Syllabus (Fourth Edition, 2023)
Topics
i. Describe the physiological consequences of intermittent positive pressure ventilation and positive end-expiratory pressure.
ii. Explain the physiological effects of hyperoxia, hypoxaemia, hypercapnia and hypocapnia.
iii. Explain the effect of changes in posture on ventilatory function.
iv. Define humidification, outline the mechanisms of humidification and its importance.
v. Explain the pathways and importance of the cough reflex.
vi. Outline the non-ventilatory functions of the lungs.
Topics not covered in previous SAQs
i. Describe the physiological consequences of intermittent positive pressure ventilation and positive end-expiratory pressure.
ii. Explain the physiological effects of hyperoxia, hypoxaemia, hypercapnia and hypocapnia.
iii. Explain the effect of changes in posture on ventilatory function.
Learning Objectives for the First Part Examination in Intensive Care Medicine
- This will ensure that trainees, tutors, and examiners can work from a common base.
- All examination questions are based around this Syllabus.
- These learning objectives are designed to outline the minimum level of understanding required for each topic.
- The accompanying texts are recommended on the basis that the material contained within them provides sufficient information for trainees to meet the learning objectives.
- Trainees are strongly encouraged to explore the existing and evolving body of knowledge of the Basic Sciences as they apply to Intensive Care Medicine by reading widely.
- For all sections of the syllabus an understanding of normal physiology and physiology at extremes of age, obesity, pregnancy (including foetal) and disease (particularly critical illness) is expected.
- Similarly, for pharmacology, trainees are expected to understand a drug’s pharmacology in these contexts.
- An understanding of potential toxicity and relevant antidotes is also expected.
Definitions
Throughout the document specific wording has been used under the required abilities to indicate the level of knowledge and understanding expected and a glossary of these terms is provided.
Definitions
Calculate | Work out or estimate using mathematical principles. |
Classify | Divide into categories; organise, arrange. |
Compare and contrast | Examine similarities and differences. |
Define | Give the precise meaning. |
Describe | Give a detailed account of. |
Explain | Make plain. |
Interpret | Explain the meaning or significance. |
Outline | Provide a summary of the important points. |
Relate | Show a connection between. |
Understand | Appreciate the details of; comprehend. |
SAQs
i. Describe the physiological consequences of intermittent positive pressure ventilation and positive end-expiratory pressure.
2023A 08
Describe the cardiovascular and respiratory effects of positive pressure ventilation.
2019A 20
Describe the cardiovascular effects of positive pressure ventilation on a patient who has received a long acting muscle relaxant.
2016A 02
Describe the respiratory and cardiovascular effects of applying 10 cm of PEEP (positive end-expiratory pressure) to a healthy mechanically ventilated adult.
2014B 03 – 2009A 17
Describe the physiological consequences of Positive End-Expiratory Pressure (PEEP).
CICMWrecks Answer
Positive Pressure Ventilation
- PEEP = Positive End Expiratory Pressure.
- Equivalent to a constant pressure applied throughout the respiratory cycle.
- Intrinsic PEEP = unintentional or un-measured end-expiratory hyperinflation
- Physiological effects of Positive Pressure Ventilation mostly related to increased mean airway pressure
Cardiovascular Effects
- Causes constant ↑ intrathoracic pressure (ITP) throughout respiratory cycle
- Left Heart
- On Preload
- Initially: → ↑ LV PL → ↑ CO
- Secondarily: → ↓RV output → ↓ LV PL → ↓ CO
- On Afterload
- → ↓myocardial transmural pressure → ↓LV AL → ↑CO
- PEEP > Ao diastolic pressure
- → Collapse of intrathoracic aorta → Starling resistor mechanism → ↑LV AL → ↓ CO
- PEEP < Ao diastolic pressure
- ↑ pressure gradient for flow to systemic circulation
- ↓ AL → ↓ myocardial work
- On Compliance
- diastolic buldging of septum → ↓ LV compliance → ↓LV CO
- On Preload
- Right Heart
- → ↑ Pulmonary vascular resistance → ↑ RV AL → ↓ RV output
- → ↓ Venous return → ↓ RV PL → ↓ RV output
- In the failing LV → ↑CO
- ↓ LV afterload
- ↑ pressure gradient thorax to abdomen
- ↓ transmural pressure → ↓ LV wall tension = ↓afterload
- ↓ LV preload (ie more favourable position on compliance curve)
- ↓ LV afterload
Respiratory Effects
Beneficial Effects:
- ↓ atelectasis and gas trapping
- ↑’s FRC > “closing capacity”
- Shifts position on P-V curve right, above the “closing point”
- ↑ lung compliance → shifts back to steep/compliant part of P-V curve
- ↓ intrapulmonary shunt thus improved V/Q match and ↑ PaO2
- ↓ atelectasis and gas trapping
- ↓ extravascular lung water → ↓interstitial/alveolar oedema
- ↓ AWR
- ↑ lung volume → ↑ radial traction by parenchyma → ↑ airway calibre
- ↓ work of breathing
- ↑ lung compliance = ↓ elastic work
- ↓ AWR = ↓resistance work
Negative Effects:
- ↑ V/Q mismatch
- ↑ west zone 1
- ↓ lung compliance
- shift to flat part of P-V curve
- ↑ Work of breathing (due to compliance change)
- ↑ PVR and RV afterload
- extrinsic compression of pulmonary vessels
- Barotrauma
End-Organ Effects
- Renal:
- ↓CO & ↑renal venous pressure
- → Reduced renal blood flow → Reduced GFR → Reduced urine output
- → Reduced atrial stretch and ANP release → Increased ADH → Fluid retention → Oedema
- ↓CO & ↑renal venous pressure
- Hepatic:
- Reduced hepatic blood flow due to:
- Increased CVP and decreased CO lowering the pressure gradient for hepatic flow
- May result in circulation only intermittently throughout the cardiac cycle
- Hepatocyte dysfunction
- Haematological:
- Neutrophil sequestration in the compressed pulmonary vasculature
- CNS:
- ↓VR ⇒ ↑CVP ⇒ ↑ICP
Gladwin / Mooney / JC 2020
Examiner Comments
2023A 08: 21% of candidates passed this question.
This question required a breadth of response that was largely unrepresented in the answers provided by candidates. Better answers divided responses into cardiovascular effects and respiratory effects however many did not provide sufficient detail or provided only detail on some of the effects and missed the breadth of the question. Cardiovascular effects could be grouped into effects on the; right heart, left heart, ventricular interdependence, cardiac output, other circulations, baroreceptor and hormonal reflexes. Respiratory effects could be grouped into lung volumes, dead space, V/Q matching, compliance, airway resistance and the effect of excessive pressures on the lung. Despite this division in the question many candidates abandoned a structure which made their answers and explanations lack clarity. The examiners commented that most candidates who wrote an answer in paragraph format were likely to provide long vague answers rather than those more factual and succinct answers presented in a format with headings as above and pertinent associated dot point descriptions underneath these headings.
2019A 20: 33% of candidates passed this question.
Structured answers separating effects of positive pressure on right and left ventricle, on preload and on afterload were expected. Overall there was a lack of depth and many candidates referred to pathological states such as the failing heart. Simply stating that positive pressure ventilation reduced right ventricular venous return and/or left ventricular afterload, without some additional explanation was not sufficient to achieve a pass level.
2016A 02: 29% of candidates passed this question.
This topic has been asked previously. It was expected candidates could detail the impact of PEEP on a variety of respiratory parameters such as lung volume, dead space, arterial pO2 and intrapleural pressure. The cardiovascular consequences are well described including the effect on cardiac output, blood pressure and oxygen delivery. The physiological impact of lower levels PEEP in a young healthy person is different to that often seen in the critically ill and this was not appreciated by most candidates.
2014B 03: 27% of candidates passed this question.
Most answers were quite brief and superficial. They simply did not cover enough of the required knowledge base to gain a pass mark. A definition of PEEP is a useful way to start this answer and this was missing in more than half the answers. Deficiencies in knowledge included even the primary respiratory and cardiovascular effects of PEEP. Many candidates incorrectly concluded that PEEP would increase afterload and decrease pulmonary vascular resistance. Some candidates provided
description of the cardiovascular effects of Valsalva, which was not part of the question. It was expected candidates would also mention physiological effects on other organ systems such as potential cerebral and renal effects.
This topic (Level 1) requires a detailed knowledge and candidates should read widely to gain the depth of understanding required. The core material is covered in texts such as Nunn’s’ Applied Respiratory Physiology and additional applied information can be found in a variety of texts such as Textbook of Critical Care by Fink et al, Irwin and Rippe’s Intensive Care Medicine or Miller’s Anaesthesia.
2009A 17: Pass rate: 40%
Points required included a definition of PEEP, both intrinsic and extrinsic.
The important physiological consequences that need to be discussed are respiratory including increased FRC, increased compliance and decreased work of breathing.
Cardiovascular consequences include decreased venous return and subsequently decreased cardiac output and an increased pulmonary vascular resistance.
Renal consequences include decreased renal blood flow and increased ADH
Effects on intra-abdominal pressure, hepatic blood flow and the beneficial effects in cardiac failure earned marks.
Syllabus B1k.2a
Reference: Nunn 6th edition p. 431.9
ii. Explain the physiological effects of hyperoxia, hypoxaemia, hypercapnia and hypocapnia.
2011A 01
Describe the physiological consequences of breathing 100% oxygen at sea level.
CICMWrecks Answer
Therefore, owing to equation (2) and the haemoglobin dissociation curve, at PaO2 > 100mmHg there is:
Only a minimal increase in haemoglobin saturation
Only a minimal increase in dissolved oxygen concentration
Oxygen stores
Functional residual capacity = lung volume with no active insp. or exp. effort
= volume when equilibrium between chest wall recoil and lung elastic recoil
If FiO2 increased → more oxygen in FRC (not exhaled with tidal volume)
→ Able to tolerate a period of hyperventilation
Absorption atelectasis
- Nitrogen is an inert gas, comprising ~78% of room air
- As it is biologically inert, it is in equilibrium throughout body tissues
- There is no concentration gradient to cause absorption from the lungs
- If FiO2 increased
- PiO2 of O2 increases
- PaN2 decreases as a result
- Moves out of alveoli down its concentration gradient
- ∴Total alveolar pressure falls -> alveolar collapse
- Clinically significant when FiO2 > 50%
Hypoxic vasoconstriction
- In under ventilated lungs portions, there is adaptive pulmonary vasoconstriction, reducing V/Q mismatching
- If FiO2 increased
- Under ventilated lung portions have a higher PaO2
- V/Q mismatching -> venous admixture, ↓ pulmonary vein PAO2
- Under ventilated lung portions have a higher PaO2
Haldane effect
Deoxygenated haemoglobin has a higher affinity for CO2 than oxygenated Hb
If SaO2 is artificially raised, lower CO2 carrying capacity -> build-up of CO2 in tissues
Oxygen radicals
- A radical is a molecule with an unpaired electron outside of an electron shell
- Highly reactive, cause tissue damage
- Important reactive oxygen species (ROS): Superoxide radical (O2–); peroxide radical (O22-); hydroxide radical (•OH)
- ROS can usually be eliminated by cellular antioxidant defence mechanisms
- When FiO2 > 50%
- Defense mechanisms overwhelmed
- Paranchymal injury
- Tracheobronchitis
- Pulmonary capillary endothelial damage -> pulmonary oedema + fibrosis
Mooney 2016
Examiner Comments
2011A 01: 6 (60%) of candidates passed
The question related to physiological changes occurring when FiO2=1. Many candidates focused on the toxic effects of oxygen, which were often incorrect (CNS symptoms will not occur at one atmosphere). Candidates simply lacked knowledge, those that did have some understanding failed to provide adequate detail (ie. it was occasionally mentioned oxygen stores are increased but not the mechanism by which or extent to which stores are increased). In addition, it was expected that candidates would outline and describe the mechanism behind the changes in PaO2in arterial and mixed venous blood, shift in CO2 ventilation, hypoxic pulmonary vasoconstriction as well as pulmonary toxic effects.
2018B 14
Explain the detection and response to hypoxaemia.
2012A 01
Outline the physiological detection and response to an acute decrease in PaO2
CICMWrecks Answer
Hypoxaemia
Hypoxaemia = Acute ↓ PaO₂ = (low partial pressure of oxygen in arterial blood)
Overall response: Hypoxaemia → ↑ peripheral vasosmotor tone, ↑rate and depth of respiration
Local response to ↓ PaO₂:
- ↑ local vasodilation → ↓ SVR and ↓ BP
- ↓ BP → Baroreceptors → SNS activation → ↑ HR and ↑ C.O.
- PaO2 < 50-60 mmHg
- → peripheral chemoreceptors stimulation (see below)
- → ↑ventilation
- If ↓ PaO₂ is Gradual ↑ MV PaO2 < 500mmHg,
- If ↓ PaO₂ is Rapid ↑MV PaO2 < 50 mmHg
- Severe hypoxaemia
- ↓ HR and BP → shock
- ↑ CBF (regardless of PaCO2)
Chemoreceptor (CR) Reflex:
Two types of CR:
- Central CR
- CR location: Below the ventral surface of the medulla
- Stimuli: Δ ECF [H+] whereby ↑ ECF [H+] stimulates ↑ ventilation
- Rapid Response:
- CO2 rapidly diffuses into CSF
- Low CSF protein and HCO3 (c.f. plasma)
- Poor buffering capacity
- ΔCSF pH per ΔPaCO2 is GREATER than that of blood
- Peripheral CR
- CR location:
- “Carotid bodies” (more important)
- at bifurcation point of common carotid arteries
- supplied by CN IX
- “Aortic bodies” (less important)
- above and below the aortic arch
- supplied by CN X
- “Carotid bodies” (more important)
- Composition:
- PCRs found in “Glomus cells”
- ↓ PaO2 – via inhibition of O2 sensitive K-channels
- ↑ PaCO2 and/or ↓pH – via effect on pH sensitive K-channels
- Type I cells (rich in NAd, DA, ACh)
- Hypoxia causes release of NTs
- NAd/ACh – ↑ AP firing rate of AB or CB afferent fibres
- DA – Damping of type 2 cell responses
- Hypoxia causes release of NTs
- Type II cells (rich in capillary supply)
- ↓ PaO2, ↑ PaCO2 and/or ↓pH
- ↓ IC [ATP] which leads to ↑ NT production and release
- ↑ AP firing rate of AB or CB afferent fibres
- PCRs found in “Glomus cells”
- Response:
- ↓’d perfusion → ↑[H]/↓PaO2 → CR stimulation
- Afferent signal → Sinus nerve (of herring) / Vagus Nerve → Chemosensitive area of medulla
- Vasomotor area → ↑ peripheral vasoconstriction
- Respiratroy center → ↑rate and depth of respiration → ↑ venous return
- CR location:
Chronic Response
- Adrenal medullary hypoxia → EPO
- ↑ erythropoiesis (chronic)
Gladwin 2016
Examiner Comments
2018B 14: 34% of candidates passed this question.
A logical approach to answering this question included a definition of hypoxaemia and then a discussion of the sensors, integrators and effectors involved. It was expected that candidates would cover the peripheral chemoreceptor response (including the respiratory, cardiovascular and autonomic effects), time course of the ventilatory response, hypoxia-inducible factors, vascular effects (hypoxic vasoconstriction in the pulmonary circulation and vasodilatation in the systemic circulation) and metabolic changes (switch to anaerobic metabolism). No marks were awarded for discussing the causes of hypoxaemia. Many candidates incorrectly stated that hypoxaemia is detected by the central chemoreceptors.
2012A 01: 0 (0%) of candidates passed.
Candidates were expected to focus on the physiology (including mechanisms) involved with detection of hypoxia (e.g. peripheral chemoreceptor stimulation) as well as response. Examples of response included sympathetic stimulation, the respiratory centre and organ specific (e.g. cardiac, CNS, blood, cellular, etc) responses. This topic is well covered within a number of fundamental texts, in particular, Nunn’s Respiratory Physiology. In general candidates’ answers were not “broad” enough, lacked detail and were too focused on chemoreceptors only.
2010B 01
Discuss the physiological causes of early post-operative hypoxaemia
CICMWrecks Answer
Hypoxaemia
- Hypoxaemia = Acute ↓ PaO₂ = (low partial pressure of oxygen in arterial blood)
- Normal PaO2 = 75-100mmHg
- Hypoxaemia can lead to tissue hypoxia and subsequent adverse effects
Causes of early post-operative hypoxaemia:
- Low inspired oxygen concentration
- PAO2 = PiO2 – PCO2/R + F, where PiO2 = (PATM-47mmHg) x FiO2
- FiO2 may be insufficient to meet the metabolic demands of the patient
- may been ↑due to recent surgery
- Hypoventilation
- VA = (TV – dead space) x RR
- may be due to:
- pain
- respiratory depression 2° drugs (opioids, barbiturates)
- paralysis of respiratory muscles
- damage to chest wall
- high resistance to breathing
- results in a ↓PaO2 and a ↑PaCO2
- easily reversed by increasing FiO2
- along with V/Q scatter are the major causes of post-operative hypoxaemia
- V/Q Ratio Differences
- V/Q scatter refers to areas of low ventilation compared with perfusion
- atelectasis
- V/Q mismatch refers to areas of low perfusion compared with ventilation
- severe hypotension
- PE
- V/Q scatter refers to areas of low ventilation compared with perfusion
- Shunt
- V/Q = 0
- refers to blood that enters the arterial system without going through ventilated areas of lung
- cannot be abolished by an ↑FiO2
- may be anatomical or physiological
- Increasing oxygen consumption
- may be due to:
- pyrexia (fever)
- shivering
- hypermetabolic states (thyrotoxicosis, malignant hyperthermia)
- may be due to:
- Diffusion barrier
- very minor role in healthy patients (likely to be present pre-op though)
- PaO2 usually very close to PAO2 but never quite equal
- PaO2 will decrease with blood-gas barrier thickening as it ↑ the diffusion distance between the alveolar gas and pulmonary capillary blood
- Fick’s Law of Diffusion
- Increasing Age:
- the PaO2 ↓ with ↑age from about 20 years of age
- this is due to closing capacity being greater than FRC
blood draining from these alveoli has a lower PO2
JC 2020
Examiner Comments
2010B 01: 5 (33%) of candidates passed this question.
For a good mark it was expected that candidates define hypoxaemia and, in a structured manner, discuss the physiological causes of early postoperative hypoxaemia. Candidates should always try and begin their answer with a definition of the term that is to be discussed. Many candidates invoked clinical disease related causes and not physiological. This did not score marks. Similarly, factors leading to tissue hypoxia, such as anaemia or low 2,3-dpg were not given marks. Candidates may have done so because they confused “hypoxaemia” with “hypoxia”. Better answers addressed different physiological causes of hypoxaemia, including areas of low V/Q; hypoventilation; increased oxygen consumption; loss of hypoxic pulmonary vasoconstriction and closing capacity exceeding functional residual capacity.
Syllabus: B1a2b, B1e,f,g
References: Nunn’s respiratory physiology – various sections
2007B 11
Describe the acid-base changes that occur in acute hypoxaemia.
CICMWrecks Answer
Hypoxaemia:
= Hypoxic Hypoxia= decreased PaO2 due to problems at the level of the lungs
Causes:
- Normal A-a Gradient
- Alveolar hypoventilation – neuromuscular disease
- Low PiO2 – FiO2 < 0.21, Barometric pressure < 760mmHg
- Raised A-a Gradient
- Diffusion defect – COPD, Pulmonary fibrosis, exercise
- V/Q Mismatch – Aging
- Right-to-left shunt – PDA, PFOs, ASDs or VSDs
- Increased O2 extraction – Sepsis
Physiological Outcome:
- ↑ local vasodilation → ↓ SVR and ↓ BP
- ↓ BP → Baroreceptors → SNS activation → ↑ HR and ↑ C.O.
- PaO2 < 50-60 mmHg
- → peripheral chemoreceptors stimulation
- → ↑ventilation
- Severe hypoxaemia
- ↓ HR and BP → shock
- ↑ CBF (regardless of PaCO2)
- Lactic Acidosis:
- Embden-meirhoff continues (glycolysis is anaerobic)
- Mitochondrial hypoxaemia → ↓d ETC (electron transport chain) activity → ↑ cytoplasmic Acetyl CoA → ↓ conversion of Pyruvate to Acetyl CoA → ↑ [Pyruvate] → ↑ shunting of pyruvate to lactate → ↓ SID → Acidosis (↑ [H])
Chemoreceptor Reflex
- Mechanism:
- ↓’d perfusion → ↑[H]/↓PaO2 → CR stimulation
- Afferent signal → Sinus nerve (of herring) / Vagus Nerve → Chemosensitive area of medulla
- Vasomotor area → ↑ peripheral vasoconstriction
- Respiratory centre → ↑rate and depth of respiration → ↑ venous return
- ↓’d perfusion → ↑[H]/↓PaO2 → CR stimulation
- Result
- Hypoxia/hypercaponea → ↑ peripheral vasosmotor tone, ↑rate and depth of respiration
- ↓ PaO2
- Gradual ↑ MV PaO2 < 500mmHg,
- Rapid ↑MV PaO2 < 50 mmHg
- ↓ PaO2
- Hypoxia/hypercaponea → ↑ peripheral vasosmotor tone, ↑rate and depth of respiration
Acid-Base changes:
- ↑[H] → ↑rate and depth of respiration → Respiratory alkalosis
- ↑ HCO3 if chronic (> 3 days)
- ↑[lactate] → ↑ Anion gap
CICMWrecks 2016
Examiner Comments
2007B 11: 1 candidate (14%) passed this question.
The main points expected for a pass were
- Definition of hypoxia
- Physiological causes of hypoxaemia
- Formation of a lactic acidosis
- Development of hypocarbia secondary to stimulation of respiration from hypoxia and acidosis
- Explanation of the decreased bicarbonate concentration and negative base excess
- Extra points were given for more detailed explanation of the lactic acidosis, commenting on the anion gap and some consequences of hypoxaemia.
A common problem was to incorrectly state the relationship between pH and hydrogen ion concentration.
2012B 21
Describe the physiological consequences of a progressive rise in blood carbon dioxide levels.
CICMWrecks Answer
Normal Ranges
- Normal 35 < PaCO₂< 45mmHg
- Moderate hypercaponea 45 < PaCO₂< 80mmHg
- Severe hypercaponea 80mmHg < PaCO₂
Sensors
Central Chemoreceptor (CCR) | Peripheral Chemoreceptor (PCR) | |
---|---|---|
Location | Below the ventral surface of the medulla Within the BBB | In Glomus cells of: • Carotid bodies: At the bifurcation of the common carotid arteries • Aortic bodies: Above and below the aortic arch. |
Mechanism | CO2 rapidly diffuses into CSF • Converted to H+ by carbonic anhydrased • Δ ECF [H+] closes pH sensitive K-channels • ↑ ECF [H+] stimulates ↑ ventilation • CSF has: – Low CSF protein and HCO3 (c.f. plasma) – Poor buffering capacity – Thus ΔCSF pH per ΔPaCO2 is GREATER than that of blood | Type 2 cells: • ↓ PaO2, ↑ PaCO2 and/or ↓pH • ↓ IC [ATP] which leads to ↑ NT production and release • ↑ AP firing rate of AB or CB afferent fibres |
Contribution to ↑MV | 80% | 20% |
Response Speed | Fast | Relatively fast compared with CCR |
Physiological effects of Hypercaponea
Moderate 45mmHg < PaCO₂<100mmHg
- Resp
- CO₂ strongest determinant of ↑MV (2-3 L/min per mmHg)
- Hypoxaemia has a synergistic effect on hypercapnoeic-ventilatory drive
- CO₂ is a potent pulmonary vasoconstrictor → ↑ pulmonary vascular resistance
- CVS
- Direct depression of cardiac muscle and vascular SM
- Peripheral vasodilation → ↓ SVR and ↑ regional BF
- ↓ heart rate/contractility
- ↑ RV Afterload due to effect on PVR
- ↑ circulating catecholamines
- Direct depression of cardiac muscle and vascular SM
- Neuro
- ↑ CBF (4% per mmHg ↑PaCO2) → ↑ ICP
- Haeme
- Right shift in OHDC
Severe (PaCO₂ >100mmHg)
- SNS stimulation
- hyperkinetic circulation →
- ↑ C.O., ↑ BP and arrhythmias
- ↑ cardiac MRO2 demand
- ↓ cardiac blood supply
- “CO2 nacrosis”
- PaCO2 > 80 mmHg → ↓ CSF pH
- Disorientation, confusion, headache, unconsciousness
- Hypoxia due to displacement of O2 from alveoli (as per Alveolar gas equation) → further ↑MV as above
- Respiratory acidosis →
- ↑ PaCO2 overwhelms buffering capacity of blood
- ↑ serum K+ and Ca2+ (stewart)
- ↑PaCO2 can cause anuria
Gladwin 2016
Examiner Comments
2012B 21: 5 (22.7%) of candidates passed.
Candidates were expected to present a mechanistic description the neuro-cellular events following a rise in PaCO2 such as changes in H+ in CSF, stimulation of central and peripheral chemoreceptors and neural pathways that lead to stimulation of respiratory centre. A systematic approach to the question with in-depth details of direction and magnitude of physiologic changes were required. Most candidates presented graphs of cerebral blood flow and tidal volume changes with increasing PaCO2. Common omissions included other important points, such as the cardiovascular and respiratory effects of rising CO2 and the rightward shift of oxygen haemoglobin curve.
iii. Explain the effect of changes in posture on ventilatory function.
iv. Define humidification, outline the mechanisms of humidification and its importance.
2012B 03
Outline the anatomy and physiology of humidification during normal breathing (50% of marks).
Describe the mechanisms of humidification used within Intensive Care practice (50% of marks).
2010B 13 – 2009B 19
Define the following terms (40%)
a. Saturated Vapour Pressure of Water b. Absolute Humidity c. Relative Humidity d. Latent heat of vaporisation.
Briefly outline how the humidity of air is altered during inspiration and expiration by the respiratory tract (60%)
CICMWrecks Answer
Humidity
Humidity
- Humidity is the concentration of water vapour present in the air.
Saturated Vapour Pressure of Water
- The water vapour pressure when the air is fully saturated.
- Depends on both pressure and Temperature
- = 47mmHg at STP
Absolute Humidity
- The amount of water vapour present in a given volume of gas (units g H2O/m3 or mgs H2O /L)
- Room air at sea level has Absolute humidity of 10g H2O/m3
- < 100% saturation
- Absolute humidity is temperature independent
- 100% saturation
- Absolute humidity is temperature dependent – due to ΔSVP fully saturated air
- at 0 °C contains 4.8 mg/L;
- at 20 °C contains 17 mg/L;
- at 37 °C contains 44 mg/L
- Absolute humidity is temperature dependent – due to ΔSVP fully saturated air
Relative Humidity
- the amount of water vapour present in the gas expressed as a percentage of the amount of water vapour that would be present if the gas were saturated with water vapour.
Latent Heat of Vaporisation
- the heat required to convert 1g of a substance from the liquid phase to the gaseous phase at a given temperature (expressed in Jg-1)
Humidification Process
INSPIRED AIR (During nose breathing)
- Air is warmed by the radiant heat from nasal blood supply.
- ↑ing temperature → ↑ SVP → ↑’s water carrying capacity
- Moisture evaporates from the epithelia → ↑ relative humidity of the inspired air to ~90%
- Mouth breathing reduces the relative humidity of inspired air to 60-70%
- At the lungs, it reaches the isothermic saturation boundary where it achieves BTPS (body temperature and pressure, saturated with water vapour) conditions.
- This usually occurs at the second generation of bronchi.
- Absolute Humidity @ Carina = 44 g H2O/m3
- Relative Humidity @ Carina = 100%
EXPIRED AIR
- Expired gas transfers heat back to the cooler trachea and nasal mucosa.
- As the saturated gas cools, it can hold less water vapour (its saturated water vapour pressure falls)
- Condensation occurs on the mucosal surfaces, where the liquid water is reabsorbed.
- Reabsorption reduces potential airway water losses from 300ml/day to ~150ml/day
- Tracheal temperature and humidity fall with an increase in respiratory rate (ie, the isothermic saturation boundary moves more away from the upper airway)
Complications of non-humidified air:
- Mucosal dehydration
- Altered ciliary function
- Inspissation of secretions
- Atelectasis and V/Q mismatching (if underlying lung disease)
- ↑ heat loss (5-10%) as the inspired gases are warmed and more H2O needs to be added as vapour
Humidification Mechanisms in ICU:
- Passive
- HME (Heat-Moisture Exchanger)
- Active
- Bubble Humidification
- Passover
- Heats water in chamber
- Evaporated water entrained by fresh gas
- Nebulisation
- Pressure and heat vapourises water
Gladwin 2016
Examiner Comments
2012B 03: 0 (0%) of candidates passed.
For a good answer candidates were expected to provide information on essential facts such as, normal inspired air has low water content, normal humidification provides for saturated water vapour 47mmHg at sea level at the alveolus, which corresponds to an absolute humidity of 44 g/m3 (or 100% relative humidity) at 37.C. Furthermore, candidates were expected to outline the basics of the anatomical features of the respiratory tract that promotes humidification e.g. lining of nose and hypopharynx dissolving warm water vapour into the dry inhaled air, the fact that the turbinates act to increase surface area and that full saturation is achieved by the time air reaches the upper trachea. For the second part of the question candidates were expected to mention, and briefly describe, the mechanisms of achieving humidification, e.g. bubble systems, heat-moister exchange filters, heated water, ultrasonic, etc. mechanisms. Candidates generally did not have sufficient knowledge of the basic conepts or a structured approach to this topic.
2010B 13: 3 (20%) of candidates passed this question.
This question was poorly answered by candidates. Basic aspects, which were critical for a good answer such as definitions were often inaccurate. Terms such as ‘amount’ or ‘content’ were commonly used without provision of units, when mass or pressure was required. The importance of temperature was often not mentioned. Most candidates identified the importance of the upper airway in humidification but did not describe details of this process and failed to discuss the events occurring during expiration.
Syllabus: R2c, S2e, B1k,2d
References: Davis, Basic Physics and Measurement in Anaesthesia, pgs 145-6, Nunn’s respiratory physiology pgs 12, 19, 166-7
2009B 19: 1 (11%) of candidates passed this question
It is essential that candidates read and respond to the question asked of them. The first part of the question required simple, accurate definitions, which the majority of candidates were unable to provide. Marks were awarded for the definition only. Descriptions of measurement, potential effects on oxygenation, etc. were not asked and gained no marks.
The core of the second part required an outline of the exchange of heat and moisture through the upper airways and bronchial tree, culminating in fully saturated gas at core body temperature by level of the 2nd generation bronchi. Whilst the question asked ‘humidity’ and not temperature, correct definitions in the first part would have dictated a joint outline of both. The effects of surface area, the nasal turbinates, mucosal secretion and blood flow were all relevant. The contribution to insensible moisture and heat loss should have been mentioned. No candidate considered the effects of respiratory rate, mouth versus nose breathing, or dry medical gases versus room air.
Syllabus – B1k2d
Reference: Nunn pages15 and 26
v. Explain the pathways and importance of the cough reflex.
2020B 08 – 2014A 13
Describe the cough reflex.
CICMWrecks Answer
Afferent
- In response to chemical, mechanical or noxious stimuli in larynx, trachea, carina or bronchi
- Signal transduced via internal laryngeal nerve (vagus nerve)
Central processor
- Medullary respiratory centre
- No single “cough centre” isolated
Efferent
Co-ordinated action of inspiratory muscles, pharyngeal muscles, and expiratory muscles
- Inspiratory phase
- Inhalation of a volume of air sufficient for cough generation (phrenic nerve and intercostal nerves)
- Compressive phase
- Closure of the glottis (recurrent laryngeal nerve)
- Activation of expiratory muscle against closed glottis
- Generation of transient increase in pressure of 300mmHg in thorax arterial blood and CSF
- Expulsive phase
- Opening of glottis due to posterio-cricoarytenoid muscle activation
- High velocity flow generated in bronchi, trachea and larynx
- “Choke point” – high velocity flow causes turbulant flow → increased shear stress between gas and airway lining → drag and expulsion of irritant
Intrinsic muscles of the larynx
- Cricothyroid
- Upper lip of cricoid cartilage → Inferior aspect of thyroid cartilage
- Innervated by superior laryngeal nerve
- Tenses vocal cords – elevates vocal tone
- Thyroarytenoid
- Anterior inner surface of thyroid à arytenoid cartilage
- Innervated by inferior laryngeal nerve
- Relaxes vocal cords – depresses vocal tone
- Posterior cricoarytenoid
- Posterior aspect of cricoid cartilage → arytenoid cartilage
- Innervated by inferior laryngeal nerve
- Abducts vocal cords
- Lateral cricoarytenoid
- Lateral aspect of cricoid cartilage → arytenoid cartilage
- Innervated by inferior laryngeal nerve
- Adducts vocal cords
- Transverse and Oblique arytenoids
- Spans the arytenoid cartilages
- Innervated by the inferior laryngeal nerve
- Adducts vocal cords
Sakurai 2016
Examiner Comments
2020B 08: 62% of candidates passed this question.
Overall, this question was reasonably well answered. Those that performed well had suitably detailed knowledge and structured their responses which generally included a definition and purpose of the reflex as well as the identification and a description of the afferent, integrator/controller, and efferent limbs of the reflex. This structure allowed a logical platform for the elucidation of the detail required in the answer, including types of stimulus, receptors, nerves (for both limbs of the reflex) and the muscles used in the phasic response to be clearly articulated
2014A 13: 26% of candidates passed this question.
Many candidates struggled with this question. A good answer included a brief description of the role of the cough reflex, an outline of the sensory pathways, central integration and motor pathways of the reflex, and a description of the components of the cough reflex. Most candidates failed to accurately describe the sequence of events involved in the cough reflex. Very few gave sufficient detail on the nerve supply of the larynx
vi. Outline the non-ventilatory functions of the lungs.
2013B 07
Outline the non-respiratory functions of the lung.
CICMWrecks Answer
Filter
- Narrow capillary bed
- Large particles prevented from returning to systemic circulation
- Blood clots → stroke
- Bacteria → killed by macrophages
Immunological
- Numerous macrophages present in lung
- Secretes IgA
Reservoir
9% of blood normally contained within pulmonary vasculature
- Sympathetic stimulation or straining (increase intrathoracic pressure
- Blood mobilised to systemic circulation
- Able to absorb blood volue
- Increase pulmonary artery pressure
- → Recruitment of pulmonary alveoli
- → Increased lung blood volume
- → Recruitment of pulmonary alveoli
- Increase pulmonary artery pressure
Metabolic
- α1-antitrypsin
- Removal of proteases
Endocrine
- Renin/angiotensin/aldosterone axis
- Lung is main source of ACE
- Angiotensin I —ACE—> angiotensin 2
Thermoregulation
- Humidification and warming on inspiration
- Cooling and condensation on expiration
- Prevents excessive loss of heat
Inhalational agents
- Route of administration for drugs e.g. bronchodilators
Sequestration of drugs (“taking up drugs”)
- Fentanyl has affinity for lungs
- Contributes to large VoD
Surfactant production
- Reduces alveolar surface tension
- Prevents alveolar collapse and overdistension
- From type 2 pneumocytes
Mnemonic: FIRM TITS
Mooney 2016
Examiner Comments
2013B 07: 5 candidates passed (18.5%).
In general, this question was not well answered. In particular candidates did not demonstrate sufficient depth of information. Nunn’s Applied Respiratory Physiology devotes an entire chapter to this topic (Ch. 12, in the 6th edition).
vii. Physiology at extremes of age, obesity, pregnancy (including foetal), altitude, and disease (particularly critical illness)
2020A 06
Outline how the respiratory system of a neonate differs from that of an adult.
2013A 07
Describe how the respiratory system of a newborn differs from that of an adult.
CICMWrecks Answer
Anatomical and Mechanical Differences
- Relatively larger head
- Prominent occiput
- Short neck
- Neonates do not have dentition
- Relatively larger tongue
- Higher larynx (C3-4 in infant, C4-5 in adult)
- Larynx lies more cephalad and anteriorly
- Epiglottis is narrower, omega shaped, more floppy and relatively larger
- The narrowest part of the airway is the subglottic area at the level of the cricoid cartilage
- Infants are obligatory nasal breathers until 3-5 months of age, the narrow nasal passages can easily be obstructed by secretions
- Shorter compressible trachea
- Increased risk of laryngospasm with inappropriate instrumentation.
- The smaller the patient the harder it is to position a LMA appropriately and maintain the correct position
- More compliant tissues and reduced muscle tone
- Main carina also lies more cephalad
- Small peripheral Airways (50% are < 2 mm diameter)
- ↓↓↓ bronchial muscle → so bronchospasms uncommon and bronchodilator drugs have minimal response
- Alveoli immature, and number <10% of adult total
- Reduced mucociliary clearance
- ↓ % type I muscle fibres (highly oxidative/slow contraction) in diaphragm (25% in neonate vs 55% in adults) and IC muscles (45% vs 65%) → ↑ risk of muscle fatigue
Lung Volumes
- FRC (30 mL/kg) → same as adult (as FRC remains 40% of TLC) BUT ↓ stable and ↑ risk of atelectasis due to following reasons:
- Significant ↓ in outward recoil of CW (as rib is cartilaginous and contains very little respiratory muscles)
- Mild ↓ inward recoil
- TLC (75-80 mL/kg) → same as adult
- VC (45 mL/kg) → lower cf. adult (60 mL/kg)
- TV same as adult (7 mL/kg)
- ↑ CC due to low elastic recoil of lungs → in fact, CC > FRC such that there is small AW closure during tidal ventilation → results in gas trapping and hypoxaemia
Lung Physiology
Variable | Neonate vs Adult |
Oxygen consumption | 6mL/min vs 3mL/min in adult |
O2 flux to tissues | ↑ due to: ↑ O2 carrying capacity(↑Hb, HbF, ↑MV → ↑pO2) ↑ Cardiac output |
Minute Ventilation | 2x adult (to cope with ↑MRO2) Generated by ↑↑RR → ↑ work of breathing |
Control of ventilation | Respiratory control less developed CO2 ventilatory response curve shifted to left O2/CO2 ventilatory response easily depressed by hypothermia Peripheral chemoreceptors immature Hering-Breuer reflex (transient apnoea lasting 5 secs evoked by gradual lung inflation) Head’s paradoxical inflation reflex (↑ inspiratory effort evoked by partial inflation of lungs) Periodic respirational (pauses lasting 5-10 sec (up to 6 x per hr during sleep) |
Chest Wall Compliance | Neonates have ↑ chest wall compliance relative to adult → Prone to collapse on inspiration → ↓ Tv for same respiratory effort → ↑ WOB |
Tissue Compliance | Initially neonates ↓ relative to adults until a few days of life when surfactant production is fully initiated, then → ↑ WOB in the first few days |
V/Q matching | Significant V/Q mismatch (V/Q = 0.4) due to shunting from gas trapping/small AW closure |
Physiological dead space | ↑ physiological dead space further necessitating ↑ MV |
Work of Breathing | ↑ WOB overall |
Gladwin / JC 2019
Examiner Comments
2020A 06: 20% of candidates passed this question.
This question required an outline of the anatomical, mechanical and functional differences. It was expected that factors leading to an increased work of breathing and oxygen cost would be mentioned. The mechanics of expiration were not often included in candidates’ answers. Immaturity of the alveoli and peripheral chemoreceptors were common omissions. Inaccuracies regarding upper airway anatomy and compliance of the chest wall cost some candidates marks. The question did not call for an explanation of the relative difficulty of intubation. Discussion of pathophysiology due to airway obstruction, causes of central apnoea or sensitivity to drugs was not required. Many answers included inaccurate information. Points which were often missed were difference in bronchial angles, number of alveoli, number of type 1 fibres in diaphragm, ciliary function and peripheral chemoreceptors.
2019A 19
Describe the effects of ageing on the respiratory system.
CICMWrecks Answer
Basic Changes
- Limited reserve
- ↓ Airway protective reflexes
- ↓ Respiratory Muscle Strength
- ↓ Maximal inspiratory pressure, Transdiaphragmatic pressure
- ↓ Maximum voluntary ventilation
- ↓ Elastic recoil of lung tissue (changes in the spatial arrangement and/or crosslinking of the elastic fibre network)
- Stiffening of chest wall and calcification of costal cartilages, ↓ in size of intervertebral spaces
- Loss of alveolar surface area and pulmonary capillary blood volume
- Compliance
- Chest wall compliance decreased (due to stiffening and structural changes)
- Lung compliance – normal or increased
- Total respiratory compliance normal or decreased
Static Respiratory Compliance (= Slope)
W = Chest wall
L = Lung
RS = Respiratory system
A – 20yr old man
B – 60 year old man
In elderly:
Compliance ↓ , RV & FRC ↑ , Static recoil pressure ↓
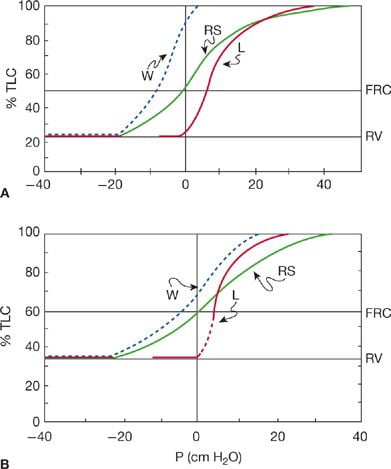
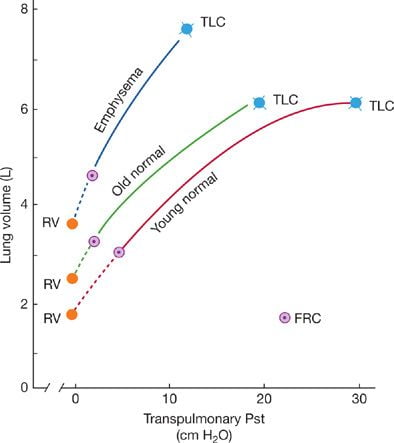
Static pressure–volume curves of the lungs.
Static recoil pressure (~transpulmonary pressure) plotted against lung volume
Lung Function
PFT:
- ↓ FEV1 (non-linear, accelerating in decline after 70 yrs)
- ↓ FVC
- TLC unchanged
- ↓ VC
- ↑ FRC (due to structural chest wall changes – kyphosis, increasing AP diameter)
- ↑ RV (Increased air trapping)
- ↑ CV/CC → airways close during normal TV (due to reduction in supporting tissues)
Gas Exchange:
- Anatomical dead space increases
- Ventilation-perfusion matching is increasingly mismatched
- Increase in units with low V/Q (dependent parts)
- pO2 falls ~ 0.3mmHg/yr
- Decreased Diffusion capacity
Others
Exercise capacity
- ↓ VO2 max
- ↑ Dead space ventilation
Immunology
- Bronchial fluid
- ↑ Neutrophils %
- ↑ Ratio of CD4+/CD8+ cells
- ↓ Epithelial lining fluid antioxidants
Regulation of Breathing
- Diminished Ventilatory response to hypoxia and hypercapnoea
- Increased oxygen cost of breathing for a given Minute ventilation
- Obstructive pattern develops even in lifetime non-smokers
Source: https://thoracickey.com/aging-of-the-respiratory-system/
JC 2019
Examiner Comments
2019A 19: 5% of candidates passed this question.
Answers should have included the effects of ageing on the efficiency of gas exchange, how the expected PaO2 changes with age, and its causation. Anatomical changes should have been included as should changes in lung volumes, particularly the significance of an increased closing volume. Marks were not awarded for the effects of disease states.
2008B 17
Describe the respiratory changes that occur in morbid obesity.
CICMWrecks Answer
Definition
BMI | As per LBW | |
---|---|---|
Normal | 15 – 25 | LBW |
Overweight | 25 – 30 | 110% – 119% LBW |
Obese | 30 – 35 | 120% – 199% LBW |
Morbidly Obese | 35 – 40 | >200% LBW (or) LBW + 50-100kg |
Super Morbidly Obese | > 40 | LBW + >100kg |
LBW(kg)
Adult males: Height (cm) – 100
Adult females: Height (cm) – 105
BMI = Weight (kg) / (Height(m))2
Respiratory Complications
- Lung Volumes
- ↓’d ERV (due to limitation of diaphragmatic excursion + Δ Compliance)
- +/- ↑’d residual volume (2nd to gas trapping or concurrent disease)
- Δ ERV is usually greater than any ↑ in RV thus
- ↓ FRC
- Can fall to < Closing capacity → right-to-left shunting + arterial hypoxemia
- ↓’d TLC
- ↓ FRC
- Lung Mechanics
- ↑’d work of breathing due to
- Altered pulmonary system compliance
- ↓’d lung compliance
- ↑ intrapulmonary blood volume
- ↓FRC to < Closing Capacity → Small airway narrowing/closure
- → ↑elastic work
- ↓’d chest wall compliance
- accumulation of fat tissue in and around the chest wall
- ↓’d lung compliance
- ↑’d airway resistance
- ↓ airway calibre
- Elevation of diaphragm during erect/head up positioning
- Altered pulmonary system compliance
- Result:
- shallow rapid pattern of breathing
- ↑ the work of breathing
- ↓’d respiratory muscle efficiency
- ↑ WOB → ↑RR → ↑ WOB → Capacity limitation
- ↑’d work of breathing due to
- Gas Exchange
- ↑’d metabolic activity of the excess fat + ↑’d workload on supportive tissues
- ↑’d O2 consumption
- ↑’d CO2 production
- ↑’d ventilation/perfusion abnormalities (especially supine/under anaesthesia)
- Limited maximum lung capacity (inability to increase ventilation in response to ↑’d demand)
- ↑’d sensitivity to respiratory depressant drugs
- ↑’d metabolic activity of the excess fat + ↑’d workload on supportive tissues
- Respiratory Control
- Obstructive sleep apnea
- defined arbitrarily: total cessation of airflow for 10 seconds or more despite continued respiratory efforts against an obstructed pharyngeal airway
- Hypopnea: a 50% reduction in airflow or a reduction sufficient to lead to >4% reduction in arterial oxygen saturation
- physiological responses to recurrent apnea
- hypoxemia and hypercapnia
- pulmonary and systemic vasoconstriction
- Secondary polycythemia and ↑ pulmonary vascular resistance
- Recurrent pulmonary vasoconstriction leads to right ventricular impairment.
- Obesity hypoventilation syndrome (chronic OSA)
- Loss of normal respiratory control during the day and daytime respiratory failure
- Altered chemoreceptor sensitivity to carbon dioxide.
- Reduction in respiratory drive leading to central apneic events (i.e. apnea without respiratory effort)
- Loss of normal respiratory control during the day and daytime respiratory failure
- Obstructive sleep apnea
- Airway Changes
- Fatty infiltration of the soft tissues of the neck →
- Difficulties maintaining airway
- Requirement for higher than normal BV mask pressures → gastric inssuflatio → ↑ aspiration risk
- Decreased FRC → ↓’d apoenic time on induction (compounded by ↑ O2 consumption)
- High risk of airway and intubation difficulties at induction of anesthesia
- Fatty infiltration of the soft tissues of the neck →
Gladwin 2016
Examiner Comments
2008B 17: 2 (40%) candidates passed this question
Obesity is an increasing problem in the broader community and in Intensive Care practice. Hence it is important candidates understand the physiological and pharmacological consequences of obesity. This question confined its scope towards obesity and the respiratory system. Major area of weakness of candidates was a lack of depth and breadth in knowledge of this topic and in applying basic physiology. A good answer required the following points –
Definition of morbid obesity (>200% ideal body weight or body mass index > 35)
Upper airway effects: fat infiltration of pharyngeal soft tissues difficult airway, prone to airway obstruction eg OSA
↑ O2 consumption and CO2 production: due to ↑ total body fat, requires ↑ cardiac output and ↑ alveolar ventilation
↓ FRC mainly via ↓ ERV: due to mass loading and splinting of diaphragm, upright obese,
closing capacity > FRC small airway closure, ↑ V/Q mismatch, ↑ venous admixture and
arterial hypoxaemia, ↓ O2 stores
↓ total respiratory system compliance: ↓ chest wall compliance , subcutaneous and intra
abdominal fat excess, ↓ lung compliance, ↑ airways resistance, ↑ work of breathing, ↓ resp
muscle efficiency
Altered ventilatory control: Obstructive sleep apnoea, Obesity hypoventilation syndrome
Syllabus: B1k B1d2k
Reference Text: Nunn’s Applied Respiratory Physiology / A B Lumb & J F Lunn – 6th ed
2023A 18
Explain the respiratory changes that occur at term in pregnancy.
2020A 18
Describe the respiratory changes that occur throughout pregnancy
2014A 04
Describe the respiratory changes during pregnancy.
CICMWrecks Answer
Respiratory Changes In Pregnancy
Anatomical
- Diaphragm ascends ~4cm due to foetus
- AP and lateral diameters of thorax increase 2~3cm due to relaxin effect on chest
wall - Thoracic circumference increases 5~7cm (AP and lateral diameters increased 2~3cm)
- Airway dilatation → decreased resistance (35%) and increased deadspace (45%)
- Increased cardiac output, intravascular volume, and decreased oncotic pressure
- Increased plasma volume → increased pulmonary blood volume → decreased compliance
- Venous engorgement → Upper airway oedema
Mechanical
- Lung volume changes occur from 20 weeks
- TLV decreases 5% from baseline
- FRC decreased 20% due to both ERV and RV
- Significant postural change in FRC of 70%
- Progesterone → increased sensitivity to CO2 → Minute ventilation increases 50%
- TV increases (25%)
- RR increases
- Results in hypocarbia and compensated respiratory alkalosis
- pCO2 ~ 26-32mmHg
- HCO3 ~ 18-20mmol/l
- Further increase in MV during contractions
- Inspiratory capacity increased by 10%
- Closing capacity unchanged
- Increased O2 consumption during labour (60%)
After birth
- FRC and RV return to normal within 48 hours
- TV returns to normal within 5 days
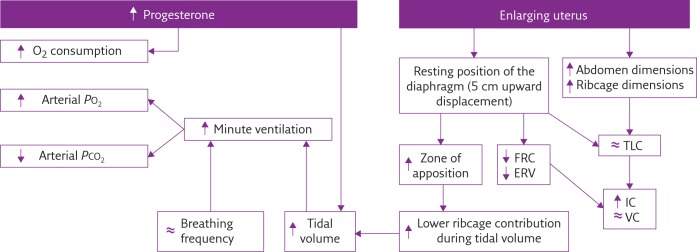
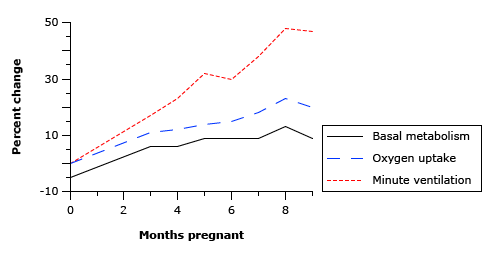
Sakurai 2016
Examiner Comments
2023A 18: 43% of candidates passed this question.
Good answers addressed the following in an ordered way: anatomic changes (including to airway, thoracic dimensions, dead space, airways resistance); what changes there are to respiratory volumes, capacities, and compliance; what happens to oxygen consumption and oxygen tension; and the acid-base changes that occur. Good answers didn’t just list these changes, but also provided an explanation for them. Few candidates mentioned the changes in oxygen tension and oxygen consumption, and why these occurred.
2020A 18: 31% of candidates passed this question.
The question asked for a description of the respiratory changes throughout pregnancy, which includes labour. Simple lists of changes did not score highly. A straightforward structure including; first, second and third trimester delineation would have elevated many answers from below par to a pass. Many good answers gave succinct detail on both mechanical respiratory changes and the hormonal mechanisms behind them. Higher scoring answers also described the overall effect of individual changes to spirometry, geometry or respiratory control.
2014A 04: 26% of candidates passed this question.
This question was variably answered, with a wide range of marks. Candidates often mentioned central regulation, anatomy, respiratory mechanics, static lung volumes or gas transfer, but did not provide comprehensive cover of all areas. Very few clearly described the extent of any change (either as a percentage or actual amount relative to the non-pregnant state). A number of candidates presented information well in diagrammatic form (e.g. static lung volumes) however then repeated this same information in text, which was unnecessary.
2009B 10
Describe the changes in inspired and alveolar oxygen partial pressure with increasing altitude. (20% of marks). Outline the respiratory physiological responses to altitude. (80% of marks)
CICMWrecks Answer
Changes in Inspired and Alveolar PO2
PAO₂ used as surrogate for PaO2
Alveolar PO2 can be described by the alveolar gas equation
Where
- FiO2 is the fraction of inspired O2
- 0.21 when breathing room air
- PATM is the atmospheric pressure which changes with altitude
- 760mmHg at sea-level
- 560mmHg in pressurized cabin of commercial aircraft (610mmHg in Boeing 787)
- Approx. 240mmHg at the summit of Everest
- SVPH2O is the saturated vapour pressure of water (47mmHg)
- ↓’s with ↑ing altitude → small ↑ in PAO₂
- PaCO2 is the arterial partial pressure of CO2
- Respiratory exchange ratio
- P is a correction factor
High Altitudes
- With ascension in altitude, PATM decreases therefore, PAO2 will decrease
- A-VO₂ gradient is lower
- Rate of O₂ dissolving in blood ↓’s at altitude → ↓ Diffusion by Fick
- PvO2 sits in the lower portion of the OHDC → ↓O₂ Affinity → ↑PaO2 to get same HbO2 carriage
- CaO2 rises much slower and may become diffusion limited with any exertion
Physiological Response to Altitude
Serum
- If there is no change in the diffusion of O2 PaO2 will similarly decrease proportionally
- Tissues will have a greater reliance on anaerobic metabolism, with an increase production of lactate, and decrease in pH.
Control of ventilation
- Peripheral chemoreceptors in the aortic bodies, sense changes in paO2, paCO2, [H+].
- Central chemoreceptors in the retrotrapezoidal nucleus (RTN) in the medulla sense changes in paCO2, once CO2 diffuses across the BBB and converts to H+ via carbonic anhydrase.
- Both signal to the medullary respiratory centre leading to an increase in ventilation (RR and tidal volume) via ventral group CPG
- There is an increase sensitivity of the respiratory centre to pO2 that is poorly understood
- Ventilation is more sensitive to changes in pCO2, usually maintained within 35~45mmHg.
- Response to hypoxia occurs once paO2 drops below 50~60mmHg and occurs in 3 stages
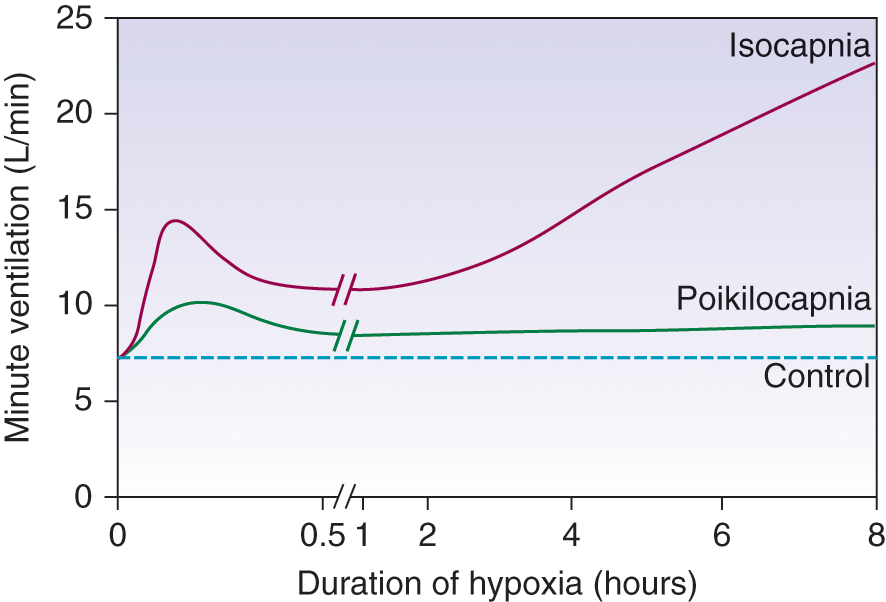
Three Phases:
- Acute Hypoxic Response
- ↑ altitude causes hypoxaemia
- ↓barometric pressure → ↓PiO2 → ↓PAO2 → ↓ PaO2
- ↓ PaO2 stimulates Peripheral Chemo Receptors (in CB and AB)
- ↑ MV significantly
- ↑ altitude causes hypoxaemia
- Hypoxic ventilatory decline (HVD)
- ↑ MV then ↓ PaCO2 causing
- ↓ brain ECF [H+] → ↓Central CR stimulation (in medulla) (→ response curve reset to left)
- limits any further ↑ MV due to hypoxaemia
- Ventilatory response to sustained hypoxia
- Brain ECF [H+] returns to normal (3 days)
- HCO3 rapidly equilibrates across the BBB
- hypoxic ventilatory drive is restored
- allows further ↑ MV to occur
↑ MV causes
- ↑venous return → ↑ CO → ↑ pulmonary Blood volume
- May ↑/↓ V/Q matching → ↑/↓ PaO₂
- ↓ pulmonary compliance → ↑WOB
- ↑ risk of diffusion limitation on exercise
Other Important Physiological Changes
- Hypoxia → ↑2,3DPG → ↓ HbO₂ affinity → ↑delivery
- Hypoxia Inducible Factors in the kidneys upregulate production of EPO, increasing O2 carriage.
- Alkalosis → ↓ammoniogesis → ↓HCO3 reabsorption → ↑ effective bicarb secretion which tends to return pH to normal
- High-Altitude Pulmonary Oedema may occur
- Poorly understood, however throught to be due, in part, to hypoxic pulmonary vasoconstriction
- This inhibits diffusion of O2 across the alveolar membrane, increasing the A-a gradient
Sakurai / Gladwin 2016
Examiner Comments
2009B 10: 0 (0%) of candidates passed this question.
Any description of the changes in inspired and alveolar PO2 with altitude required the description of, and an understanding of, the equations for calculation of PiO2
(PiO2=0.21(BMP-47)mmHg or 0.21(BMP-6.3)kPa) and PAO2 (PAO2 =PiO2 – PaCO2/R).
It was important to mention alteration in atmospheric pressure (BMP) with altitude and that saturated vapour pressure (SVP) is constant for the same body temperature.
A good answer to the second part of the question (respiratory physiological responses to altitude) was one that had some structure, eg responses divided into acute and chronic, respiratory, renal, haematological, cardiovascular and CNS categories, and relevant detail for each category. Candidates were expected to at least mention, but not restrict themselves to the following: acute responses, eg carotid/aortic body chemoreceptor stimulation hyperventilation, proportional to reduced BMP and hypoxia, limitations to hypocarbia (due to alterations in CSF pH) etc: physiological responses to early and chronic acclimatisation (eg renal HCO3-excretion, respiratory centre CO2 response curve resets to left, altered peripheral chemoreceptor sensitivity, 2,3, DPG levels and related right. Shift in HbO2 curve, alterations in pulmonary diffusing capacity due to increased alveolar surface area and pulmonary blood volume, etc).
It is important that current and future candidates use the examination reports to guide their learning. Thus it is essential that candidates not just read the feedback provided to each exam question but also refer to the reference(s) supplied below and other relevant sources they find to be useful.
Syllabus – B1k, 2i
Reference – Nunn’s Respiratory Physiology, Ch 17 and 5.
Power and Kam 432-4.
Recent Comments