functions, i.e.:- Acid-base imbalance
- Electrolyte derangement – Particularly hyperkalaemia.
- Intoxications
- Overload
- Ureamia
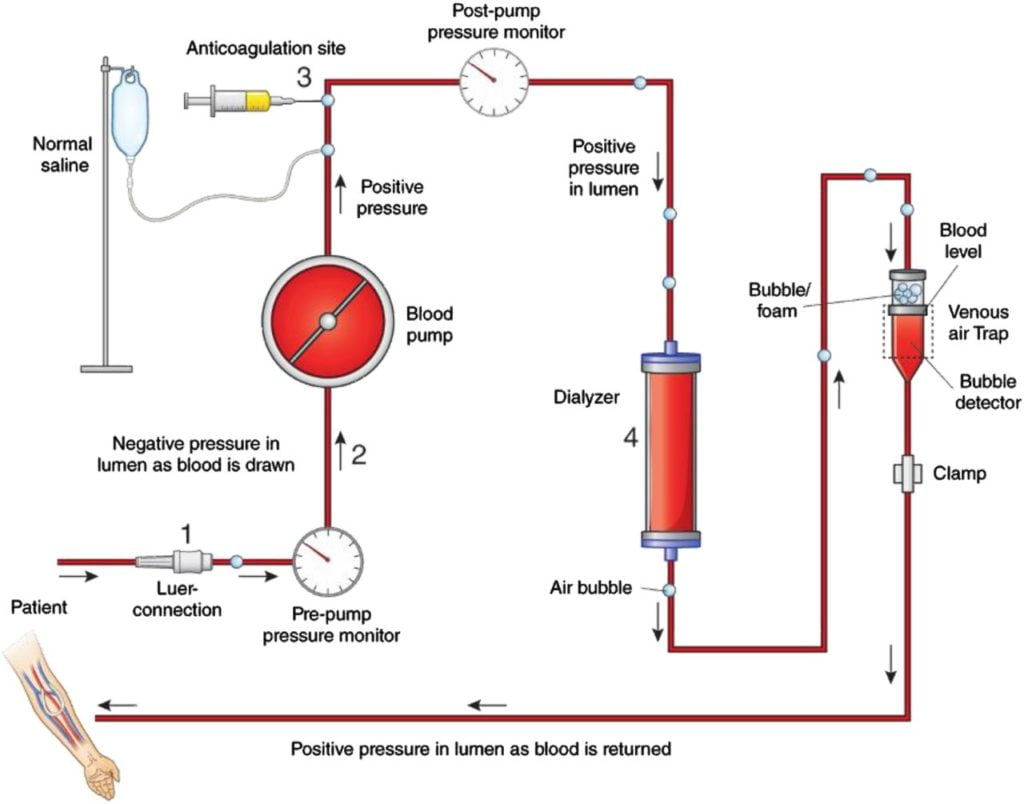
Physical principles related to blood flow:
where, Q = Flow
ΔP = pressure gradient
r = radius
η = Viscosity
l = length
- In a dialysis circuit, blood travels from the patient to a dialysis machine where it passes through a dialyser, allowing movement of fluid and plasma solutes across the membrane in either direction.
- Flow across the circuit is determined by:
- Set flow rate of centrifugal dialysis pump
- parts of the circuit:
- access port of vascular access (catheter / fistula / graft)
- from patient (pre-membrane)
- across membrane (trans-membrane)
- to patient (post-membrane)
- return port of vascular access (catheter / fistula / graft)
- Assuming that machine delivers the flow which is set and the flow remains laminar and constant, the pressure across the various components varies based on the resistance.
- The pressure gradient across the circuit depends upon:
- characteristics of patient:
- Blood viscosity
- hematocrit
- Blood protein and lipid content
- Temperature changes changes flow and viscosity
- Fåhræus–Lindqvist effect: Viscosity reduces in smaller diameter – because erythrocytes move over to the centre of the vessel/circuit, leaving only plasma near the wall of the vessel.
- variable resistance during breathing or movement
- collapsing vessels due to decreased vessel size / hypovolemia
- antivoagulation used
- Blood viscosity
- characteristics of vascular access:
- gauge and length of access
- resistance from physical obstruction
- resistance position
- resistance from clots, fibrin
- characetristics of circuit:
- Age of circuit, presence of clots, fibrin
- radius
- length (usually constant ~3.5m)
- surface coating of circuit
- anticoagulation used
- characteristics of membrane:
- resistance worsens with clot, fibrin, lipid deposition
- characteristics of patient:
- In some cases, resistance increases significantly, flow changes to turbulent, and dialysis machine may not be able to acheive set blood flow.
(This usually activates some sort of alarm and will require quick troubleshooting) - As flow is set, the pressure across the various components varies based on the resistance
Physical Mechanisms of fluid and solute removal
- Fluid removal Mechanisms:
- Osmosis:
- the movement of a pure solvent such as water, through a differentially permeable membrane, from a solution that has a lower solute (particle) concentration to one that has a higher solute concentration
- The rate of osmosis depends on the concentration of solute, the temperature of the solution, the electrical charge of the solute and the difference between the osmotic pressures exerted by the solutions.
- Movement across the membrane continues until the concentrations of the solutions equalise.
- Ultrafiltration
- the movement of fluid through a membrane caused by a pressure gradient (hydrostatic or osmotic pressure)
- Uses both positive and negative pressure:
- Positive pressure: pressure exerted by the blood flowing through the dialyzer, Results from blood being pushed by blood pump
- Negative pressure: pressure applied to the dialysate side by the machine
- Pulls excess fluid from blood compartment to dialysate compartment
- Solute removal mechanisms:
- Diffusion
- the movement of solutes from a high to a low solute concentration across a semipermeable membrane
- a concentration gradient is necessary for diffusion to occur
- it removes all small molecules
- the rate of diffusion is dependent on
- surface area of filter
- ratio of dialysate flow to blood flow
- size of the molecules
- Convection:
- The movement of solutes with a water-flow “solvent drag” across a membrane during osmosis or ultrafiltration
- Important for movement of small solute (urea, creatinine).
- Adsorption:
- when the molecules (solutes) adhere to the surface or interior of the membrane.
- with the movement of fluid across the membrane, if no fluid is moving then adsorption can not occur.
- in 2 manners:
- surface adsorption where the molecules are too large to permeate and migrate through the membrane; however they can adhere to the membrane.
- bulk adsorption occurs within the whole membrane where molecules can permeate it.
- Molecules that can be effectively adsorbed include:
- B2 microglobulin
- Cytokines
- Coagulation factors
- Anaphylatoxins
- The rate of movement of solute across the dialyzing membrane depends on
- the concentration gradient of the solute between the two solutions,
- the permeability of the membrane to the solute,
- the surface area of the membrane, and
- the length of time that the blood and fluid remain in contact with the membrane
- Concentration gradient:
- The concentration gradient is developed by using dialysate fluid with low concentration of solutes that are usually cleared by the kidney (eg; Na, K, PO4, urea, creatinine), in order to create a steep concentration gradient to encourage movement of these solutes from the blood to the dialysate.
- The movement of blood and dialysate in different directions ensures this concentration gradient does not reach equilibrium and reduce further solute exchange.
JC 2019
Examiner Comments
2011A 24: 2 (17%) of candidates passed this question.
This question required candidates to describe the physical principles of blood flow through a dialysis circuit and the movement of solute across a dialysis membrane. While most candidates were able to allude to important factors contributing to the flow of a fluid through a hollow tube, few did so in a systematic way and only some provided relevant formulae showing the relationship between pressure, fluid viscosity and tube resistance. A short discussion proceeding to flesh out the factors that determine blood viscosity, circuit pressures and practical examples was expected.
Some candidates discussed convective processes extensively, which was not required in this question focussed on dialysis. Most candidates were able to describe the physical chemistry involved in diffusion across a semipermeable membrane in basic terms, however few provided sufficient details of these important principles.
Very few candidates went on to properly discuss electrochemical forces affecting solute and water movement across a membrane or the factors that influence the performance of dialytic therapies in practical application.
Syllabus: A2c, R2e, D1, 2b,c
Recommended sources: Basic Physics and Measurement in anaesthesia, Davis and Kenny, various sections. Also Review of Medical Physiology, Ganong, chp 2, 32
2021B 20
Describe the physical principles of haemodialysis and haemofiltration, including the factors affecting clearance (80% marks).
Outline the key components of renal replacement fluids (20% marks).
CICMWrecks Answer
Physical Principles and factors affecting clearance
- Haemodialysis:
- Uses diffusion: “the movement of solutes from a high to a low solute concentration across a semipermeable membrane”
- Convection (see below) occurs during diffusion
- Blood is pumped through an extracorporeal circuit that contains a dialyser
- Dialysate flow is countercurrent, with maximizes the gradient for diffusion
- Solutes move across a membrane between blood and dialysate as per Fick’s Law
- Flow across the circuit is determined by:
- Set flow rate of centrifugal dialysis pump
- parts of the circuit:
- access port of vascular access (catheter / fistula / graft)
- from patient (pre-membrane)
- across membrane (trans-membrane)
- to patient (post-membrane)
- return port of vascular access (catheter / fistula / graft)
- Assuming that machine delivers the flow which is set and the flow remains laminar and constant, the pressure across the various components varies based on the resistance.
- The pressure gradient across the circuit depends upon:
- characteristics of patient:
- Blood viscosity
- hematocrit
- Blood protein and lipid content
- Temperature changes changes flow and viscosity
- Fåhræus–Lindqvist effect: Viscosity reduces in smaller diameter – because erythrocytes move over to the centre of the vessel/circuit, leaving only plasma near the wall of the vessel.
- variable resistance during breathing or movement
- collapsing vessels due to decreased vessel size / hypovolemia
- antivoagulation used
- Blood viscosity
- characteristics of vascular access:
- gauge and length of access
- resistance from physical obstruction
- resistance position
- resistance from clots, fibrin
- characetristics of circuit:
- Age of circuit, presence of clots, fibrin
- radius
- length (usually constant ~3.5m)
- surface coating of circuit
- anticoagulation used
- characteristics of membrane:
- resistance worsens with clot, fibrin, lipid deposition
- characteristics of patient:
- Uses diffusion: “the movement of solutes from a high to a low solute concentration across a semipermeable membrane”
- Haemofiltration:
- Uses ultrafiltration: “the movement of fluid through a membrane caused by a pressure gradient (hydrostatic or osmotic pressure)”
- Convection (see below) occurs during ultrafiltration
- Positive hydrostatic pressure in blood and a negative hydrostatic pressure in dialysate is generated → causing ultrafiltration and removal of solutes via solvent drag
- Elimination via bulk flow is independent of solute concentration gradients across the membrane
- Transport is dependent on starling forces
- The transmembrane pressure generated: blood flow to the membrane; oncotic pressure gradient
- Porosity of the membrane
- Additionally, a high filtration fraction will cause excessive haemoconcentration, and clotting of the filter
- The filtered fluid (ultrafiltrate) is discarded, and replaced with another fluid depending on the desired fluid balance
- Uses ultrafiltration: “the movement of fluid through a membrane caused by a pressure gradient (hydrostatic or osmotic pressure)”
Other Mechanisms involved:
- Osmosis:
- the movement of a pure solvent such as water, through a differentially permeable membrane, from a solution that has a lower solute (particle) concentration to one that has a higher solute concentration
- The rate of osmosis depends on the concentration of solute, the temperature of the solution, the electrical charge of the solute and the difference between the osmotic pressures exerted by the solutions.
- Movement across the membrane continues until the concentrations of the solutions equalise.
- Convection:
- The movement of solutes with a water-flow “solvent drag” across a membrane during osmosis or ultrafiltration
- Important for movement of small solute (urea, creatinine).
- Adsorption:
- when the molecules (solutes) adhere to the surface or interior of the membrane.
- with the movement of fluid across the membrane, if no fluid is moving then adsorption can not occur.
- in 2 manners:
- surface adsorption where the molecules are too large to permeate and migrate through the membrane; however they can adhere to the membrane.
- bulk adsorption occurs within the whole membrane where molecules can permeate it.
- Molecules that can be effectively adsorbed include:
- B2 microglobulin
- Cytokines
- Coagulation factors
- Anaphylatoxins
Renal Replacement Fluids
- Typically pre-packaged in 5L bags of sterile water
- contain sterile water, electrolytes and buffer
- Vary slightly in composition, but all are balanced salt solutions with either a lactate, bicarbonate or citrate buffer
- Bicarbonate-based solutions: most commonly used two-compartment systems. 25-35mmol/L and used as both dialysate and replacement fluids
- Lactate-based solutions: stable, cheaper, practical. But buffering capacity depends on conversion of lactate to bicarbonate. So not commonly used in CRRT
- Citrate-based solutions: new generation, administered prefilter. Advantage that additional anticoagulation is not required.
- Calcium-free solutions: When citrate-based fluids are used prefilter, these are used as dialysate and replacement fluids
- At present, there is no evidence to suggest that the choice of replacement fluid has an impact on survival or renal recovery.
- Replacement fluid can be added pre- or postfilter in varying ratios.
- The benefit of adding some of the replacement fluid prefilter is that it lowers the hematocrit of the blood, which reduces the likelihood of the filter clotting.
- The downside is that predilution reduces solute clearance and a compensatory increase in flow rates should be considered (15% for ultrafiltration rates of 2 L/h and up to 40% for rates of 4.5 L/h).
Composition (mmol/L) | PrismOcitrate 18/0 (Baxter) | PrismOcal (Baxter) | PrismOcal B22 (Baxter) | Prismasol (Baxter) | Hemosol B0 (Baxter) |
---|---|---|---|---|---|
Sodium | 140 | 140 | 140 | 140 | 140 |
Potassium | 0 | 0 | 4 | 0 | 0 |
Calcium | 0 | 0 | 0 | 1.75 | 1.75 |
Magnesium | 0 | 0.5 | 0.75 | 1 | 0.5 |
Chloride | 86 | 106 | 120 | 110 | 109.5 |
Bicarbonate | 0 | 32 | 22 | 32 | 32 |
Phosphate | 0 | 0 | 0 | 0 | 0 |
Citrate | 18 (=54mmol bicarb post metabolism) | – | – | – | – |
Lactate | 0 | 3 | 3 | – | 3 |
Osmolality (mOsmol/L) | 244 (280 post metabolism) | 282 | 282 | 287 | 287 |
Source: Mishra, Rajesh Chandra. ISCCM Manual Of RRT And ECMO In ICU A Reference Book For Practicing Intensivists (p. 25). Jaypee Brothers Medical Publishers.
Kerr’s notes
JC / Kerr 2022
Examiner Comments
2021B 20: 28% of candidates passed this question.
A brief description of the underlying mechanisms of dialysis and hemofiltration was required. Diffusion, the predominant mechanism in haemodialysis, involves movement of solute down the concentration gradient across the semipermeable membrane. This concentration gradient is generated and maintained by counter current movement of dialysate and blood. In hemofiltration the predominant mechanism is convection and solvent drag of the solute across the semipermeable membrane by application of transmembrane pressure. The filtrate is then replaced by replacement fluid. Small molecules are effectively removed by dialysis whereas hemofiltration can remove small and middle molecules. Various factors that impact clearance in haemodialysis and haemofiltration were expected separately.
Constituents of replacement fluid should have included three broad headings of electrolytes, buffer and sterile water. Many answers lacked the details of how counter current mechanisms help, the difference in the two modalities in regard to clearance of molecules, how clearance is impacted by protein binding and volume distribution, sieving coefficient of the membrane and flow rates of blood and dialysate (or effluent) flow. The constituents of replacement fluid lacked details of various types of electrolytes, the common buffers and the strong ion difference.
H3. Renal Pharmacology
i. Understand the pharmacology of diuretics.
2007B 02
Outline the sites and mechanisms of actions of diuretics. Give one example of each class and two side effects.
CICMWrecks Answer
Sites and Mechanisms of actions of diuretics
Location | Drug | Mechanism of Action | Adverse Effects |
---|---|---|---|
PCT | Acetazolamide | Prevents the intracellular conversion of CO2 and H2O to H2CO3 → Resulting HCO3 usually reabsorbed via Na/HCO3 co transporter therefore decreased HCO3 leads to decreased Na reabsorption → decreased reabsorption of water | Metabolic acidosis Renal stones Hyponatraemia |
LoH | Frusemide | Inhibits Na/K/2Cl transporter on luminal membrane preventing ion reabsorption → increases osmolality of luminal fluid → decreased osmotic gradient for water reabsorption | Hypochloraemic metabolic alkalosis Hypernatraemia Hypokalaemia Hypomagnesaemia |
DCT | Thiazide | Inhibits Na/Cl transporter on the luminal membrane preventing Na and Cl reabsorption → increases osmolality in luminal fluid → decreased osmotic gradient for water reabsorption | Hypochloraemic metabolic alkalosis Hyponatraemia Hypokalaemia Dyslipidaemia Hyperglycaemia |
CD | Spironolactone | Prevents activity of aldosterone → Decreased ENaC channels for Na reabsorption in the collecting duct and decreased Na/K ATPase activity in collecting duct → Prevents Na reabsorption and consequently reduced osmosis | Gynaecomastia Hyperkalaemia Renal stones |
Other | Mannitol | Increases osmolality of of luminal fluid → decreases osmotic gradient for H2O reabsorption | Hypotension Tachycardia Thrombophlebitis Convulsions |
CICMWrecks 2019
Further Reading:
Examiner Comments
2007B 02: 4 candidates (57%) passed this question.
Good answers to this question were those that had a tabular format to the structure of the answer – for example column headed mechanism, sites, drug and side effects. Most common omissions were not to further describe how the different mechanisms of action of diuretics increased urine output, e.g. “disruption of the counter current multiplier system by decreasing absorption of ions from the loop of Henle into the medullary interstitium, thereby decreasing the osmolarity of the medullary interstitial fluid”. There was ofter little mention of increased urine solutes and the effect the electro chemical effect had in promoting a diuresis. Examples of drugs were well done.
2022B 17
Describe the site of action, mechanism of action and the biochemical effects of five different classes of diuretics; include an example in each class.
CICMWrecks Answer
Sites and Mechanisms of actions of diuretics
Class | Drug | Sites | Mechanism of Action | Biochemical effects |
---|---|---|---|---|
Carbonic Anhydrase Inhibitor | Acetazolamide | PCT | Prevents the intracellular conversion of CO2 and H2O to H2CO3 → Resulting HCO3 usually reabsorbed via Na/HCO3 co transporter therefore decreased HCO3 leads to decreased Na reabsorption → decreased reabsorption of water | Metabolic acidosis Hyponatraemia Hypokalemia Hyperchloremia |
Loop | Frusemide | LoH | Inhibits Na/K/2Cl transporter on luminal membrane preventing ion reabsorption → increases osmolality of luminal fluid → decreased osmotic gradient for water reabsorption | Hypochloraemic metabolic alkalosis Hypernatraemia Hypokalaemia Hypomagnesaemia Hypophosphatemia Acidification of urine |
Thiazide | Hydrochlorothiazide | DCT | Inhibits Na/Cl transporter on the luminal membrane preventing Na and Cl reabsorption → increases osmolality in luminal fluid → decreased osmotic gradient for water reabsorption | Hypochloraemic metabolic alkalosis Hyponatraemia Hypokalaemia Dyslipidaemia Hyperglycaemia |
Aldosterone Antagonist | Spironolactone | CD | Prevents activity of aldosterone → Decreased ENaC channels for Na reabsorption in the collecting duct and decreased Na/K ATPase activity in collecting duct → Prevents Na reabsorption and consequently reduced osmosis | Hyponatremia Hyperkalaemia Metabolic acidosis |
Osmotic | Mannitol | Other | Increases osmolality of luminal fluid → decreases osmotic gradient for H2O reabsorption | Hypokalemia Hyponatremia Hypocalcemia |
CICMWrecks 2019
Further Reading:
Examiner Comments
2022B 17: 90% of candidates passed this question.
This question was best structured using the headings supplied in the stem (site, mechanism of action, biochemical effects and an example). Some answers utilised a table format which often meant that the content was squashed. Given the amount of information required a better format was simply to use separate sections for each class. Non-biochemical effects did not attract any marks. Similarly, an introductory statement about diuretics was not required and attracted no marks. Biochemical effects were not answered clearly or completely. Effect on acid-base was answered correctly by very few candidates. Vague statements such as “increased sodium” did not score as well as more precise terminology like “hypernatraemia” or “raised serum sodium”. A few answers incorrectly stated that the mechanism of action of thiazides was ENaC inhibition.
2020A 14 – 2019A 18
Describe the pharmacology of frusemide.
Examiner Comments
2020A 14: 51% of candidates passed this question.
Most candidates presented a well-structured answer and provided a basic understanding. Answers that provided accurate indications and details of the mechanism underlying the actions of frusemide attracted more marks. Those recognising the increased delivery of sodium and chloride to the distal tubule (exceeding resorptive capacity) were awarded more marks that those answers that attributed the diuretic action solely to reduction in the medullary gradient. Frusemide has many potential adverse effects and a reasonable list was expected. Conflicting information was common (e.g. highly bound to albumin – Vd 4 L/kg) and better answers avoided this.
2019A 18: 13% of candidates passed this question.
The majority of answers were well structured, some using tables and others using key headings. In general, for a commonly used drug that is listed in the syllabus as Level 1 of understanding, detailed information was lacking. In particular, mechanism of action, dose threshold and ceiling effect and pharmacokinetics lacked detail and/or accuracy.
2012A 16
Describe the biochemical abnormalities, and the mechanisms by which they arise, that may be observed in a patient who is taking frusemide.
CICMWrecks Answer
Frusemide:
- Loop Diuretic
- MOA:
- Inhibition of Na/K/2Cl symporter TAL of LoH.
- ↓ reabsorption of Na, K, Cl.
- Less positive luminal charge
Biochemical Abnormalities with Frusemide
Abnormality | Mechanism |
---|---|
Hyponatraemia | Blockade of Na/K/2Cl symporter – ↓ trans cellular Na reabsorption – loss of luminal positive charge → ↓ paracellular Na reabsorption |
Hypokalaemia | Blockade of Na/K/2Cl symporter – loss of paracellular reabsorption of K |
Hypochloraemia | Blockade of Na/K/2Cl symporter |
Hypomagnesaemia/hypocalcaemia | loss of luminal positive charge → ↓ paracellular reabsorption of Mg and Ca |
Hyperuricaemia | ↓ECF → ↑renin → ↑aldosterone → ↓ Urea secretion and ↑ tubular reabsorption of urea |
Hyperglycaemia | ↓ glycolysis in RBCs ↓ sensitivity of insulin-induced skeletal muscle uptake |
Metabolic Alkalosis | ↓ plasma chloride → ↑SID → alkalosis |
Gladwin 2016
Examiner Comments
2012A 16: 3 (30%) of candidates passed.
This was a relatively straightforward question with marks available for listing the abnormality and then discussing its origin. Many candidates simply listed an abnormality or confused the direction of electrolyte change. Few candidates went beyond hypokalaemia, hyponatraemia and hypochloraemia. Several candidates gave confused answers as to the mechanism(s) or drew pictures of a tubule with directional arrows for electrolytes with inadequate explanation. Some candidates simply ran out of time and wrote very little – this is a pity as a list would have generated marks. Candidates are reminded to practise the exams to time and attempt all questions.
2023B 08 – 2018A 16 – 2013A 20
Compare and contrast the pharmacology of furosemide (frusemide) and acetazolamide.
Examiner Comments
2023B 08: 31% of candidates passed this question.
Pharmacology questions largely have a standardised structure to follow; pharmaceutics, pharmacokinetics and pharmacodynamics. However compare and contrast questions require answering in a way that highlights important similarities and differences between the drugs chosen and the impact of these differences when administered. Whilst these are both diuretics they have very different renal and non-renal effects and thus very different metabolic and electrolyte disturbances. It was expected that these points be highlighted as well as the provision of other pharmacological information in order to pass this question.
2018A 16: 30% of candidates passed this question.
The use of a table assisted with both clarity and the ability to compare the two drugs. Writing separate essays about each makes it difficult to score well. It was expected that candidates would follow a standard pharmacology format and discuss pharmaceutics, pharmacokinetics, pharmacodynamics and adverse drug reactions. Both of these drugs are ‘Level A’ in the syllabus and a suitable level of detail was expected.
It was expected candidates would discuss in detail the mechanism of action, electrolyte and acid-base effects. Pharmacokinetic values were poorly answered. Qualitative terms such as ‘moderate, good and some’ are vague and should be avoided. Only correct numerical values (or ranges) attracted full marks.
2013A 20:
Frusemide and acetazolamide are commonly used drugs and candidates were expected to have a sound knowledge of their pharmacology, including (amongst other features) their site and mode of action, renal and other organ effects, adverse effects and essential pharmacokinetics.
2013A 10
Outline the pharmacokinetics, and mechanism of action of carvedilol and spironolactone.
Examiner Comments
2013A 10:
Carvedilol and spironolactone are common drugs used in the management of cardiac failure. They have different mechanisms of action and pharmacokinetics. Both are drugs listed in the Syllabus as Level B and thus candidates are expected to have a general understanding of their pharmacology. Many candidates gave class specific information about beta blockers rather than demonstrating an understanding of carvedilol`s particular properties. Most candidates were able to score marks by commenting upon the results of aldosterone antagonism suggesting an understanding of the physiology of this hormone but appeared to know little more about the pharmacology of spironolactone. Overall there was insufficient information provided by most candidates for both drugs.
ii. Understand the pharmacology of renal replacement fluid.
Click here for SAQs (H2.i. Describe the principles of dialysis and filtration.)
H4. Renal Measurement
i. Describe the principles of measurement of glomerular filtration rate and renal blood flow.
2016B 03
Describe the factors that determine glomerular filtration rate (GFR) in the kidney (70% of marks). Outline methods by which GFR can be measured (30% of marks).
CICMWrecks Answer – GFR, Determinants, Factors
GFR
GFR:
- “The amount of glomerular ultrafiltrate formed divided by the time of filtration”
- Normal value is approx 125 mL/min or 180 L/day
- Renal blood flow 1.25l/min in 70kg male → Filtration fraction 0.2
Functional anatomy:
3 Distinct Layers:
- glomerular capillary endothelium
- highly specialised endothelium with fenestrations to ↓ filter thickness
- prevents cellular components of blood from coming into contact with BM
- glomerular BM
- made of CT; -vely charged
- acts as filter
- bowmans epithelial cells (podocytes)
- epithelial cells with foot processes → large SA
- negatively charged
- maintain BM + phagocytic functions
Direct GFR Determinants
The GFR (Net flux across the membrane) is the balance of hydrostatic pressure and oncotic pressure, as defined by the Classic Starling Equation.
where
Kf = Filtration coefficient
P = hydrostatic pressure
π = oncotic pressure
σ = Staverman’s reflection coefficient ie. Permeability of membrane to protein
- Normally NFP = 17mmHg
- Tubular oncotic pressure = zero throughout
- GC oncotic pressure varies from 21 to 33 mmHg as filtrate is removed.
- Thus less GFR produced at distal end of tubule.
Afferent end of Glomerular Capillary (mmHg) | Efferent end of Glomerular Capillary (mmHg) | |
---|---|---|
PGC | 60 | 58 |
PT | 15 | 15 |
πGC | 21 | 33 |
NFP | 24 | 10 |
Kf | Filtration coefficient | = LpS = Hydraulic conductivity x Surface Area Glomerular surface area = 0.8m2 • Altered by Mesangial cell contraction (see circulating factors e.g. Angiotensin II → ↓SA → ↓GFR) Patency of the normal capillary wall structure (ie in tubular dysfunction Kf ↑’s ↑GFR) |
PGC | hydrostatic pressure in capillary | relates to • RBF which is autoregulated for MAP 70-170mmHg • relative afferent/efferent arteriolar tone Affected by • Catecholamines • Local autoregulation -> Myogenic -> Tubuloglomerular feedback -> Hormones (see below) |
PT | hydrostatic pressure in tubule | relates to • obstruction to urinary flow (usually pathological) • ↑PT → ↓ GFR (ie post renal obstructiion causing renal failure) |
πGC | oncotic pressure in capillary | relates to • plasma protein concentration (incr in dehydration, decreased in heart failure) • ↑Systemic plasma oncotic pressure → ↑πGC → ↓GFR • ↓Renal plasma flow → ↑πGC → ↓GFR |
πT | oncotic pressure in tubule | • usually zero, but can increase in renal failure/proteinuria • ↑’d πT → ↑GFR |
σ | Staverman’s reflection coefficient | • Permeability of membrane to protein usually 1 (no protein leak) • can decrease with nephritis/proteinuria |
Factors influencing GFR
Circulating Factors
- Prostaglandins
- PGI2 and PGE2 → vasodilates → ↑ renal blood flow → ↑ GFR
- ↓ PGE2, PGI2 and NO levels → inhibits afferent arteriolar vasodilation → ↓ GFR
- Long term → ↓ renin release → ↓ ATII-induced efferent arteriolar vasoconstriction → ↓ GFR
- Noradrenaline / Adrenaline
- constrict renal afferent and efferent arterioles → ↓ renal blood flow and reducing filtration → ↓GFR
- Constrict mesangial cells to → ↓ GFR.
- Mesangial cell tone:
- Angiotensin 2, Endothelin, vasopressin → ↓ glomerular surface area and GFR
- ANP, PGE2, Dopamine and cAMP → all increase GFR
Solute factors
- Size
- < 7 kDa (Eg. glucose, ions, urea, H2O) filtered freely,
- > 70 kDa (Eg. albumin) are not filtered;
- neutral particles < 4 mm diameter filtered freely, > 8 mm are excluded
- Charge
- Negatively charge (Anionic) particles repulsed
- Cationic substances filtered more readily
- Protein binding
- Albumin excluded
Disease Factors
- Filtration decreases
- Shock → decreased glomerular pressure
- Obstruction → increased bowman’s capsule hydrostatic pressure
- Hypoproteinaemia → hepatic failure, nephrotic syndrome
JC / Gladwin / Sakurai / Kerr 2020
CICMWrecks Answer – GFR Measurement
GFR Measurement
Clearance:
- Renal clearance = vol of plasma completely cleared of a given substance by the kidneys per unit time (ml/min)
- Involves: glomerular filtration, secretion, reabsorption, and rarely tubular metabolism
- Renal clearance = V x [U]/[P]
- V = volume of urine or urine flow rate in ml/min
- [U] = urinary concentration of substance in mg/ml
- [P] = plasma concentration of substance in mg/ml
Estimating GFR:
- GFR = renal clearance of a substance if it is:
- Freely filtered at glomerulus
- Not secreted
- Not reabsorbed
- Not synthesized
- Not metabolised
- The amount excreted in the urine = amount filtered
- i.e. [plasma] x GFR = [urine] x urine vol
- Rearrange: GFR = urine vol x [urine] / [plasma]
Substances used to estimate GFR:
- Criteria
- easily measured in urine + plasma
- non-toxic
- easily administered
- Inulin
- Protein; polymer of fructose
- MW 5800 Da
- freely filtered
- Not reabsorbed, secreted, metabolised, or synthesized by the kidney
- Therefore clearance of inulin = GFR
- Requires continuous infusion for several hours to achieve steady state
- Creatinine
- used to approximate GFR as is more practical
- Released at a steady state from skeletal muscle cells (phosphocreatine)
- Freely filtered + not reabsorbed
- *Small amount secreted → overestimates GFR by small amount
- Cockcroft Gault formula used
JC / Gladwin / Sakurai / Kerr 2020
Examiner Comments
2016B 03: 57% of candidates passed this question.
Good answers included a description of Starling forces acting at the glomerular basement membrane. A description of the local and systemic factors influencing each component was expected.
It was expected candidates would discuss autoregulation of GFR & RBF, tubuloglomerular feedback, and integrated responses the body uses to keep GFR steady. Confusion about the nature of induced effects on afferent or efferent arteriolar dilation and constriction limited marks for some candidates. Many failed to mention the effects of mesangial surface area, Bowmans space pressure or serum protein content.
Candidates were expected to outline the methods of GFR estimation. Better responses described the rationale behind the use and limitations. Creatinine clearance, inulin and nuclear medicine techniques all scored marks. Some candidates made no attempt at this section and missed the opportunity to score marks. Estimates of CrCl/GFR [eGFR by formulae such as Cockcroft Gault, and serum Cr] are not measurement of GFR.
ii. Describe the utility of biochemical estimates of renal function (including but not limited to the measurement of serum creatinine, cystatin C and estimates of Creatinine Clearance such as eGFR).
2022A 07
Write notes comparing the use of serum creatinine and creatinine clearance in the assessment of renal function in the critically ill.
2013B 11 – 2008A 13
Describe the relationship between creatinine clearance and serum creatinine. (60% of marks).
What are the limitations in using serum creatinine to assess renal function in the critically ill? (40% of marks)
CICMWrecks Answer
Clearance:
- Renal clearance = vol of plasma completely cleared of a given substance by the kidneys per unit time (ml/min)
- Involves: glomerular filtration, secretion, reabsorption, and rarely tubular metabolism
- Renal clearance = V x [U]/[P]
- V = volume of urine or urine flow rate in ml/min
- [U] = urinary concentration of substance in mg/ml
- [P] = plasma concentration of substance in mg/ml
Estimating GFR:
- GFR = renal clearance of a substance if it is:
- Freely filtered at glomerulus
- Not secreted
- Not reabsorbed
- Not synthesized
- Not metabolised
- The amount excreted in the urine = amount filtered
- i.e. [plasma] x GFR = [urine] x urine vol
- Rearrange: GFR = urine vol x [urine] / [plasma]
Creatinine
- used to approximate GFR as is more practical
- Released at a steady state from skeletal muscle cells (phosphocreatine)
- Freely filtered + not reabsorbed.
- *Small amount secreted → overestimates GFR by small amount
- Serum creatinine levels can be used as a surrogate marker of GFR
- Creatinine clearance calculation better than creatinine levels
- most accurate to collect urine and use the formula to assess creatinine clearance
- because serum creatinine it is at steady state, eGFR can be calculated by Cockroft-Gault, MDRD or CKD-Epi
- During acute changes in GFR, serum creatinine will underestimate GFR until a new steady state is reached.
Creatinine Clearance Estimation from Serum Creatinine
- CG (Cockcroft-Gault Equation): common method which has a correlation of ~0.83 with CrCl:
- CrCl = [(140−A) × W x S)] / (72 × Cr) , where:
- Cl = Clearance (mL/min), A = Age, W = Lean body Wt (kg)
- S = Sex coefficient (Male = 1, Female = 0.85), Cr = Creatinine in µmol.L-1
- CrCl = [(140−A) × W x S)] / (72 × Cr) , where:
- Alternative formulas are MDRD and CKD-EPI. These equations have two advantages over Cockcroft-Gault:
- They are better predictors of GFR
- They do not require weight, and so can be calculated by the laboratory automatically, Other required data (gender, race, age, creatinine) can be taken from hospital records.
- MDRD (Modification of Diet in Renal Disease Study): useful in estimating glomerular filtration rate (GFR) in stable chronic kidney disease
- CKD-EPI (Chronic Kidney Disease Epidemiology Collaboration): more precise formula to estimate glomerular filtrate rate (GFR) from serum creatinine and other readily available clinical parameters, especially at when actual GFR is >60 mL/min per 1.73m2
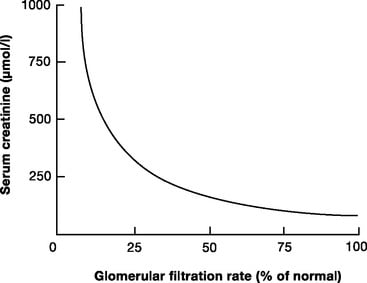
Limitations
- General limitations
- Dependent on serum creatinine, which can be highly variable. Formulas are derived from average values of dependent variables, and so will be unreliable at extremes of:
- Age
- Muscle mass
- Critically ill
- Malignancy
- Diet (High production with red meat)
- the relationship between creatinine clearance and serum creatinine is non-linear
- filtration is only one component of a complex kidney, although GFR is used as a surrogate of function
- Small amount of creatinine is secreted by proximal tubule – so Creatinine clearance is ~10-20% higher than GFR
- More severely overestimates GFR in patients with very low GFR
- Dependent on serum creatinine, which can be highly variable. Formulas are derived from average values of dependent variables, and so will be unreliable at extremes of:
- Critically ill patients
- the amount of creatinine produced varies with muscle mass, nutrition, steroid use, muscle injury
- there can be a decline of almost 50% of function before serum creatinine levels rise
- they do not indicate dynamic changes in renal function
- are modified by aggressive fluid resuscitation
JC 2019
Examiner Comments
2022A 07: 11% of candidates passed this question.
It was expected that candidates would define both components of the question, discuss the factors which affect serum creatinine and creatinine clearance and demonstrate their interrelationship. More complete answers described the advantages, disadvantages and limitations of their use in critical illness. In many cases candidates failed to correctly define creatinine clearance. Answers that scored well clearly utilised the above-mentioned breadth of knowledge and linked these to pertinent specific changes that may be associated with critical illness.
2013B 11: 4 candidates passed (14.8%).
This was a straightforward question of core CICM material. Most candidates were able to describe the Fick equation as it related to clearance, and then relate it to Glomerular Filtration Rate (GFR). Formula relating GFR to serum creatinine were often quoted incorrectly, graphs poorly constructed and/or labelled and many answers were very superficially answered.
Better candidates were able to relate the graph to functional nephron loss and hence serum creatinine. The non-linear relationship between nephron mass (and function) and the serum creatinine was poorly appreciated by many. The nature of the variability of creatinine production with age, sex, ethnicity etc. was often omitted, as were the factors involved in the variability in an ICU patient.
2008A 13: No candidates (0%) passed this question.
It was expected candidates would describe that both these are surrogate measures for Glomerular filtration rate. Credit was given for clear definitions, formula and normal values (such as plasma clearance is the volume of plasma cleared per unit time). It was expected candidates could explain that serum creatinine results from a balance of creatinine produced and excreted and hence the slow response time and limitations for its use because of changes in both production and excretion.
Extra credit was given for appreciating the non linear relationship between changes in serum creatinine and creatinine clearance and that significant changes in glomerular filtration can occur before this is reflected in the serum creatinine. Comment on the problems of dilution with acute changes in fluid balance and that tubular secretion of creatinine can occur when the serum creatinine concentration is high both gained extra marks.
Syllabus D1 2
Reference Ganong 22nd edition 699-728
VIVAs
2023B | Renal blood flow, anatomy of renal blood supply Renal handling of potassium Renal handling of bicarbonate |
2023A | Name and describe the components of nephron |
2022B | Renal vasculature, explain divisions beyond arcuate artery Renal blood flow, regulation |
2022A | Urea production, effective osmole, renal tubular function Renal blood flow regulation Principles of haemodialysis |
2021B | Renal water handing, concentrates urine Renal physiology, ABG interpretation (Temp 37.5 pH 7.31 pCO2 39 pO2 84 fiO2 30% HCO3 18 BE -4 Ba 136 K 3 Cl 101 Lactate 3.4) |
2021A | Principles of haemodialysis |
2020B | Bowman’s capsule, renal anatomy and physiology |
2019B | Functions of kidney Renal handling of sodium |
2019A | Renal clearance, GFR measurement |
2018B | Renal blood flow, JXG apparatus Diuretics – pharmacology, MoA |
2018A | Renal clearance and aspects of the ECG. Describe the physiological processes which cause oliguria in response to acute hypovolaemia? |
2017B | renal physiology and potassium |
2017A | physiological causes of polyuria |
2016B | Renal clearance, Diuretics |
2016A | |
2015B | |
2015A | renal physiology and related pharmacology |
2014B | |
2014A | renal physiology and diuretic pharmacology |
2013B | Renal physiology and pharm, GFR and determinants, values, hypovolaemia scenario, cointer current mechanism and multiplier effect, diuretics |
2013A | |
2012B | |
2012A | |
2011B | |
2011A | Renal function, hyperkalaemia, cardiac consequences (Action Potential, ECG) |
2010B | Renal circulation, blood oxygen tension in kidney, autoregulation, GFR and determinants |
2010A | |
2009B | Urinalysis, Ketone, Urobiilinogen, Specific gravity Nephron – physiology, blood supply |
2009A | |
2008B | |
2008A | ABG – Metabolic alkalosis with resp compensation – Renal physiology |
2007B |
Recent Comments