Syllabus (Fourth Edition, 2023)
Topics
i. Describe the carriage of oxygen in blood.
ii. Explain the oxyhaemoglobin dissociation curve and factors that may alter it.
iii. Describe the carriage of carbon dioxide in blood.
iv. Explain the carbon dioxide dissociation curve.
v. Describe the oxygen and carbon dioxide stores in the body.
vi. Describe the physiology of foetal haemoglobin
Topics not covered in previous SAQs
iv. Explain the carbon dioxide dissociation curve.
v. Describe the oxygen and carbon dioxide stores in the body.
vi. Describe the physiology of foetal haemoglobin
Learning Objectives for the First Part Examination in Intensive Care Medicine
- This will ensure that trainees, tutors, and examiners can work from a common base.
- All examination questions are based around this Syllabus.
- These learning objectives are designed to outline the minimum level of understanding required for each topic.
- The accompanying texts are recommended on the basis that the material contained within them provides sufficient information for trainees to meet the learning objectives.
- Trainees are strongly encouraged to explore the existing and evolving body of knowledge of the Basic Sciences as they apply to Intensive Care Medicine by reading widely.
- For all sections of the syllabus an understanding of normal physiology and physiology at extremes of age, obesity, pregnancy (including foetal) and disease (particularly critical illness) is expected.
- Similarly, for pharmacology, trainees are expected to understand a drug’s pharmacology in these contexts.
- An understanding of potential toxicity and relevant antidotes is also expected.
Definitions
Throughout the document specific wording has been used under the required abilities to indicate the level of knowledge and understanding expected and a glossary of these terms is provided.
Definitions
Calculate | Work out or estimate using mathematical principles. |
Classify | Divide into categories; organise, arrange. |
Compare and contrast | Examine similarities and differences. |
Define | Give the precise meaning. |
Describe | Give a detailed account of. |
Explain | Make plain. |
Interpret | Explain the meaning or significance. |
Outline | Provide a summary of the important points. |
Relate | Show a connection between. |
Understand | Appreciate the details of; comprehend. |
SAQs
i. Describe the carriage of oxygen in blood.
2018A 01
Describe the carriage of oxygen in the blood, including total oxygen delivery per minute.
2016A 01
Outline the determinants of oxygen delivery to the tissues.
CICMWrecks Answer
O2
- Total body O2 content ~ 1.55L
- ~850mls – blood (20.4ml/100ml blood) (O2 Carriage)
- 20.1ml/100ml – bound to Hb
- 0.3ml/100ml – dissolved
- ~200ml – bound to myoglobin
- ~450ml in FRC
- ~50ml dissolved in tissue
- ~850mls – blood (20.4ml/100ml blood) (O2 Carriage)
- O2 Content
- O2 Delivery
- Determinants
- O2 Extraction / Extraction Ratio
Carriage of O2
- Normal arterial blood has a PO2 of 100mmHg and is 97.5% saturated
- Venous blood has a PO2 of 40mmHg and is 75% saturated
- O2 is carried either bound to Hb or dissolved in solution
- Dissolved O2 (2%)
- obeys Henry’s Law: amount dissolved ∝ the partial pressure.
- For each mmHg of PO2, there is 0.003ml O2/100ml blood
- therefore normal arterial blood with PO2 of 100mmHg contains 0.3ml O2/100ml
- Bound to Hb (98%)
- each gram of fully saturated Hb can bind 1.34ml of O2 (= Hufners constant)
- 98% O2 in blood = bound to Hb, a protein tetramer with an iron-porphyrin ring attached to each chain
- O2 coordinates with each Fe atom → inducing a conformational change → promotes binding of O2 to the other Fe atoms.
- total O2 binding capacity of Hb in blood (at normal p, temp, and PCO2) = 1.34ml/g → giving a total O2 carrying capacity of blood which an Hb of 150g/l of 20.1ml/100ml
O2 content
- 1.34 = Hufners constant at 37 degrees
- 0.03 = solubility coefficient for O2 in water
- O2 content per 100mL:
CaO2 (Arterial O2 content) | CvO2 (Venous O2 content) |
---|---|
= (15 x 1 x 1.34) + (0.003 x 100) | = (15 x 0.75 x 1.34) + (0.003 x 40) |
= 20.1 + 0.3 | = 15.08 + 0.12 |
= 20.4 ml | = 15.2 ml |
Relevant Anatomy (for O2 Carriage)
- RBCs
- small, flexible, biconcave discs
- cell membrane contains carbohydrate based antigens (ABO) and transmembrane proteins (Rhesus)
- No nucleus; no mitochondria (therefore aerobic metabolism not possible – entirely dependent on glucose and glycolytic pathway)
- Hb: large, ion containing protein contained within RBCs
- HbA
- Most common form of adult Hb (95%)
- Quaternary structure comprising 4 polypeptide globin subunits (2 alpha and 2 beta chains) in tetrahedral arrangement
- 4 globin chains held together with weak electrostatic forces
- each globin chain has its own haem group – an ion containing porphyrin ring with iron in the ferrous state (Fe2+)
- O2 molecules are reversibly bound to each haem group through a weak coordinate bond to the Fe ion
- In total: 4 O2 molecules can be bound to each Hb molecule – one for each heme group
- Different Hb types have different structures of globin chains
- HbA: 2 alpha, 2 beta (Adult)
- HbF: 2 alpha, 2 gamma (Foetal – replaced by HbA by 6/12 age)
- HbA2: 2 alpha, 2 delta (2-3% adults)
- Different O2 carriage and dissociation curves
- Disorders of Hb synthesis:
- Decreased production of normal globin change: Thalassaemia
- Abnormal globin chains: Sickle cell anaemia
- Affects O2 Carriage
O2 Delivery, Uptake
O2 Delivery and Uptake
- Normal O2 Delivery = 1000ml/min
- Determinents of tissue O2 delivery
- Diffusion from alveolus to blood: Fick’s law of diffusion and factors affecting rate
- Diffusion from capillaries into tissues and cells: partial pressure difference
- O2ER: O2 Extraction ratio = % of oxygen removed = O2 extraction / CaO2
Oxygen Delivery (DO2) | Oxygen Return | Oxygen uptake (VO2) |
---|---|---|
= CO x Art O2 content | = CO x Ven O2 content | = O2 delivery – O2 return |
= 5000 x 20 x 0.1 | = 5000 x 15.2 x 0.1 | = 1000 – 750 |
= 1000ml/min | = 750ml/min | = 250ml/min |
Determinants of O2 Delivery
- Cardiac output = HR x SV
- Pathological states like low output or hyperdynamic states can alter CO
- Oxygen content dependent on Hb, Sats, PaO2
- O2 delivery decreased in:
- decreased cardiac output (decreased preload, contractility, HR or increased afterload)
- decreased saturations (V/Q mismatch, decreased V, decreased PiO2, Shunt, increased CO2)
- decreased Hb (blood loss, iron deficient anaemia, anaemia of chronic disease, etc)
- O2 Uptake increased in:
- increased metabolic activity (sepsis, exercise, malignancy, pregnancy)
- right shift of HbO2 curve (CO2, ¯pH, 2,3 DPG)
HR
affected by automatic rhythmicity of cell and balance between sympathetic & parasympathetic systems
Stroke Volume
- = Amount of blood pumped into the circulation per contraction
- dependent on preload, contractility, afterload
- Preload
- ~ Venous return
- (MSFP-RAP)/RVR
- MSFP – Mean Systemic Filling Pressure
Equilibration of pressures in the systemic circulation if cardiac output was abolished
Describes the filling state of the circulation and tone of capacitance vessels - RAP – Right atrial pressure
- Resistance to venous return
- Calibre of transmitting venous system according to Poisuille-Hagen equation (where resistance ∝ 1/r4 )
- Physical obstructions to venous return: Mechanical obstruction, Valvular stenosis
- MSFP – Mean Systemic Filling Pressure
- Thoracic pump (negative intrathoracic pressure created during inspiration)
- Skeletal muscle pump
- One way valves
- Pump function of the ventricle
- Venous tone
- Skeletal muscle pump
- One way valves
- Pump function of the ventricle
- Venous tone
- Afterload
- Proportional to aortic pressure and ventricular size
- Inversely proportional to ventricular wall thickness
- Affected by obstruction to the LVOT
- Contractility
- Affected by adrenergic or muscarinic stimulation
- Availability of Ca
O2 diffusion into blood:
- Fick equation:
- Describes Diffusion through tissues
- Rate of movement of solute across semi-permiable membrane J is
where
C = concentration (or partial pressure for gasses)
A = cross-sectional area
T = thickness of the membrane or distance over which diffusion takes place.
- Thickness of membrane (normal: 0.3μm)
- Surface area of membrane (normal: 50-100m2)
- Age, posture, degree of inflation (1 mark for any)
- Partial pressure gradient across membrane
- Rate of blood flow through lungs
- (no points for mentioning diffusion constant of gas – question relates to only oxygen)
- Rate of oxygenation of reduced Hb
- Shift of oxygen dissociation curve (pH, temperature, PCO2, 2,3-DPG)
- Haematocrit
- Abnormalities of haemoglobin
Kerr / JC 2020
Examiner Comments
2018A 01: 32% of candidates passed this question.
Better answers divided oxygen carriage into that bound to haemoglobin and that carried in the dissolved form. A reasonable amount of detail on the haemoglobin structure and its binding of oxygen was expected, including an explanation of co-operative binding and the oxygen carrying capacity of haemoglobin. Better answers mentioned Henry’s law in the description of dissolved oxygen, along with an estimation of the amount of oxygen that is normally in the dissolved form.
It was expected that answers include the equation for oxygen delivery, a brief description of the components of that equation and the normal value, which a large number of candidates omitted.
2016A 01: 61% of candidates passed this question.
An opening statement such as oxygen delivery = cardiac output x oxygen content then allowed a more detailed description of the determinants of both oxygen content and cardiac output. It was expected candidates could detail the formula for Oxygen Content = Sat O2 % x 1.34 x Hb x 10 (depending on units) + PaO2 x .003, or x .03 (units) and accurately describe each element. Determinant of cardiac output completed the answer, often starting with CO = HR x SV while discussing the importance of preload, afterload and contractility. Many candidates spent little time on cardiac output which cost valuable marks. Some candidates provided a lot of detail on how oxygen is managed within the lung, the majority of which was not required as part of the answer. Some candidates answered the question describing the oxygen cascade only which was not sufficient to score well.
2012B 01
Compare and contrast the carriage of oxygen and carbon dioxide in blood.
CICMWrecks Answer
Carbon Dioxide
Total CO2 in arterial blood: 480ml/L
Total CO2 in venous blood: 520ml/L
Oxygen
CaO2 = (1.34 x [Hb] x SpO2) + 0.03 paO2
= 200ml/L
Approx 150ml/L in venous blood
Carried in 4 forms:
Carried in 2 forms:
Dissolved CO2 CO2 has 20 times solubility compared with O2 PaCO2 ~40mmHg PvCO2 ~46mmHg | Dissolved O2 Minor component According to Henry’s law: – Dissolved O2 proportional to partial pressure of O2 in gas phase. – Solubility coefficient 0.24 PaO2 ~100mmHg PvO2 ~45mmHg |
Carbamino compounds Minor component of CO2 carriage in blood, however 30% of the A-V difference in CO2 carriage Hb is major protein for CO2 carriage Reduced Hb has 3.5x CO2 binding capacity and this is the major factor for the Haldane Effect Haldane effect Increased CO2 carrying capacity in deoxygenated blood – Due to increased buffering capacity of reduced Hb | Haemoglobin bound O2 Major component of O2 carriage According to oxyhaemoglobin dissociation curve Curve altered by pH, pCO2 and 2,3 BPG – Right shift (decreased affinity) due to increased CO2 and 2,3 BPG – Left shift (increased affinity) due to increased pH – CO2 effect on Hb is the Bohr Effect: In presence of CO2, there is decreased oxygen carrying capacity in blood |
Bicarbonate Major component of CO2 carriage in blood | – |
Carbonic acid Negligible | – |
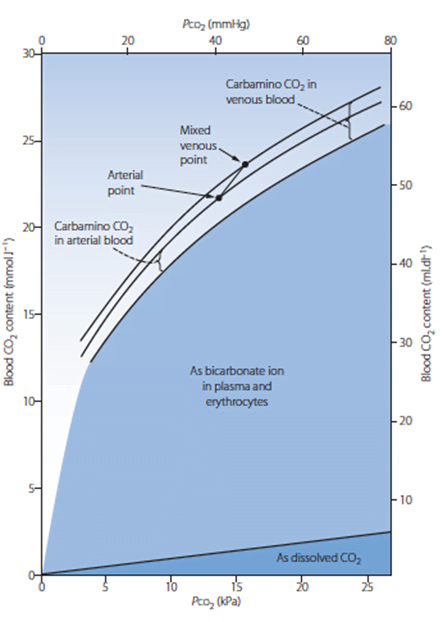
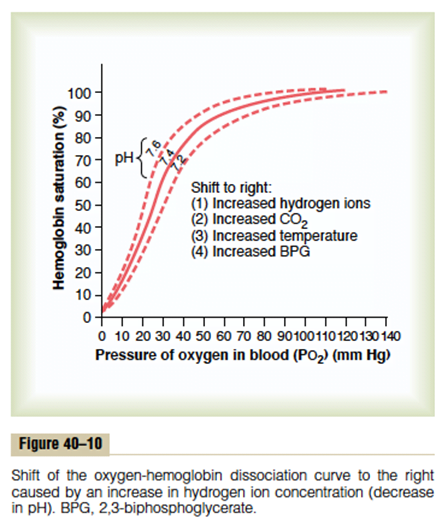
Sakurai 2016
Examiner Comments
2012B 01: 11 (50 %) of candidates passed.
Candidates who scored well for this question not only had a good knowledge of the topic but also displayed an organised approach to their answer through the use of a tabular format or some other structured approach. For a good answer, candidates were expected to provide information on the amount (both arterial and venous blood content, partial pressure) and form of carriage (binding to, loading and unloading from haemoglobin, dissolved, as bicarbonate, etc.) of oxygen and carbon dioxide in blood
ii. Explain the oxyhaemoglobin dissociation curve and factors that may alter it.
2015B 05
Explain the oxyhaemoglobin dissociation curve and the factors that may alter it.
CICMWrecks Answer
Oxyhaemoglobin dissociation curve
- OHDC describes the relationship between SaO2 and PaO2 at 37 degrees
- Demonstrates cooperative binding of Hb:
- ↑ in O2 affinity of Hb with each successive O2 binding
- 1st O2 molecule = difficult to bind – 2° strong electrostatic charges to be overcome to achieve conformational changes in Hb molecule: tense conformation → β chains far apart
- once 1st O2 has bound confirmation of Hb changes → β chains closer together → 2nd O2 has ↑ binding affinity → ↓ energy to bind
- 4th molecule binds 300 times more easily than 1st
- once 4th O2 bound → Hb in relaxed state
- The max amount of O2 that can be combined with Hb is the O2 capacity → all available binding sites are occupied by O2
- ↑ in O2 affinity of Hb with each successive O2 binding
- Importance of sigmoidal shape
- Upper portion is flat: if PO2 in alveolar gas ↓s slightly, loading of O2 will be little affected. I.e. a small ↓ in PO2 at normal O2 levels → causes only a slight ↓ in arterial saturation
- However, where O2 levels are already low, and on the steep part of the curve, the same small ↓ in PO2 will cause a sharp ↓ in SaO2
- Oxygen saturation of Hb = the % of the available binding sites that have O2 attached:
- (O2 combined wit Hb / O2 capacity) x 100
- In arterial blood, O2 sats are usually >97%. This corresponds to a PO2 of 100mmHg and is on the flat part of the curve
- In venous blood, the saturations are ~75%. This corresponds to the start of the steep part of the curve and a PO2 of ~40mmHg
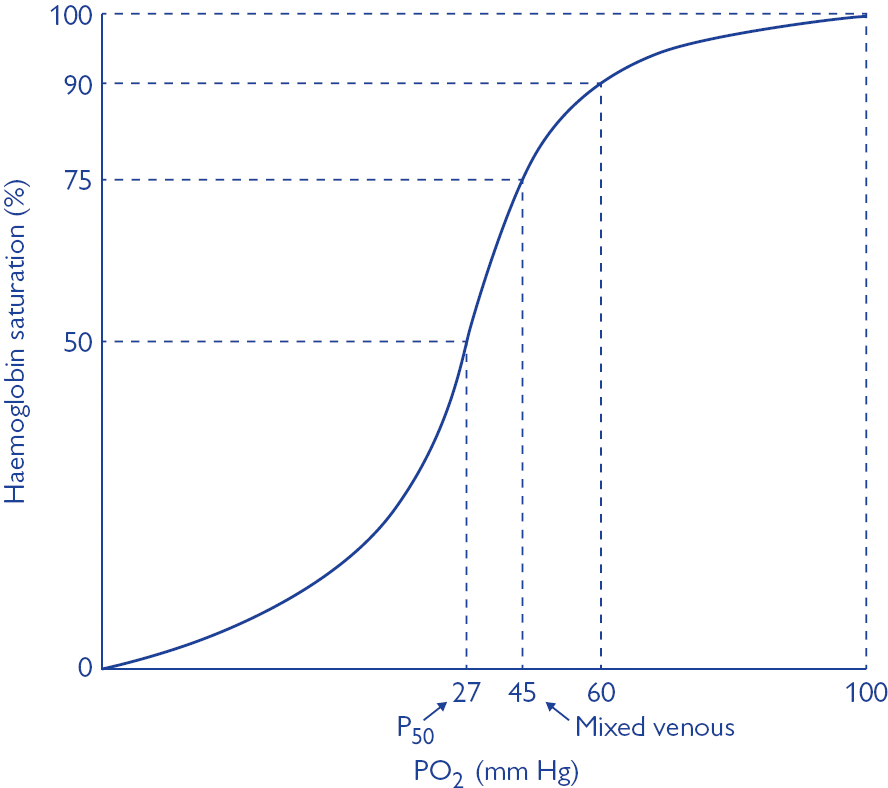
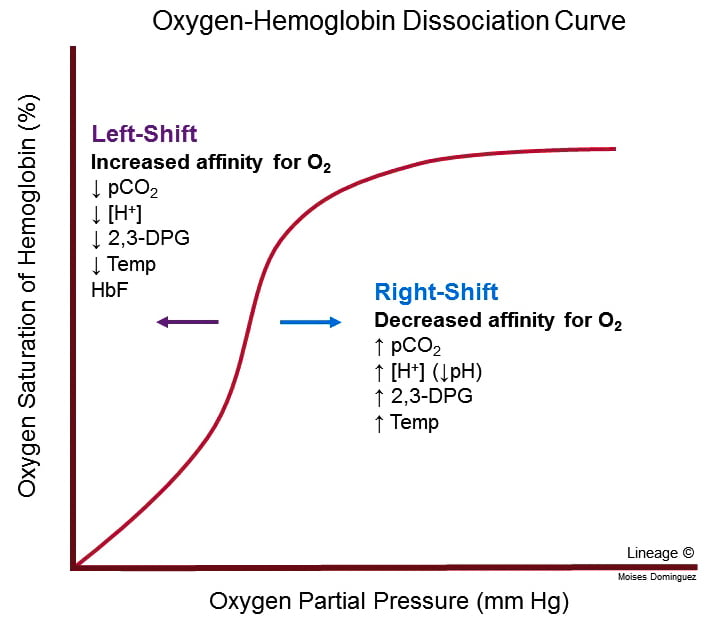
Factors that may alter the OHDC
- Position of OHDC is described by P50 value = the PO2 at which 50% of Hb is bound to O2; corresponds to PaO2 of 26mmHg
- Right shift
- ↓ affinity of O2 for Hb → O2 ↑ easily offloaded (i.e. for a given PO2, SaO2 is lower)
- ensures ↑ tissue oxygenation in states of ↓ perfusion
- Causes: ↑ PCO2; acidosis; ↑ 2, 3, DPG; exercise; ↑ temp; HbS
- Bohr effect: ∆ PCO2 and pH affect O2 transport → ↑ PaCO2 and ↓ pH sabilises deoxyHb → facilitates release of O2 → right shift
- Left shift
- Left shift → ↑ O2 binding affinity
- Causes; ↓PaCO2; alkalosis; ↓2, 3, DPG; hypothermia; methaemoglobin; HbF
2,3 DPG
- Formed by RBC during glycolysis
- Binds to beta chains of deoxy-Hb
- DPG binds strongly with beta chains → changing protein conformation → ↓O2 affinity
- ↑2,3DPG → ↑unloading of O2 from Hb → ↑ tissue supply
- Factors ↑ DPG:
- High altitude: aclimatisation response
- Anaemia
- Alkalosis
- Chronic hypoxaemia
- Exercise
- Pregnancy
- Hyperthyroidism
- Factors ↓DPG
- Stored blood: DPG ↓ in stored blood: storage ↓ glycolysis (only issue with MTP); levels return to normal after 24-48hr
Kerr
Examiner Comments
2015B 05: 77% of candidates passed this question.
Marks were awarded for an appropriate curve with values, an explanation of the nature of positive cooperatively and notes on those factors causing changes in the p50 or “shifts” in the curve.
Most candidates were able to provide the required sigmoid shaped curve with appropriate key value points (p50, venous and arterial points). Better candidates were able to identify p50 as a measure of avidity or affinity for oxygen and commented on T- Tense and R- Relaxed states, the role and production of 2,3 DPG , binding to the beta chains (nature of the lack effect on foetal haemoglobin). Describing the mechanisms associated with factors shifting the curve and commenting on changes in oxygen content over the steep and flatter parts of the curves gained additional marks. Candidates are reminded to answer the question asked – no marks were awarded for a description of dissolved oxygen delivery. Some answers confused the Bohr and Haldane effects.
iii. Describe the carriage of carbon dioxide in blood.
2020A 01 – 2018B 05 – 2015A 13 – 2012A 06
Describe the carriage of carbon dioxide (CO2) in blood /
Describe how carbon dioxide (CO2) is carried in the blood
CICMWrecks Answer
CO2
- End-product of aerobic metabolism
- Produced almost entirely in mitochondria
- Normal production approx. 200ml/min
- Total CO2 in blood
- Venous 52mL/dL
- Arterial 48mL/dL
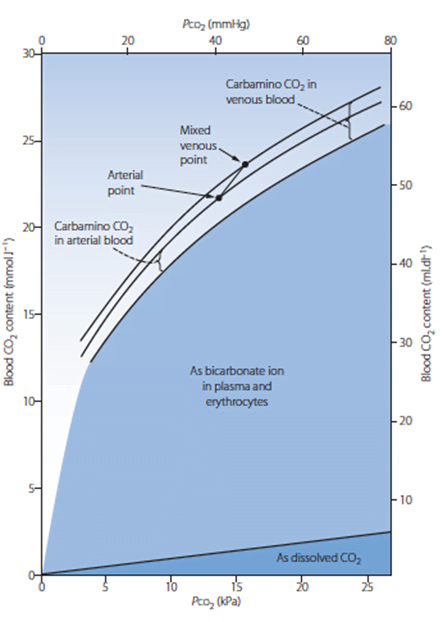
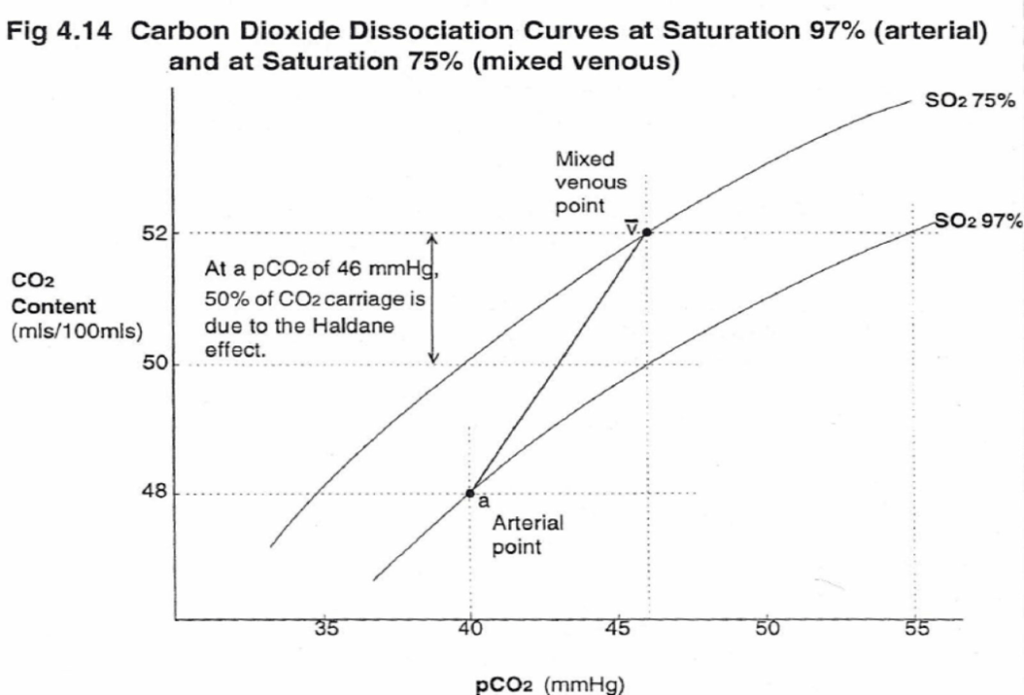
CO2 Carriage in 4 forms:
Arterial % | % of A-V diff | Details | |
---|---|---|---|
1. Bicarbonate | 90 | 60 | Main form of CO2 delivery in blood Via Hendersen-hasselbach: CO2 + H2O ⇔ H2CO3 ⇔ H+ + HCO3– Process 1. CO2 diffuses into RBCs 2. Carbonic anhydrase converts it to Bicarb 3. Hydrogen ions are buffered by binding to Hb molecules inside RBCs (30% Haldane.) Higher percentage in venous blood as: • Deoxygenated pKa 8.2 • Oxygenated pKa 6.6 • decreased pH = greater increase in [A-]/[HA] of DeoxyHb rel to OxyHb thus greater ability to accept protons 4. Bicarb is transferred out of cell in exchange for Cl ion by an exchange transporter called Band 3. (Hamburger Shift) |
2. Carbamino compounds | 5 | 30 | CO2 able to bind amino end of proteins Hb largely responsible as in much greater concentrations than any other protein (15 g/dL vs 7 g/dL) in serum CO2 combines with terminal amine to form carbamic acid dissosciates → at physiological pH to carbamate Affinity of Hb for CO2 increases in venous blood via Conformational change (70% Haldane): • Unbinding of O2 causes a conformational change in the beta chain • CO2 binding site at the N-terminus moves 1nm • Affinity for CO2 is increased. • Deoxy-Hb is 2-3x better at forming carbamino compounds than oxy-Hb |
3. Dissolved | 5 | 10 | CO2 ~20 x more soluble than O2 thus via Henry’s Law more CO2 is dissolved PvCO2 ~46mmHg (PaCO2 40mmHg) |
4. Carbonic Acid | <1% | <1% | Carried as dissolved H2CO3 Conversion to CO2 via Hendersen-Hasselbach equation above has pKa 6.1 At physiological pH Carbonic acid is ~96% dissociated Reaction is catalysed by Carbonic Anhydrase Not present in plasma, present in high concentrations in erythrocytes and pulmonary capilaries |
Gladwin / Sakurai 2016
Examiner Comments
2020A 01: 68% of candidates passed this question.
A detailed understanding of the carriage of carbon dioxide (CO2) in the blood is essential to the practice of intensive care medicine. Comprehensive answers classified and quantified the mechanisms of CO2 carriage in the blood and highlighted the differences between the arterial and venous systems. An explanation of the physiological principles surrounding these differences and the factors which may affect them was expected. The changes that occur at the alveolar and peripheral tissue interfaces with a similar explanation of process was also required. Candidate answers were often at the depth of knowledge required for an ‘outline question’ and a more detailed explanation was required to score well.
2018B 05: 65% of candidates passed this question.
A definition of arterial and venous CO2 content (mls and partial pressure) and an outline of the 3 forms of CO2 in the blood and their contribution to the AV difference, followed by a detailed explanation of each form of carriage was required for this question. A good answer included a table of the contribution of each form of carriage to arterial and venous content and the AV difference; explained the concepts of chloride shift when describing carriage as HCO3-; detailed the Haldane effect and its contribution to carbamino carriage and referenced Henry’s law when describing dissolved CO2.
West’s Chapter 6 on gas transport details the key information to score well on this question.
2015A 13: 79 % of candidates passed this question.
It was expected answers would describe each of the main categories of how CO2 is carried: Dissolved (10%), Plasma Bicarbonate (70%) and conjunction with plasma proteins and Hb as Carbamino Hb (20%). An opening statement quantifying the amount of CO2 dissolved in arterial (48mL/100mL) and venous blood (52mL/100mL) (4mL/100mL) and how this compares with Oxygen was expected (20 times more soluble).
For dissolved CO2, the application and description of Henry’s Law was awarded marks. A description of the consequences of the Haldane effect: difference in CO2 carriage of oxygenated and deoxygenated blood was expected. A diagram of pCO2 v CO2 content was helpful
2012A 06: 8 (80%) of candidates passed.
For a good answer candidates were expected to mention values for CO2 content in blood as well as the various ways it is carried (e.g. dissolved, as bicarbonate, combined with haemoglobin, etc) and a description of these modes. Wherever possible candidates are encouraged to illustrate their answer, in particular if those illustrations are core knowledge. Candidates who didn’t, were not penalised if they were still able to provide the required responses. However, candidates who did appeared to better synthesize a response. Candidates are reminded to include, and know, what are the appropriate units for any values they mention.
2012B 01
Compare and contrast the carriage of oxygen and carbon dioxide in blood.
CICMWrecks Answer
Carbon Dioxide
Total CO2 in arterial blood: 480ml/L
Total CO2 in venous blood: 520ml/L
Oxygen
CaO2 = (1.34 x [Hb] x SpO2) + 0.03 paO2
= 200ml/L
Approx 150ml/L in venous blood
Carried in 4 forms:
Carried in 2 forms:
Dissolved CO2 CO2 has 20 times solubility compared with O2 PaCO2 ~40mmHg PvCO2 ~46mmHg | Dissolved O2 Minor component According to Henry’s law: – Dissolved O2 proportional to partial pressure of O2 in gas phase. – Solubility coefficient 0.24 PaO2 ~100mmHg PvO2 ~45mmHg |
Carbamino compounds Minor component of CO2 carriage in blood, however 30% of the A-V difference in CO2 carriage Hb is major protein for CO2 carriage Reduced Hb has 3.5x CO2 binding capacity and this is the major factor for the Haldane Effect Haldane effect Increased CO2 carrying capacity in deoxygenated blood – Due to increased buffering capacity of reduced Hb | Haemoglobin bound O2 Major component of O2 carriage According to oxyhaemoglobin dissociation curve Curve altered by pH, pCO2 and 2,3 BPG – Right shift (decreased affinity) due to increased CO2 and 2,3 BPG – Left shift (increased affinity) due to increased pH – CO2 effect on Hb is the Bohr Effect: In presence of CO2, there is decreased oxygen carrying capacity in blood |
Bicarbonate Major component of CO2 carriage in blood | – |
Carbonic acid Negligible | – |
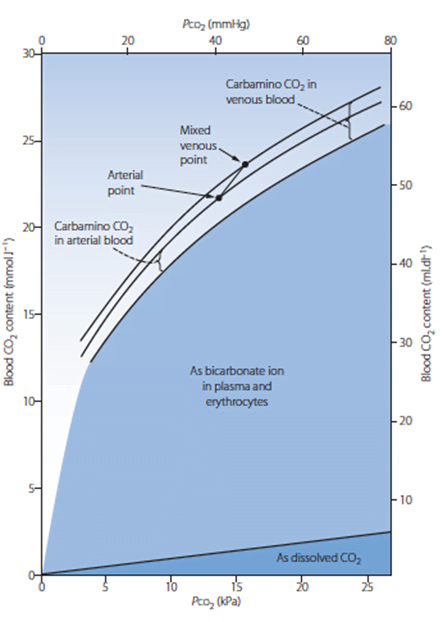
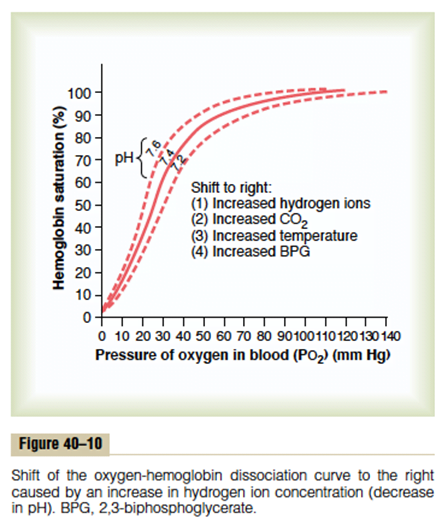
Sakurai 2016
Examiner Comments
2012B 01: 11 (50 %) of candidates passed.
Candidates who scored well for this question not only had a good knowledge of the topic but also displayed an organised approach to their answer through the use of a tabular format or some other structured approach. For a good answer, candidates were expected to provide information on the amount (both arterial and venous blood content, partial pressure) and form of carriage (binding to, loading and unloading from haemoglobin, dissolved, as bicarbonate, etc.) of oxygen and carbon dioxide in blood
2009A 02
Describe the production of carbon dioxide in the body (60% of marks).
What are the physiological reasons why the PaCO2 may be high? (40% of marks)
CICMWrecks Answer
PaCO₂ is determined by the balance of production and elimination.
CO2 production:
- Normally 200ml/min (compared to average oxygen intake of 250ml/min)
- Byproduct of aerobic metabolism
- Produced in the cell mitochondria via TCA
- Entry to TCA as acetyl CoA (from CHO, FA, aa)
- TCA products
- CO2
- Reduced compounds FADH and NADH
- H+ ions
- 2 molecules of CO₂produced for each 1 molecule of ATP
- Amount of CO2 produce per molecule of substrate varies with the substrate.
- 1.5 Mole CO₂ per mole of Glucose
- Respiratory quotient
- R = CO2 production / O2 consumption
- Depends on fuel substrate
- 0.7 for fat, 1.0 for CHO
- 0.8 for typical western diet
CO2 Storage and transport
- ~ 120 litres predominantly as bicarbonate ion
- CO2 is carried in the blood in 3 forms
- dissolved (5%)
- bicarbonate (70-80%)
- carbamino compounds (20-25%).
Elimination:
- Small amount dissolved in sweat
- Most via lungs
- PaCO₂ ~ PACO₂
- PACO₂ determined by alveolar ventilation equation
Factors which ↑PaCO₂
- ↑Shunts or ↑V/Q scatter → ↑PaCO₂
- ↑ Alveolar CO2 → ↑PaCO2
- ↑ Production (VCO2)
- During vigorous exercise
- Moderate exercise doesn’t effect pCO2 and may actually result in ↓pCO2 due to ↑MV
- Hypermetabolic states (Sepsis, Fever, MH, neurolept malignant syndrome)
- Altered (ie pure carb) diet
- ↑ Barometric pressure/↓Pressure of water
- ↑ Inspired CO₂
- Determined predominantly via alveolar ventilation which depends on:
- Dead space (DS):
- PE, low CO state, ↑d PEEP → ↑West’s zone
- ↑DS → ↓Alveolar Vent → ↑Alveolar CO2 → ↑PaCO2
- Respiratory Rate: ↓RR → ↓Alveolar Vent → ↑Alveolar CO2 → ↑PaCO2
- Tidal Volume (Tv): ↓Tv → ↓Alveolar Vent → ↑Alveolar CO2 → ↑PaCO2
- Dead space (DS):
- ↑ Production (VCO2)
Gladwin 2016
Examiner Comments
2009A 02: Pass rate: 10%
The main points for a pass include a brief description of the citric acid cycle and a list of facts such as storage of CO2 (120L), production (200ml/min) and that 2 molecules of CO2 are produced for 1 molecule ATP. A statement that PaCO2 is proportional to CO2 production/alveolar ventilation would help answer the second part. An example of increased CO2 production is fever, and of decreased alveolar ventilation is increased anatomical dead space.
Syllabus B1h 2e
References: Power and Kam 2nd edition p78-79,101, Stoelting and Hillier 4th edition p790-791, Nunn 6th edition p148-156
Recent Comments