1. (a) What are receptors (20% of marks)?
(b) Discuss the relationship between the properties of a drug and potential receptor response under the following headings (20% of marks each):
(i) Agonists
(ii) Partial agonists
(ii) Inverse agonists
(iv) Antagonists
CICMWrecks Answer
Receptors
- Receptor → protein-based moiety that contains a region that binds a natural ligand (or drug) to bring about a response
- They are located either:
- Within the cell membrane → ligand is poorly lipid-soluble (Ie. cannot cross cell
membrane) - Intracellularly → ligand is lipid-soluble (Ie. can cross cell membrane)
- Within the cell membrane → ligand is poorly lipid-soluble (Ie. cannot cross cell
- Drug-receptor binding can invoke 3 responses:
- Elicits an effect → agonist response
- Prevent the action of a natural ligand → antagonist response
- Reduce a constitutive effect of a receptor → inverse agonist response
- There are 5 classes of receptors:
- Ligand-gated ion channels
- G-protein coupled receptors (GPCR)
- Membrane guanylyl cyclase
- Tyrosine kinase receptors
- Intracellular receptors
Receptor | Structure / Mechanism | Class | Action | Example | ||
---|---|---|---|---|---|---|
Ion channel receptors (ionotropic) | Ligand-gated | – part of membrane-spanning complex of protein subunits that can form a channel through the membrane – generally found in excitable tissue | Ligand (or drug) binds to receptor to regulate ion flow through channel | Pentameric family | Possess 5x membrane-spanning units | nAChR – Na GABA-A receptor – Cl 5-HT3R glycine receptor |
Ionotropic glutamate | Glutamate is an excitatory NT in CNS that acts on various ligand-gated ion channels | NMDA receptor – Ca2+ AMPA, Kainate receptors – Na / K | ||||
Ionotropic purinergic receptors | ATP acts on PX1/PX2 receptors → involved in pain and mechano-sensation | PX1/PX2 receptors – Na,K,Ca – mechanosensation and pain | ||||
Voltage-gated | Have a common 4-subunit structure (each with 6 transmembrane segments) surrounding a central pore This pore is selective for the particular ion | Undergo a conformational change when the threshold potential is reached This is sensed by the S4 helix, which acts to open and close the channel | Na+ | Important in generating and transmitting an action potential by permitting sodium influx into cells | Located in myocytes and neurons | |
Ca+ | L | Muscular contraction | ||||
T | Cardiac pacemaker | |||||
N/P/Q | Neurotransmitter release | |||||
K+ | Located in myocytes and important in repolarisation following an action potential. | |||||
GPCR (metobotropic) | – Membrane bound protein with serpentine structure – associated with a heteromeric G-protein (α, β, γ subunits – α subunits binds either GTP or GDP) on the intracellular aspect of cell membrane | Ligand (or drug) binds to GPCR extracellularly → induces conformational change that activates G-protein, which triggers a cascade of intracellular signalling mechanisms – Inactive: GDP bound to α subunit, which is associated with a βγ-dimer – Active: G-protein activated by ligand-bound GPRC – GTP replaces GDP → activates or inhibits effortor protein: adenyl cyclase (AC) / phospholipase C (PLC) / ion channel – Intrinsic GTPase in α subunit hydrolyses GTP to GDP → regenerates an α-GDP subunit which reassociates with βγdimer → inactive | Gs | α-subunit activates AC → ↑ cAMP synthesis → cAMP binds protein kinase A (PKA) to produce cellular effect (Ie. ∆ gene transcription, ∆ ion permeability of membrane) | β1, β2, β3 receptors H2 receptor | |
Gi | α-subunit inhibits AC → ↓ cAMP synthesis → ↓ PKA activation → cellular effect | α2 receptor M2 and M4 receptors | ||||
Gq | α-subunit activates PLC → cleaves membrane phospholipid (phosphatidylinositol biphosphate; PIP2) into: – Inositol tri-phosphate (IP3) → causes Ca2+ release from ER – Diacylglycerol (DAG) → activates protein kinase C | α1 receptor M1, M3 and M5 receptors H1 receptor | ||||
Enzyme-linked receptors (Catalytic) | MONOMER: Membrane guanylyl cyclase | activated by a ligand and cause enzymatic activity on the intracellular side | Membrane-bound receptors a/w an intrinsic guanylyl cyclase | Ligand or drug binds to receptor → activates intrinsic guanylyl cyclase → ↑ cGMP → phosphorylation of IC enzymes → cellular effects | guanylyl cyclase (ANF receptor) Guanylyl cyclase for NA | |
DIMER: Tyrosine kinase receptors | activated by a ligand and cause enzymatic activity on the intracellular side | Transmembrane receptor-enzyme complex that consists of – extracellular ligand-binding domain (2x α-subunits) and – membrane-bound domain (2x β-subunits) associated with a cytoplasmic enzyme (Tyrosine kinase) | Ligand or drug binds to the α-subunits (extracellular domains) which causes the β-subunits (membrane-bound domains) to dimerise → IC tyrosine residues on β-subunits are then phosphorylated leading to activation of tyrosine kinase → this phosphorylates various IC proteins that elicits a cellular effect | Fibroblast GF receptor Insulin receptor | ||
Intracellular receptors | act as “ligand-regulated transcription factors”: – receptors reside within the cytoplasm → held in the inactive form by association with inhibitory proteins – Binding of hormone (or drug) induces conformational change that activates receptor (by releasing inhibitory protein) → receptor-ligand complex translocates to nucleus and associates with DNA promoter sequences → alter gene transcription | Ligands acting on these receptors are lipid soluble → cross cell membrane and bind to receptors within the cytoplasm | Steroid Receptor Superfamily which include steroids, sterols, thyroxine, retinoic acid and vitamin D. |
Drug – Receptor Response
Agonist:
Defined as a ligand or drug that binds to a receptor and alters the receptor state resulting in a biological response
Antagonist:
Defined as a ligand that binds to a receptor but does not activate it, instead blocking that receptor to a natural agonist
Efficacy:
- Defined as the ability of drug to elicit the maximal effect (EMAX) when bound to receptor
- Measured by height of plateau phase (or EMAX) in “log dose-response curve” → ↑ height of plateau phase (or EMAX) = ↑ efficacy
- It reflects “intrinsic activity” of the drug (Ie. magnitude of effect drug has once bound)
- Full agonists → 100% efficacy (or IA = 1)
- Partial agonists → efficacy b/t 0 and 100% (or 0 < IA < 1)
- Antagonists → 0% efficacy (or IA = 0)
- It is vital when selecting drugs (Ie. paracetamol and morphine are both analgesics but with different efficacy)
Potency:
- A comparative measure b/t drugs that have the same action on a receptor (Ie. have same log dose-response curve slopes) → refers to the different doses of two drugs needed to produce the same drug effect (Ie. more potent drug evokes a ↑ response at a ↓ dose)
- Measured by the EC50 in “log dose-response curve” → ↑ EC50 = ↓ potency
- It reflects the “affinity” of the drug for the receptor → ↑ receptor affinity = ↓ KD or ↑ KA = ↑ potency
- It is not as vital when selecting drugs (cf. efficacy), as long as the effective dose can be administered conveniently
Pharmacological effect of drug-receptor binding depends on:
- Properties of the drug:
- Affinity (Ie. how avidly a drug binds to a receptor)
→ determined by KA or KD of drug (such that ↑ KA or ↓ KD affinity - Intrinsic activity (IA; Ie. magnitude of effect drug has once bound)
→ drugs have IA b/t 0 and 1 (Nb. inverse agonists have IA b/t -1 and 0)
- Affinity (Ie. how avidly a drug binds to a receptor)
- State of receptor activation:
- Receptors exist in an equilibrium b/t “active form” and “inactive form”, which is altered by the presence of a drug
Receptor Affinity | Intrinsic Activity | State of receptor activation | Example | |
---|---|---|---|---|
Abscence of Drug | Equilibrium favours most receptors being in inactive form | |||
Full Agonist | High | Full (IA=1) | Drug binds receptors → shifts equilibrium towards ALL receptors being in “active form” | phenylephrine or noradrenaline at α1 receptor |
Partial Agonist | High | Fractional (0<IA<1) | Drug binds receptors → shifts equilibrium towards a FRACTION of receptors being in “active form” | buprenorphine at μ receptor |
Inverse Agonist | High | -ve (full or fractional) (-1=<IA<0) | Drug binds receptors → shifts equilibrium towards ALL or FRACTION of receptors being in “inactive form” | naloxone at μ receptor |
Antagonist | High | no (IA=0) | Drug binds to both receptor forms but does not alter equilibrium b/t “active” and “inactive” | Reversible, competitive: NDMR at nAChR Reversible, non-competitive: ketamine at NMDA receptor Irreversible: phenoxybenzamine at α receptor |
Examiner Comments
2024A 01: 28% of candidates passed this question.
A good answer provided a definition of a receptor as a protein/glycoprotein that undergoes a conformational change upon ligand binding. They then gave an overview of where these are found and the mechanisms of activation/downstream processing. A definition of each type (agonist, antagonist, partial agonist and inverse agonist) was expected with reference to the intrinsic activity at the receptor and the affinity for the receptor. A good answer also touched upon law of mass action (D+R=DR) and how this equilibrium is altered by each. Concepts such as reversible and irreversible binding and its implications as well as competitive and non-competitive antagonism would elevate the answer.
2. (a) Describe venous admixture and the sources contributing to it in an adult (60% of marks).
(b) Explain the effects of supplemental oxygen on arterial hypoxaemia (40% of marks).
CICMWrecks Answer: Venous Admixture
Venous admixture
“Theoretical volume of mixed venous blood added to pulmonary end-capillary blood to produce the observed CaO2 “
Causes of venous admixture
Shunt
- Physiological
- Thebesian vessels – drain venous blood from coronary circulation directly into cardiac chambers (including L ventricle)
- Bronchial circulation – Fracture of blood from bronchial circulation drains into pulmonary veins
- Pathological
Blood from pulmonary capillaries supplying unventilated alveoli (V/Q = 0)- Obstruction within bronchial tree
- Atelectasis
- Usually mitigated by hypoxic pulmonary vasoconstriction
V/Q mismatch
- Variation in ventilation and perfusion within the lung
- V/Q > 1 – Areas of lung with high relative ventilation to perfusion
- West Zone 1
- Lung apices
- In pathology
- Positive pressure ventilation
- Shock and hypoperfusion
- PE
- V/Q < 1 – Areas of lung with low relative ventilation to perfusion
- Lung bases
- West zone 4
- In pathology: Left heart failure, Pulmonary oedema
- V/Q > 1 – Areas of lung with high relative ventilation to perfusion
- Areas of high V/Q cannot compensate for areas of low V/Q due to the shape of the oxyHb dissociation curve and the sharp desaturation of deoxygenated blood. Also there may be decreased flow through the highly ventilated areas of lung
- V/Q mismatch in pathology
- Obstructive lung diseases: Asthma, COPD
- Restrictive lung diseases: ILD, ARDS
Sakurai 2016
CICMWrecks Answer: Effects of supplemental oxygen
Effects of Supplemental Oxygen
Cause of Hypoxemia | Mechanism | Effect of Supplemental Oxygen | Explanation |
---|---|---|---|
Ventilation-Perfusion (V/Q) Mismatch | Uneven distribution of ventilation relative to perfusion, with low V/Q areas contributing to hypoxemia (e.g., COPD, pulmonary embolism). | – Increases PAO₂ in well-ventilated areas, improving overall PaO₂. – Partial compensation for low V/Q areas. | Oxygen improves oxygenation in areas with some ventilation, as increased PAO₂ raises the oxygen gradient in regions with low but functional gas exchange. |
True Shunt | Blood bypasses ventilated alveoli entirely, leading to no gas exchange in shunted areas (e.g., ARDS, intracardiac right-to-left shunt). | – Minimal improvement in PaO₂, depending on shunt fraction size. – Limited effect in large shunts (>30%). | – Supplemental oxygen cannot oxygenate blood that bypasses ventilated alveoli. – Only oxygenating the non-shunted blood causes a modest rise in PaO₂. |
Diffusion Abnormalities | Impaired oxygen transfer across thickened alveolar-capillary membranes (e.g., pulmonary fibrosis, interstitial lung disease). | – Increases the oxygen gradient by raising PAO₂, improving oxygen diffusion. – Significant improvement seen unless limitation is extreme. | Higher PAO₂ compensates for the impaired transfer, as oxygen diffusion depends on the alveolar-arterial gradient. |
Low Inspired Oxygen (FiO₂) | Reduced oxygen concentration in inhaled air (e.g., high altitudes). | Fully corrects hypoxemia by increasing FiO₂ and raising PAO₂. | Supplemental oxygen raises PAO₂ to normal or higher levels, overcoming the reduced oxygen availability in the inspired air. |
Hypoventilation | Reduced alveolar ventilation decreases PAO₂ and causes a proportional drop in PaO₂ (e.g., opioid overdose, neuromuscular weakness). | – Corrects hypoxemia by increasing PAO₂ and PaO₂. – Does not address underlying hypercapnia or ventilation issues. | – Higher PAO₂ restores oxygenation but does not remove CO₂ buildup. – Treating the underlying cause of hypoventilation is still necessary. |
V/Q Scatter | Wide distribution of V/Q ratios due to heterogeneity in ventilation and perfusion (e.g., emphysema, interstitial lung disease). | – Improves PaO₂ by raising oxygenation in well-ventilated regions. – Cannot fully normalize due to persistent V/Q mismatch. | Supplemental oxygen increases PAO₂ in high-V/Q units, compensating for low-V/Q areas to some extent, but oxygen delivery remains uneven across the lung. |
Examiner Comments
2024A 02: 43% of candidates passed this question.
(a) This part of the question required candidates to provide a definition of venous admixture and provide detail on the sources. This would include anatomical (including atelectasis/closing capacity) and V/Q Mismatch or Scatter. V/Q Scatter was commonly omitted from answers. The use of the verb “describe” implies that further details are required beyond a simple list of the sources (such as a description of their mechanism and relative importance).
(b) This part required the effect supplemental oxygen on the many causes of arterial hypoxaemia to be explained. This would require the effect that this has on areas of true shunt and different degrees of shunt fraction and also on V/Q scatter. Information for this can be found on Nunn’s Respiratory Physiology.
3. The systemic vascular resistance is suddenly increased, describe the consequences for the otherwise healthy left ventricle.
CICMWrecks Answer
Afterload
- Load against which the muscle exerts its contractile force (Guyton)
- It is represented by the gradient of the line connecting the end-diastolic volume, to the end-systolic point
- pressure which the ventricle has to contract against (Power & Kam)
Determinants of Afterload
Modified Laplace Equation
- where
- T represents afterload
- P represents aortic pressure
- Therefore, afterload increases as aortic (or pulmonary arterial) pressure increases
- R represents ventricular radius
- Afterload increases as ventricular radius increases
- H represents ventricular thickness
- Afterload decreases as the thickness of the ventricular wall increases (hypertrophy secondary to chronic hypertension and cardiac remodelling)
Modified Hagen-Poiseuille Equation
- Afterload is affected by resistance to cardiac output
- Afterload increased by reduced radius of systemic vasculature
- Afterload increased by increasing viscosity of blood
Resistance in parallel
- Afterload is affected by addition, or loss of large capillary networks in parallel
- Systemic vascular resistance is increased significantly by loss of placenta, with parallel vascular networks
- Pulmonary vascular resistance is decreased significantly by inflation of lung, causing creation of vast capillary network in parallel
Cardiovascular Effects of a sudden increase in afterload
Effects of sudden increase in afterload can be demonstrated using a Left ventricular PV loop:
- End-Systolic Volume is increased, causing a reduction in stroke volume
- Initially, Left atrial pressure decreases
- Increased End systolic volume leads to secondary increase in end diastolic volume, hence increasing ventricular filling
- This secondary increase in preload enables the ventricle to contract with greater force (Frank-Starling mechanism) which partially offsets the reduction in stroke volume
- In patients with impaired left ventricular function, the decreased in stroke volume cannot be compensated
- ventricular end-systolic pressure: increases
- ventricular end-diastolic pressure: increases
- cardiac output (=HRxSV) – remains the same
- Initial drop in SV is compensated by increased pre-load
- In a failing heart, the drop in SV causes subsequent stimulation of baroceptors, which in turn causes increased heart rate and can potentially return cardiac output to normal
- Increase in afterload → Anrep → Small ↑Contractility to compensate
- Mechanism: ↑AL → sustained ↑stretch (prolonged isovolumetric contration) → ↑ Ca induced Ca release → ↑ contractility
- Purpose: ↑AL → ↓SV and ↑ ESV
- myocardial oxygen demand and myocardial work: Increased Afterload will increase the pressure during contraction, hence increasing MVO2 for internal work. This might be partially offset by the reduction in external work (due to decreased stroke volume) depending on the cause of the increased afterload
- coronary blood flow is autoregulated to remain normal
JC 2019
Examiner Comments
2024A 03: 11% of candidates passed this question.
This question was specific to the left ventricle only. Good answers provided information on immediate changes in LV Volumes and Pressures and how compensatory mechanisms largely within the LV itself eventually lead to restoration of stroke volume. Marks were allocated for a description of the Frank Starling mechanism, baroreceptor reflex and anrep effect. Good answers also discussed the effect of increased SVR on LV myocardial work and oxygen consumption and coronary perfusion. Common errors included focusing on definitions and determinants of SVR and providing LV PV loops without relating this to the situation of an increased SVR or without adequately labelling or explaining what they were demonstrating with the graph. This question required the integration of a number of physiological principles and is challenging.
4. (a) Describe the physical principles of haemodialysis and haemofiltration, including the factors affecting clearance (80% of marks).
(b) Outline the key components of renal replacement fluids (20% of marks).
CICMWrecks Answer
Physical Principles and factors affecting clearance
- Haemodialysis:
- Uses diffusion: “the movement of solutes from a high to a low solute concentration across a semipermeable membrane”
- Convection (see below) occurs during diffusion
- Blood is pumped through an extracorporeal circuit that contains a dialyser
- Dialysate flow is countercurrent, with maximizes the gradient for diffusion
- Solutes move across a membrane between blood and dialysate as per Fick’s Law
- Flow across the circuit is determined by:
- Set flow rate of centrifugal dialysis pump
- parts of the circuit:
- access port of vascular access (catheter / fistula / graft)
- from patient (pre-membrane)
- across membrane (trans-membrane)
- to patient (post-membrane)
- return port of vascular access (catheter / fistula / graft)
- Assuming that machine delivers the flow which is set and the flow remains laminar and constant, the pressure across the various components varies based on the resistance.
- The pressure gradient across the circuit depends upon:
- characteristics of patient:
- Blood viscosity
- hematocrit
- Blood protein and lipid content
- Temperature changes changes flow and viscosity
- Fåhræus–Lindqvist effect: Viscosity reduces in smaller diameter – because erythrocytes move over to the centre of the vessel/circuit, leaving only plasma near the wall of the vessel.
- variable resistance during breathing or movement
- collapsing vessels due to decreased vessel size / hypovolemia
- antivoagulation used
- Blood viscosity
- characteristics of vascular access:
- gauge and length of access
- resistance from physical obstruction
- resistance position
- resistance from clots, fibrin
- characetristics of circuit:
- Age of circuit, presence of clots, fibrin
- radius
- length (usually constant ~3.5m)
- surface coating of circuit
- anticoagulation used
- characteristics of membrane:
- resistance worsens with clot, fibrin, lipid deposition
- characteristics of patient:
- Uses diffusion: “the movement of solutes from a high to a low solute concentration across a semipermeable membrane”
- Haemofiltration:
- Uses ultrafiltration: “the movement of fluid through a membrane caused by a pressure gradient (hydrostatic or osmotic pressure)”
- Convection (see below) occurs during ultrafiltration
- Positive hydrostatic pressure in blood and a negative hydrostatic pressure in dialysate is generated → causing ultrafiltration and removal of solutes via solvent drag
- Elimination via bulk flow is independent of solute concentration gradients across the membrane
- Transport is dependent on starling forces
- The transmembrane pressure generated: blood flow to the membrane; oncotic pressure gradient
- Porosity of the membrane
- Additionally, a high filtration fraction will cause excessive haemoconcentration, and clotting of the filter
- The filtered fluid (ultrafiltrate) is discarded, and replaced with another fluid depending on the desired fluid balance
- Uses ultrafiltration: “the movement of fluid through a membrane caused by a pressure gradient (hydrostatic or osmotic pressure)”
Other Mechanisms involved:
- Osmosis:
- the movement of a pure solvent such as water, through a differentially permeable membrane, from a solution that has a lower solute (particle) concentration to one that has a higher solute concentration
- The rate of osmosis depends on the concentration of solute, the temperature of the solution, the electrical charge of the solute and the difference between the osmotic pressures exerted by the solutions.
- Movement across the membrane continues until the concentrations of the solutions equalise.
- Convection:
- The movement of solutes with a water-flow “solvent drag” across a membrane during osmosis or ultrafiltration
- Important for movement of small solute (urea, creatinine).
- Adsorption:
- when the molecules (solutes) adhere to the surface or interior of the membrane.
- with the movement of fluid across the membrane, if no fluid is moving then adsorption can not occur.
- in 2 manners:
- surface adsorption where the molecules are too large to permeate and migrate through the membrane; however they can adhere to the membrane.
- bulk adsorption occurs within the whole membrane where molecules can permeate it.
- Molecules that can be effectively adsorbed include:
- B2 microglobulin
- Cytokines
- Coagulation factors
- Anaphylatoxins
Renal Replacement Fluids
- Typically pre-packaged in 5L bags of sterile water
- contain sterile water, electrolytes and buffer
- Vary slightly in composition, but all are balanced salt solutions with either a lactate, bicarbonate or citrate buffer
- Bicarbonate-based solutions: most commonly used two-compartment systems. 25-35mmol/L and used as both dialysate and replacement fluids
- Lactate-based solutions: stable, cheaper, practical. But buffering capacity depends on conversion of lactate to bicarbonate. So not commonly used in CRRT
- Citrate-based solutions: new generation, administered prefilter. Advantage that additional anticoagulation is not required.
- Calcium-free solutions: When citrate-based fluids are used prefilter, these are used as dialysate and replacement fluids
- At present, there is no evidence to suggest that the choice of replacement fluid has an impact on survival or renal recovery.
- Replacement fluid can be added pre- or postfilter in varying ratios.
- The benefit of adding some of the replacement fluid prefilter is that it lowers the hematocrit of the blood, which reduces the likelihood of the filter clotting.
- The downside is that predilution reduces solute clearance and a compensatory increase in flow rates should be considered (15% for ultrafiltration rates of 2 L/h and up to 40% for rates of 4.5 L/h).
Composition (mmol/L) | PrismOcitrate 18/0 (Baxter) | PrismOcal (Baxter) | PrismOcal B22 (Baxter) | Prismasol (Baxter) | Hemosol B0 (Baxter) |
---|---|---|---|---|---|
Sodium | 140 | 140 | 140 | 140 | 140 |
Potassium | 0 | 0 | 4 | 0 | 0 |
Calcium | 0 | 0 | 0 | 1.75 | 1.75 |
Magnesium | 0 | 0.5 | 0.75 | 1 | 0.5 |
Chloride | 86 | 106 | 120 | 110 | 109.5 |
Bicarbonate | 0 | 32 | 22 | 32 | 32 |
Phosphate | 0 | 0 | 0 | 0 | 0 |
Citrate | 18 (=54mmol bicarb post metabolism) | – | – | – | – |
Lactate | 0 | 3 | 3 | – | 3 |
Osmolality (mOsmol/L) | 244 (280 post metabolism) | 282 | 282 | 287 | 287 |
Source: Mishra, Rajesh Chandra. ISCCM Manual Of RRT And ECMO In ICU A Reference Book For Practicing Intensivists (p. 25). Jaypee Brothers Medical Publishers.
Kerr’s notes
JC / Kerr 2022
Examiner Comments
2024A 04: 73% of candidates passed this question.
(a) Good answers divided this section into haemodialysis and haemofiltration and answered in two parts. Included information expected that candidates would cover the principles of haemodialysis, solute movement across a semipermeable membrane by diffusion and its dependency on the solute characteristics (size, charge, protein binding, volume of distribution) the dialysis membrane properties (porosity, thickness, surface area), the concentration gradient of substance in dialysate to blood and the rate of solute delivery (blood flow vs dialysate rate).
Haemofiltration, solute movement across semipermeable membrane by diffusion and required a discussion of the effect of transmembrane pressure, blood flow, effluent/ultrafiltration rate, plasma oncotic pressure,solute concentration in plasma water and the Sieving coefficient on the clearance.
(b) This part of the question required a brief representation of the substances found in renal replacement fluids including major electrolytes at near physiological concentrations (sodium, potassium, calcium, magnesium, and phosphate) and when these might be varied i.e. no calcium in citrate anticoagulation, less potassium in hyperkalaemia. Also the inclusion of a buffer (such as bicarbonate, lactate or citrate), water and the absence of colloid was also expected.
5. Outline the anatomy of the sympathetic nervous system including:
(i) The origin (15% of marks).
(ii) Fibre types and their course (70% of marks).
(iii) Receptors and neurotransmitters (15% of marks).
CICMWrecks Answer
Anatomy of SNS
- Posterior hypothalamus is the main site of sympathetic nervous outflow
- Receives input from cardiovascular centres of medulla and pons
- Sympathetic innervation from Sympathetic trunks
- Paired bundle of sympathetic neurons run lateral from vertebral bodies from T1 to L2
- Consists of two neurons in series
- Short Pre-ganglionic neuron → Sympathetic ganglion → Long Post-ganglionic neuron
Origin
- Origin: Preganglionic fibres originate in the grey matter of the spinal cord lateral horn
- Nerve Fibres and Pathway: Leave the spinal cord through ventral roots (with spinal nerves)
- Leave spinal nerves, travel as white rami communicantes (short myelinated B fibres)
- Meet with the ganglia of the sympathetic trunk
- Synapse with post-ganglionic neurons
- Neurotransmitter: Preganglionic neurotransmitter is acetylcholine
- Receptor: nicotinic receptors
Sympathetic trunk:
4 parts
- Cervical part
- Head
- Neck
- Thorax
- Thoracic part (T1-T5)
- Aortic plexus
- Pulmonary plexus
- Cardiac plexus
- Thoracic splanchnic nerves
- Lumbar sympathetic ganglia
- Coeliac plexus
- Pelvic part
- Hypogastric plexus
- Pelvic plexus
Post-ganglionic
- Nerve Fibres and Pathway: Post-ganglionic neurons leave the ganglia as grey rami communicantes
- Long unmyelinated C fibres
- Rejoin the spinal nerves to travel to target organs
- Neurotransmitter: Postganglionic neurotransmitter is noradrenaline
- (acetylcholine in muscles, sweat glands and hair follicles)
- Receptor: Adrenergic alpha and beta receptors on target organs/vessels
Exception is adrenal medullary outflow:
- Modified post-ganglionic cells release adrenaline into circulation
- Innervated directly by pre-ganglion sympathetic fibres
Mooney / JC 2020
Examiner Comments
2024A 05: 54% of candidates passed this question.
The question provided the headings that candidates would be expected to provide information on and those that used these headings to structure their answers provided good overall responses. A definition of the SNS and effects of activation was not required. Candidates were expected to outline SNS anatomy with respect to origin (pre-ganglionic neurons in the grey matter of the lateral horn of the spinal cord, T1 to L2), fibre types including a description of short pre-ganglionic myelinated b fibres, the sympathetic chain and the long post-ganglionic unmyelinated C fibres. Receptors and neurotransmitters included nicotinic Ach receptors in the ganglion and adrenergic alpha and beta receptors on target organs/vessels. A brief discussion of the arrangement at the adrenal medulla was also expected.
6. (a) Outline the process of fibrinolysis including how it interacts with the coagulation system (80% of marks).
(b) Describe the mechanism of action and adverse effects of alteplase (20% of marks).
CICMWrecks Answer: Fibrinolysis
Fibrinolysis
- Process by which fibrin clot is degraded to prevent excessive clot formation
- Plasminogen incorporated into clot
- Injured tissues and vascular endothelium gradually produce Tissue Plasminogen Activator (t-PA) in a delayed manner.
- Urokinase is produced by monocytes, macrophages and urinary epithelium
- Factor XII (Hageman factor) acts as a weak activator of plasminogen
- Plasminogen activated by t-PA, u-PA and factor XII to plasmin, a serine protease
- Plasmin cleaves fibrin into fibrin degradation products → reducing clot stability → Clot breakdown
Regulation of fibrinolysis
- Plasmin Activator Inhibitor (PAI) 1 & 2 – produced by vascular endothelium and inhibits tPA and uPA
- Protein C inactivates PAI, TAFI
- Thrombomodulin – expressed on in tact endothelium – binds thrombin and subsequently activates protein C
- Thrombin Activatable Fibrinolysis Inhibitor – produced by liver and cleaves plasmin binding sites on fibrin → decreased degradation
- α2 anti-plasmin – produced by liver, inhibits circulating plasmin, not fibrin bound plasmin
Sakurai 2016
CICMWrecks Answer: Pharmacology of Alteplase
Examiner Comments
2024A 06: 3% of candidates passed this question.
(a) This part required candidates to demonstrate their knowledge of the fibrinolytic pathway with details of the relevant mediators and inhibitors. Candidates where then required to apply their knowledge of both the coagulation cascade and fibrinolysis, with detail of how the pathways are simultaneously activated by the same stimuli to ensure balance between bleeding and clotting.
An example would be endothelial damage stimulating both the clotting cascade through collagen exposure and thromboplastin activation (faster response) as well as fibrinolysis through t-PA activation (slower response).
(b) This section of the question required a detailed description of the mechanism of action of alteplase, recombinant t-PA. A comparison to endogenous t-PA helped illustrate this action and the subsequent bleeding and the non-bleeding effects.
7. Outline the control of blood glucose.
CICMWrecks Answer
General
- Normal BSL 4-6mmol/L
- Tight BSL control important as:
- ↓ BSL: disrupt normal function of brain, retina, gonads (obligate glucose users)
- ↑ BSL: ↑ osmolality, osmotic load on kidneys → diuresis + cellular dehydration, loss of electolytes/ substrate → tissue damage
BSL control via feedback mechanism
- Sensors: pancreatic islets of Langerhans
- Central regulator: lateral (feeding) and ventromedial (satiety) centres of hypothalamus
- Effectors:
- Behavioural (feeding)
- Hormonal: insulin vs. glucoagon balance (act on liver, muscle, adipocytes)
- Renal (excretion)
- Modulated by: catecholamines, cortisol, thyroid homrones
- short term regulation: via secretion or inhibition of insulin + glucagon from pancreatic islets
- long term: neuronal mechanisms (SNS activation) + hormones (cortisol, GH)
Sensors
- Pancreatic beta cells → sense ↑ BSL
- Secrete insulin in biphasic pattern: initial rapid ↑ → prolonged slow ↑
- 1st phase of insulin secretion: ↑ BSL → glucose enters via GLUT2 → converted to pyruvate → enters TCA → generate ATP → inhibit ATP sensitive K channel → ↓ K efflux → depolarisation → open voltage gated Ca2+ channels → exocytosis of insulin granules
- 2nd phase of insulin secretion: glutamate produced as by-product of TCA → maturation of other insulin granules
- Pancreatic alpha cells → sense ↓ BSL
- ↑ glucagon release
Effectors
- insulin: secreted in response to ↑ BSL → following effects to ↓ BSL
- ↑ GLUT4 insertion into cell membrane → ↑ glucose uptake into cells esp. muscle + fat
- ↑ glycogen synthesis
- ↑ glucose utilisation + ↑ fat and protein synthesis
- ↓ glycogenolysis / ↓ gluconeogenesis
- glucagon: secreted in reponse to ↓BSL → following effects to ↑ BSL
- ↑ glycogenolysis / ↑ gluconeogenesis
- minimal effect on adipose tissue and muscle
- Adrenaline: stimulated by ↓ BSL, stress
- Inhibit insulin
- Liver: ↓ glycogenesis, ↑ glucose release, ↑ KB
- Fat: ↑ FFA release, ↓ glucose uptake
- Muscle: ↓ glucose uptake, ↑ FFA metabolism
- Sustained ↓ BSL stimulates GH + cortisol release
- ↓ glucose utilisation + ↑ fat utilisation → limiting further ↓ BSL
- ↓ protein synthesis / ↑ aa release / ↑ FFA metabolism
- Neuronal Mechanisms:
- Hypothalamus directly stimulated by hypoglycaemia → ↑ SYNS activity → adrenaline release → stimulates hepatic glucose release
Kerr 2016
Examiner Comments
2024A 07: 60% of candidates passed this question.
This question required candidates to discuss the role of the pancreas and liver in the homeostasis of blood glucose. This included how the pancreas senses high or low glucose levels, the mechanism of secretion of insulin and glucagon and subsequent effects on the liver. The response in early fasting when glucagon stores are depleted was expected as well as the role of other organs in this setting such as muscle glycogen and hypothalamus driven satiety. A brief mention other hormonal responses in the setting of hypoglycaemia was also expected (including ACTH, cortisol, adrenaline, growth hormone and thyroid hormones).
8. Describe the anatomy of the femoral vein as it relates to central venous cannulation.
CICMWrecks Answer
Femoral triangle (aka Scarpa’s triangle)
- The major boundaries can be recalled with the mnemonic SAIL:
- lateral border: medial border of sartorius
- medial border: medial border of adductor longus
- superior border: inguinal ligament
- floor: iliopsoas (laterally) and pectineus (medially)
- roof: skin, subcutaneous tissue, a continuation of Scarpa’s fascia, great saphenous vein (joins the femoral vein), superficial lymph nodes, fascia lata
- Contents (From lateral to medial):
- femoral nerve
- femoral sheath (thickening of the deep fascia of the thigh) which has three compartments (from lateral to medial):
- femoral artery and its branches (within the lateral compartment of the femoral sheath) and the femoral branch of the genitofemoral nerve
- femoral vein (within the intermediate compartment of the femoral sheath) and deep lymph nodes
- femoral canal (the medial compartment of the femoral sheath) which contains fat and lymph nodes (of Cloquet)
Femoral sheath
- Situated within the triangle.
- Formed from the fusion and invagination of the psoas and transversalis fascia.
- Contains femoral artery, vein and tributaries, and femoral canal
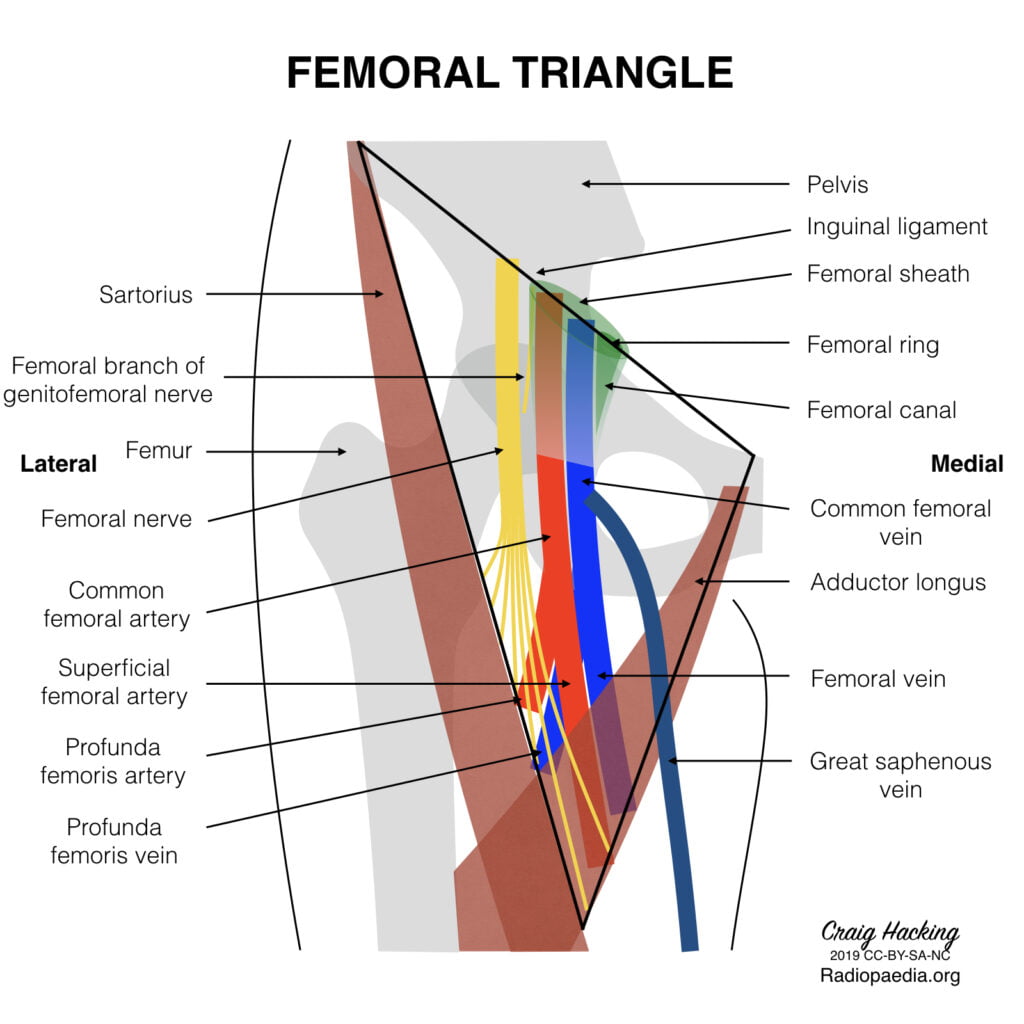
Femoral Vein
Main deep vein of the thigh and accompanies the superficial femoral artery and common femoral artery.
Course:
- Forms as the continuation of the popliteal vein at the adductor opening.
- Proximal to the confluence with the deep femoral vein, the femoral vein is commonly known as the common femoral vein. It becomes the external iliac vein as it ascends posterior to the inguinal ligament.
- In the distal adductor canal, the vein is posterolateral to the superficial femoral artery. Proximally in the canal, the vein lies posterior to the artery in the distal femoral triangle and medial to the artery at the base of the triangle.
- In the upper thigh, the vein is between the common femoral artery and femoral canal and therefore occupies the intermediate/middle compartment of the femoral sheath.
- Tributaries
- numerous muscular tributaries
- profunda femoris vein joins the femoral vein posteriorly 4-12 cm distal to the inguinal ligament
- the great saphenous vein enters anteriorly or anteromedially
- lateral and medial circumflex femoral veins are usually tributaries of the femoral vein
- Surface Anatomy:
- Palpate for the femoral pulse about 1 cm to 2 cm inferior to the inguinal ligament.
- The femoral artery is located at the midpoint between the anterior superior iliac spine and the pubic symphysis.
- With the femoral pulse identified, the femoral vein is about 0.5 cm to 1 cm medial to the pulsation.
Sources: Gray’s Anatomy, Radiopedia
JC 2019
Examiner Comments
2024A 08: 22% of candidates passed this question.
Anatomical questions are best answered with a standard structure that details the location; the boundaries (anterior/posterior, superior/inferior, medial/lateral); a description of the contents of that area and layers; and the relevant surface anatomy. For this question a description of the femoral triangle and boundaries, the origin and termination of femoral vein along with its tributaries, the relationship of the femoral vein to different structures in the triangle and information about femoral sheath and its contents was expected. Marks were also awarded for detailing the surface anatomy of femoral vein relevant to cannulation.
This was an anatomy question as such details of femoral vein cannulation technique, complications and the risks associated with femoral venous access was not required.
9. (a) Describe the pharmacokinetics of intravenous fentanyl and intravenous remifentanil (40% of marks).
(b) Explain how these features create contrasts between the two drugs (20% of marks).
(c) Discuss the concept of context sensitive half-time using these drugs as examples (40% of marks).
CICMWrecks Answer
PK and PD of Fentanyl and Remifentanil
Context sensitive half-time
- Defined as the time for plasma concentration to fall to half of its value at the time of stopping an infusion
- A method to describe the variability in plasma concentrations after ceasing an infusion
The “context” is the duration of infusion. - Used because terminal elimination half-life has little clinical utility for predicting drug offset
Half-lives are often misleading when discussing drug infusions. - Dependent on:
- Duration of infusion
During an infusion, drugs distribute out of plasma into tissues. When the infusion ceases, drug is cleared from plasma and tissue drug redistributes back into plasma.- The longer an infusion, the more drug has distributed out of tissues, and the longer the redistribution phase
- The longest context-sensitive half time occurs when an infusion is at steady-state
- Redistribution
The maximal CSHT reached depends on the:- VDss
Drugs with a larger VDss have a longer CSHT, as only a small proportion of the drug in the body will be in plasma and able to be cleared. - Rate constant for elimination
Drugs with a smaller rate constant for elimination have a longer CSHT.
- VDss
- Duration of infusion
Drugs with longer context-sensitive half-times will wear off less predictably.
Context sensitive half time – Fentanyl vs Remifentanil:
- Fentanyl shows variable CSHT – Has high redistribution, with VdSS 3-5L/kg, and a terminal half life of 3 hours. Hence, prolonged infusion may lead to increased duration of action and SE
- Remifentanil has little redistribution and a small Vd (0.2-0.3L/kg) in the peripheral compartments, and a very short elimination half life of 3 minutes. Hence, it has a very short, context-insensitive half time
It wears off reliably and quickly following cessation of infusion.

Courtesy: icuprimaryprep.com
Examiner Comments
2024A 09: 31% of candidates passed this question.
This question is essentially a compare and contrast question. It was worded specifically to encourage candidates to describe the pharmacokinetics of the drugs in question (in particular the relative lipid solubilities, volume of distribution, pka/ionisation, plasma protein binding, and metabolism) and then use these features to discuss the clinically relevant implications. In part (c) a brief definition of what is meant by the context sensitive half time followed by how these pharmacokinetic differences influence the CSHT of each was required.
10. (a) Explain the excitation contraction mechanism as it relates to the smooth muscle of the myometrium including a gravid uterus (50% of marks).
(b) For each tocolytic agent: salbutamol, nifedipine, magnesium sulphate, provide the following information:
(i) List the class (10% of marks)
(ii) Provide a dose (10% of marks)
(iii) Describe the mechanism of action (20% of marks)
(iv) Outline the adverse effects (10% of marks)
CICMWrecks Answer: Smooth Muscle in Uterus
Excitation Contraction Coupling in SM
- Motor neuron depolarisation
- ACh vesicular release
- Multiple MEPs → End plate potential
- Muscle AP propagates via T-tubles
- Opens L-type Ca channels
- Influx of EC Ca → ↑ IC [Ca]
- Variable amount of Sarcoplamic reticula in SM
- Can have some ↑ IC [Ca] via Ca induced Ca release.
- Ca binds Calmodulin → Ca-Calmodulin complex
- Ca-Calmod → ↑ MLCK activity
- → Phosphorylation of Myosin Light Chain (MLC)
- → Activation of Myosin ATPase
- Cross-bridge cycling.
Cross Bridge Cycling
- “Flexed” Myosin without ATP binds to Actin.
- Binding of ATP to Myosin causes release of Actin/Myosin complex and extension of the head
- In the presence of Ca-Calmod complex
- Actin binding sites are open and binding of myosin/Actin/ATP complex is formed
- Hydrolysis of ATP to ADP + P in myosin head by Myosin ATPase
- → conformational change “initial flexing” the myosin head and releasing phosphate
- Release of ADP further flexes Myosin head
- In presence of Ca return to step 2.
Relaxation
- Ca removed from cell
- Ca ATPase
- Ca2+/Na+ antiport
- Myosin Light Chain Phosphatase
- dephosphorylates MLC → inhibition of myosin ATPase
Differences in Gravid Uterus
These physiological adaptations in the gravid uterus maintain quiescence throughout most of pregnancy, ensuring fetal development, while preparing it to transition into a highly excitable and contractile state essential for effective labor.
Feature | Gravid Uterus | Other Smooth Muscle |
---|---|---|
Resting Membrane Potential | Less negative near term, increasing excitability | Stable, less variation in response to hormones |
Hormonal Regulation | Progesterone dominance (quiescence early), estrogen dominance (contractility late) | Task-specific, less pronounced |
Gap Junctions | Increased (connexin-43) near term for synchronized contractions | Sparse, localized contraction |
Calcium Handling | Increased L-type calcium channel activity and intracellular calcium recruitment near term | Stable calcium influx and release mechanisms |
Oxytocin Sensitivity | High near term, upregulated oxytocin receptor density | Minimal, plays minor or no role |
Relaxation Mechanisms | Progesterone/NO-mediated quiescence during pregnancy, reduced near term | Nitric oxide, prostaglandins, or sympathetic input |
Action Potentials | Long, frequent, propagating bursts near term | Short, localized |
Structural Adaptations | Hypertrophy and hyperplasia of myocytes | Minimal structural changes |
Functional Synchrony | Whole-organ synchronization with gap junctions, pacemaker cells, and oxytocin | Localized function, no whole-organ synchronization |
Gladwin / JC 2024
CICMWrecks Answer: Tocolytic Agents (Limited)
Examiner Comments
2024A 10: 42% of candidates passed this question.
(a) This question required an explanation of the process of contraction in smooth muscle, as well as specific information pertaining to the smooth muscle of the uterus. Specific information included: stimuli for contraction (i.e., hormones such as oxytocin, oestrogen, and prostaglandins and stretch); possessing an unstable membrane potential which plays a greater role than nervous input; as well as the uterus smooth muscle functioning as a syncytia and having baseline tone.
The key steps of contraction (influx of calcium, formation of calcium-calmodulin formation, activation of MLCK, creation of myosin-actin cross bridges with utilisation of ATP) are those generic to any smooth muscle and marks were awarded accordingly. Descriptions of striated muscle did not attract marks. A description or explanation was required rather than a list of steps (e.g., the sources of calcium rather than a simple statement of calcium influx occurring).
(b) Use of these drugs as tocolytics is acknowledged as a less common reason for their utilisation – the fractionation of this question was to breakdown into more achievable parcels of information. This allowed candidates to score more marks for relating the mechanism of action specifically to the uterus. Higher marks in this section were awarded for specific unwanted effects relevant to use as a tocolytic e.g., foetal tachycardia for salbutamol as result of crossing the placenta. Marks were awarded to doses for these drugs when used as a tocolytic, as opposed to for other indications (i.e., salbutamol infusion rather than nebulised).
11. Compare and contrast the carriage of oxygen in the blood with the carriage of carbon dioxide.
CICMWrecks Answer
Carbon Dioxide
Total CO2 in arterial blood: 480ml/L
Total CO2 in venous blood: 520ml/L
Oxygen
CaO2 = (1.34 x [Hb] x SpO2) + 0.03 paO2
= 200ml/L
Approx 150ml/L in venous blood
Carried in 4 forms:
Carried in 2 forms:
Dissolved CO2 CO2 has 20 times solubility compared with O2 PaCO2 ~40mmHg PvCO2 ~46mmHg | Dissolved O2 Minor component According to Henry’s law: – Dissolved O2 proportional to partial pressure of O2 in gas phase. – Solubility coefficient 0.24 PaO2 ~100mmHg PvO2 ~45mmHg |
Carbamino compounds Minor component of CO2 carriage in blood, however 30% of the A-V difference in CO2 carriage Hb is major protein for CO2 carriage Reduced Hb has 3.5x CO2 binding capacity and this is the major factor for the Haldane Effect Haldane effect Increased CO2 carrying capacity in deoxygenated blood – Due to increased buffering capacity of reduced Hb | Haemoglobin bound O2 Major component of O2 carriage According to oxyhaemoglobin dissociation curve Curve altered by pH, pCO2 and 2,3 BPG – Right shift (decreased affinity) due to increased CO2 and 2,3 BPG – Left shift (increased affinity) due to increased pH – CO2 effect on Hb is the Bohr Effect: In presence of CO2, there is decreased oxygen carrying capacity in blood |
Bicarbonate Major component of CO2 carriage in blood | – |
Carbonic acid Negligible | – |
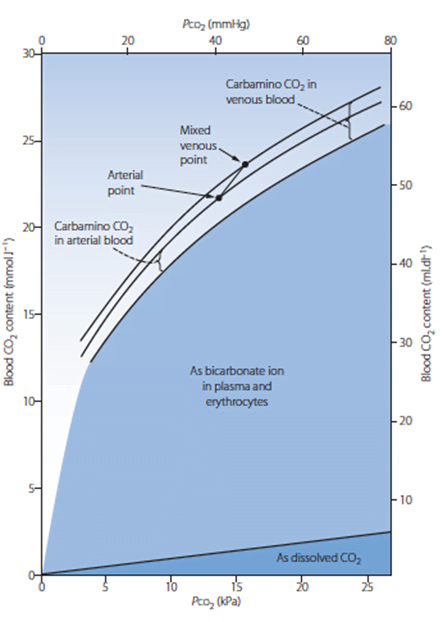
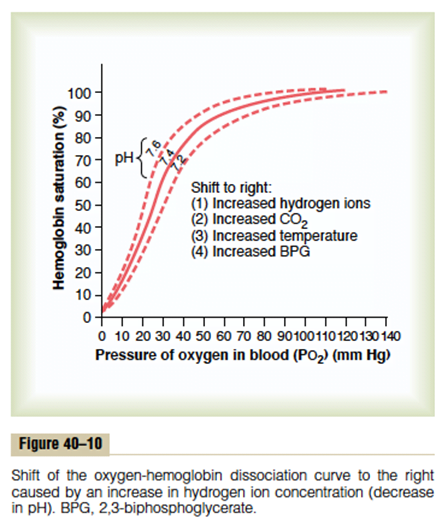
Sakurai 2016
Examiner Comments
2024A 11: 55% of candidates passed this question.
A good response included normal values of the partial pressures of both oxygen and carbon dioxide including the arterial and venous contents. Methods of carriage (haemoglobin, dissolved and other forms), haemoglobin binding characterisitcs (co-operative binding and affinities); and impact on loading/off loading at tissues and lungs for both oxygen and carbon dioxide was included. Correctly labelled diagrams with a brief accompanying explanation could be used to convey some of these concepts.
12. (a) Describe the action potential of the cardiac pacemaker cells including the ionic events (60%).
(b) Explain how excitation then propagates through the conducting pathway of the heart, including mechanisms to prevent abnormal conduction (40%).
CICMWrecks Answer
Normal Impulse Generation
Occurs in the sinoatrial node
- Innervated by (predominantly right) vagal nerve and sympathetic nerves
- Upslope of phase 4 due to “Funny” Na current (inward flow of Na)
- Parasympathetic (vagal) stimulation causes decrease (more negative) in the minima and shallower upward gradient of phase 4, delaying depolarization
- Sympathetic stimulation causes an increase (more positive) in the minima and steeper upward gradient of phase 4, hastening depolarization
- Action potential generated when threshold potential reached (~-50-55mV) → Opening of voltage gated Ca channels → Ca influx
- Cell begins repolarization when Ca channels close and K channels open à K efflux
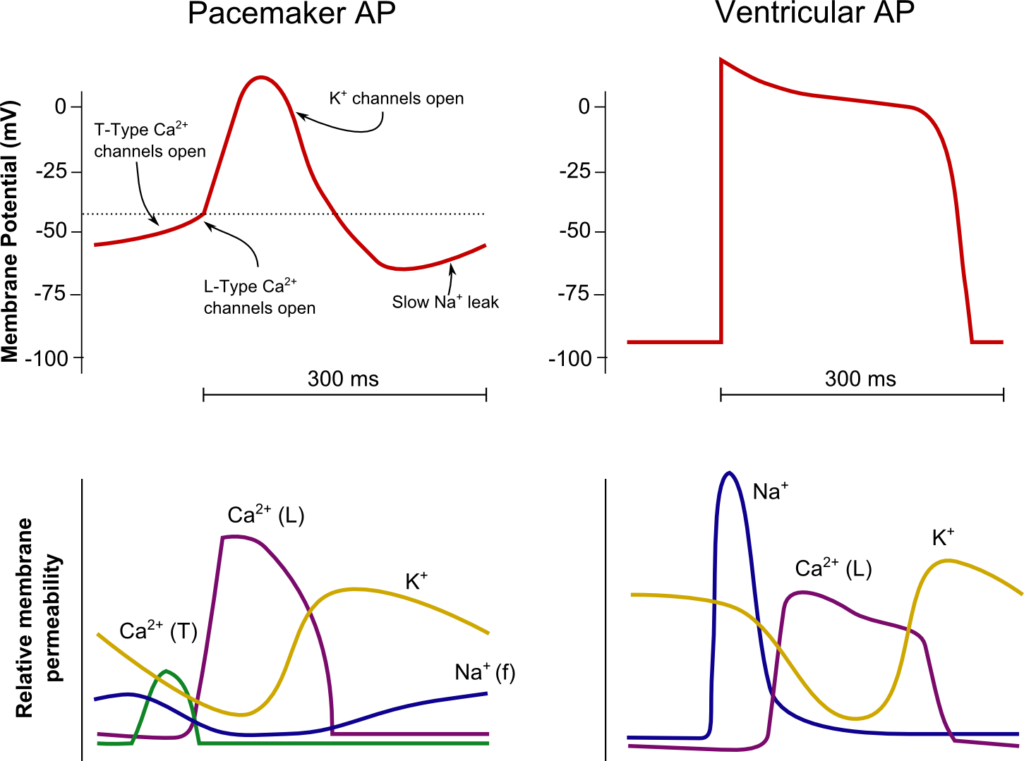
Normal Conduction
- SA node (5cm/sec) → Internodal fibres and Bachmann’s plexus (100cm/sec) → AV node (5cm/sec) → Bundle of His (100cm/sec) → Purkinje fibres (400cm/sec)
- Occurs due to fast wave action potentials (slow wave in AV node)
Ventricular AP:
- Phase 0 (Fast upstroke)
- Occurs due to opening of voltage gated Na channels (Na influx)
- Overshoot to +20mV
- Occurs due to opening of voltage gated Na channels (Na influx)
- Phase 1 (Early repolarization)
- Inactivation of Na channels and opening of K channels (K efflux) → Start of absolute refractory period
- Phase 2
- Opening of voltage gated Ca channels (Ca influx) balances K efflux
- Phase 3
- Closure of Ca channels → Repolarization
- Na channels transition to closed state (absolute refractory period transitions to relative refractory period)
- Phase 4
- Resting membrane potential (-80mV~-90mV) maintained by Na/K ATPase
Features which Prevent Arrhythmias / Conduction Abnormalities
Preventing the generation of arrhythmias
- SA nodal rate has faster intrinsic firing rate than other areas (~30bpm) causing
override inhibition of other pacemakers
Preventing the conduction of arrhythmias
- AV node prevents the conduction of impulses >220bpm
- Normal myocyte action potential has absolute (where no stimulus can initiate a new
action potential) and relative (where a greater than normal stimulus is required for initiation of a new action potential) refractory periods - Fibrous cardiac skeleton prevents conduction of action potentials other than through
the specialized conduction pathways
Sakurai 2016
Examiner Comments
2024A 12: 69% of candidates passed this question.
(a) A detailed description of the ionic events of the phases of the sino-atrial node AP was expected. A diagram of the sino-atrial node potential which included the phases and labelled x and y axis was helpful in this description. Some comparison with other cardiac action potentials added depth to answers, as did a brief description of the influences on the action potential such as the autonomic nervous system.
(b) This section required a detailed explanation of impulse propagation both between cardiac myocytes and across the myocardium. Safety mechanisms to be mentioned included anatomical insulation, refractory periods and rate.
13. Explain the counter-current mechanism in the kidney.
CICMWrecks Answer
Medullary concentrating gradient
- physiological process which sets up a concentration gradient from cortex through to medulla
- allows formation of concentrated urine
- Mechanisms:
- Counter current Multiplier: creates concentrated medullary interstitium
- Counter current Exchange (vasa recta): maintains intersisital osmotic gradient
- Recycling of urea: contributes to high osmolarity of medullary interstitium
- Normal cortico-medullary gradient 300-1400mOsmol.
Counter-current Mechanism in Kidney
Counter Current Multiplier:
Set-up
- Descending limb LoH Permeable to water only
- Ascending limb LoH Permeable to NaCl only
- Thin passive NaCl reabs
- Thick NaCl via active NKCCT cotransport and paracellular
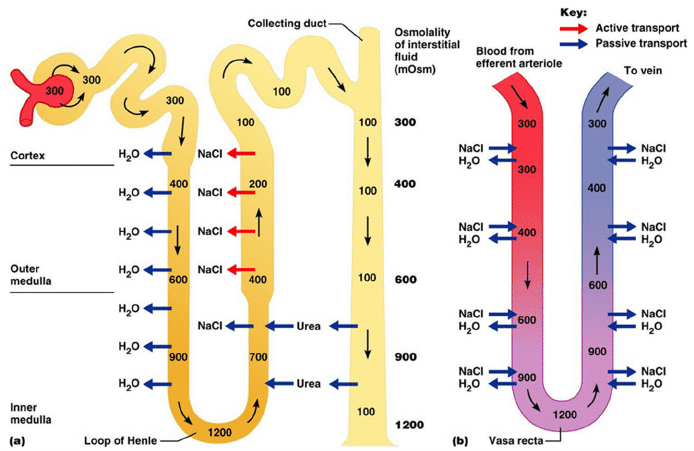
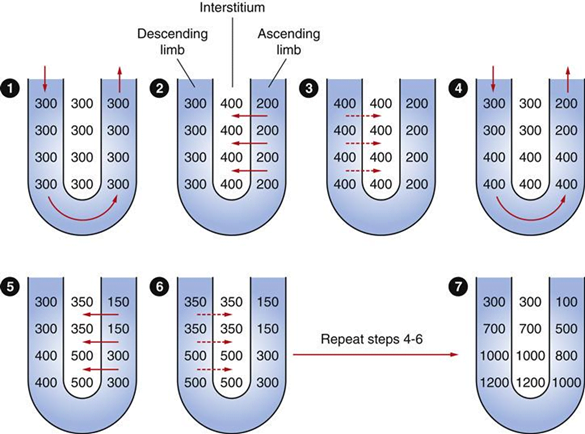
Generation
- Enters LoH osmolarity throughout = 300mOsm
- Max effort of NKCCT = 100mOsm/kg
- Tubular OSM = 200/kg
- Interstitial Osm = 400/kg
- Descending limb diffuses water down gradient
- Descend limb OSM now 400
- Process repeats (flow, pump salt, equilibrate water)
Counter Current Exchange:
- Hairpin loop arrangement of the vasa recta in the juxta-medullary nephrons.
- Provides blood flow to medullary tissue without impact of the cortico-medullary gradient.
- Relies on slow flow of blood.
- Mechanism
- On descent, water is lost from capillaries and NaCl is absorbed into capillaries increasing osmolarity
- On ascent water is reabsorbed and solute is lost
Role of Urea in Medullary Concentration Gradient
- 900mmol/day filtered
- 350-550mmol/day reabsorbed.
- Comprises 50% of osmolality (300-650 mOsm/kg)
- Freely filtered at the glomerulus
- Secreted by thin limbs of LoH down concentration gradient from medullary interstitium to tubular fluid.
- As water and Na are reabsorbed, tubular [Urea] increases
- [Urea] = >500mmol/L at collecting ducts
- Equilibrates with interstium due to slow flow rates.
- ↑ADH
- urea transporters UT1 and UT3 insertion → ↑ CD permeability to urea
- → ↑ medullary gradient (further 10% reabsorption if required)
Gladwin / JC 2020
Examiner Comments
2024A 13: 67% of candidates passed this question.
A good answer included an explanation of the purpose of the countercurrent mechanism followed by a detailed description of the functional and anatomical relevance of the Loop of Henle, vasa recta and urea recycling. A diagram if included required some explanation as it is not sufficient to completely replace text alone.
14. (a) List the normal parameters for cerebral blood flow (5% of marks).
(b) Describe the physiological factors that influence cerebral blood flow (95% of marks).
CICMWrecks Answer: Cerebral Blood Flow
Cerebral Blood Flow
- CBF = CPP / CVR (Cerebral Perfusion Pressure / Cerebral Vascular Resistance)
- CBF 15% resting CO → ~750ml/min or 50ml/100g brain tissue/min
- Gray Matter: 75–80 mL/100 g/min – Significantly higher due to the high metabolic activity and dense synaptic connections
- White Matter: 20–30 mL/100 g/min
- Abnormal CBF
- CBF<50ml/100g/min → cellular acidosis
- CBF<40ml/100g/min → impaired protein synthesis
- CBF <30ml/100g/min → cellular oedema
- CBF <20ml/100g/min → failure of cell membrane ion pumps, loss of transmembrane electrochemical gradients
- CBF <10ml/100g/min → cell death
Determinants of CBF
- CPP
- Net pressure gradient driving blood flow through the cerebral circulation
- CPP = MAP – ICP
- MAP = CO x SVR; CO = HR x SV; SVR = MAP / CO
- ICP dependent on: brain, blood, CSF
- CVR
Regulated by 4 primary factors:
- Cerebral metabolism
- flow-metabolism coupling:
↑ metabolic demand → ↑ CBF + substrate delivery - Controlled by vasoactive metabolic mediators:
H+ ions, K, CO2, adenosine, glycolytic intermediates, NO
- flow-metabolism coupling:
- CO2 and O2
- CO2
- At normotension: relationship between PaCO2 and CBF = almost linear
- ↑ PaCO2 → cerebral arteriolar vasodilation → ↓ CVR + ↑ CBF
- 2~4% increase for every 1mmHg increase in CO2
- ↓ PaCO2 → cerebral arteriolar vasoconstriction → ↑ CVR + ↓ CBF
- Initial stimulus = ↓ brain ECF pH
- Effects regulated by: NO, prostanoids, K channels, intracellular [Ca2+]
- PaO2
- little effect at normal PaO2
- PaO2 <60mmHg → cerebral arteriolar vasodilation → ↑ CBF
- Mechanism: hypoxia acts on →
- cerebral tissue to promote release of adenosine → cerebal vasodilation
- cerebrovascular smooth muscle → hyperpolarisation → ↓ Ca2+ uptake → vasodilation
- CO2
- Autoregulation
- Constant across CPP 50-150mmHg
- CPP >150mmHg: CBF µ CPP
- CPP <50mmHg: CBF <50ml/100g brain tissue/min → ischaemia
- Stimulus to autoregulation = CPP (not MAP)
- autoregulation curve R shifted in HTN; L in neonates
- Mechanism:
- Myogenic mechanism: arterioles vasoconstrict in response to ↑ wall tension + vasodilate in response to ↓ wall tension → ↓ or ↑ CVR
- May involve adenosine
- Can be impaired in SAH, tumour, stroke, head injury
- Constant across CPP 50-150mmHg
- Neurohumeral factors
- Relative lack of humoral + autonomic control on normal cerebrovascular tone
- Main action of SY nerves = vasoconstriction
- Other factors
- Blood viscosity: directly related to HCt; ↓ viscosity → ↑ CBF as per Hagen-Poiseuille law
- Temperature: ↓ CMRO2 by 7% for each ↓ 1°C in temp
- Drugs
- E.g. barbiturates ↓ cerebral metabolism
- Volatile agents → ↓ tension cerebral vascular smooth muscle → vasodilation + CBF
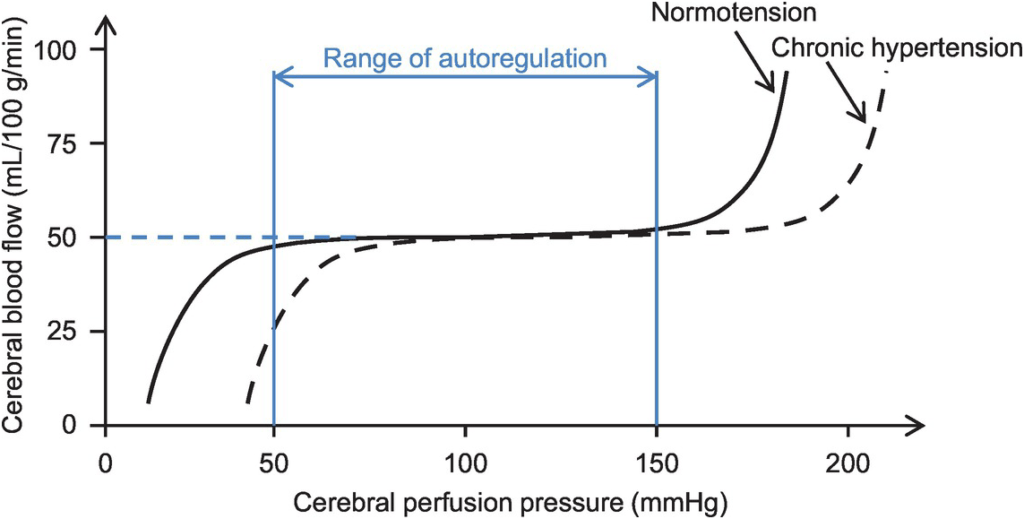
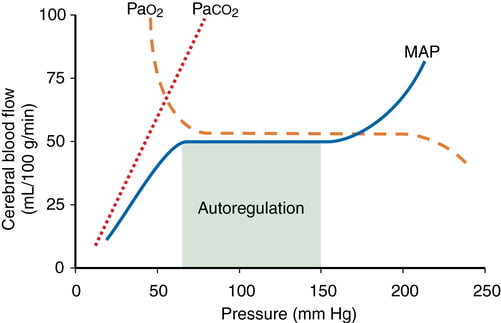
Kerr 2018
Examiner Comments
2024A 14: 62% of candidates passed this question.
The first part of this question required a list of the normal values for cerebral blood flow including differential flow to grey and white matter. For the second part, an answer structured around the cerebral perfusion/cerebral vascular resistance formula worked well to ensure breadth. Factors influencing flow include autoregulation of pressure (via the myogenic reflex), metabolic autoregulation and local acidity, arterial levels of CO2 and O2, temperature and a minor role for the sympathetic nervous system. Many answers were augmented effectively with graphs of cerebral blood flow versus CPP, PaO2 and PaCO2. A detailed discussed of ICP and factors effecting this was not required.
15. Outline how the measurement of the following can be used in the assessment of liver function (25% of marks each):
(i) Albumin
(ii) Prothrombin time (PT)
(iii) Glucose
(iv) Ammonia.
CICMWrecks Answer
Table of LFT values and clinical significance
(LIMITED)
Test | Reference Range | Clinical Significance |
---|---|---|
Serum proteins (including albumin, globulin, fibrinogen) | Total protein 60-80 g/L Albumin 35-45 g/L Globulin 25-35 g/L Fibrinogen 2-4 g/L | Liver synthesises nearly all plasma proteins (except Ig’s) |
↓ serum total protein, albumin, globulin and fibrinogen levels suggest impaired synthetic function due to hepatocellular damage | ||
Non-specific → also can suggest nephrotic syndrome, malnutrition states, burns, Etc. | ||
Note – Albumin is a long-term marker of liver function (as t ½ 20 days) → ↓ albumin means chronic liver dysfunction (as it can be normal with acute liver disease) | ||
Coagulation studies (INR and PT) | INR >1.2 PT 10-15 s | Hepatocytes synthesise clotting factors (incl vitamin K-dependent CFs) |
↑ PT or INR suggests either (i) impaired CF synthesis (hepatocellular damage → acute liver dysfunction), or (ii) vitamin K malabsorption (cholestasis) | ||
Non-specific → also can suggest warfarin use, DIC, Etc. | ||
Plasma glucose | BGL 4-8 mmol/L | Liver has vital role in glucostat function (glycolysis, glycogen metabolism, gluconeogenesis) |
BGL derangements suggest hepatocellular dysfunction | ||
Non-specific → also can suggest DM, insulin use, Etc. | ||
Plasma ammonia/urea | NH3 < 35 umol/L Urea 3-7 umol/L | Liver converts toxic NH3 (metabolite of a.a. and pyrimidine) into less toxic urea for renal excretion via the “urea cycle” |
↑ NH3 and ↓ urea suggests hepatocellular damage |
Bianca 2020
Examiner Comments
2024A 15: 56% of candidates passed this question.
A good answer should cover the following points: normal range, how and why the value is affected by liver failure and potential con-founders. As an example in the case of albumin; the normal range is 35-50g/L; it is produced in the liver and thus the measured value will decrease in synthetic liver dysfunction; the half life of albumin is long (20 days) and therefore it is a better marker of chronic dysfunction; potential con-founders include inflammation (negative phase protein), malnutrition and increased loss (nephrotic syndrome etc). Marks were not allocated for information not relevant to the question asked (such as the function of albumin).
16. (a) Define heat and temperature (15% of marks).
(b) Describe how body temperature is regulated (85% of marks).
CICMWrecks Answer: Definitions
Temperature
- A measure of a physical property of a substance that determines the tendency for heat to flow from one object to another → heat energy is transferred from a region of higher temperature to a region of lower temperature
- Units for temperature:
- SI unit → Kelvin (K), which is 1/273.16 of absolute temperature of “triple point of H2O” (where H2O exists in equilibrium as a solid, liquid and gas → occurs at 0.01°C)
- Non-SI unit → Celsius (°C), where 1°C is 1/100th the difference between the
freezing and boiling point of H2O
Heat
- A form of kinetic energy → being a state of “thermal agitation” of molecules in a substance
- Units for heat:
- SI unit → Joules (J)
- Non-SI units → calorie (where 1 calorie = 4.186 J → raises temperature of 1 g of H2O from 14.5°C to 15.5°C); Calorie (where 1 Calorie = 1000 calories = 4186 J)
- Heat can be transferred by:
- Conduction (via collision of molecules through a substance)
- Convection (via bulk flow of fluid (liquid/gas) surrounding the substance)
- Evaporation (via vaporisation of water from a substance’s surface → Nb. Latent heat of vaporisation of H2O is 580 cal/g or 2.4 MJ/kg at 37 °C)
- Radiation (via emission of EMR, often within IR band) → Nb. Unlike other heat transfer mechanisms, this can occur through a vacuum and does not require direct contact
- Heat transfer is dependent on the ambient environment:
- Heat is transferred down its “temperature gradient” (from ↑ to ↓ temperature)
- Relative humidity is vital for determining heat transfer via evaporation (as it establishes a “moisture gradient”)
Specific Heat
- Specific heat of substance is the amount of heat energy required to ↑ temperature of 1 g of substance by 1°C
- SI unit → J/kg (Eg. 4.2 kJ/kg per °C for H2O; 3.6 kJ/kg per °C for body tissue (of which 85% is H2O))
Bianca’s notes
CICMWrecks Answer: Temperature Regulation
Definitions
- Heat – A form of kinetic energy → being a state of “thermal agitation” of molecules in a substance
- Temperature – A measure of a physical property of a substance that determines the tendency for heat to flow from one object to another → heat energy is transferred from a region of higher temperature to a region of lower temperature
- Temperature setpoint is the level at which the body attempts to maintain its temperature.
- Core body temperature:
- Refers to deep body temperature of main internal organs (in head, trunk, abdomen) →
sites where metabolic activity occur (Ie. heat production) - Kept constant at 37 +/- 0.4 °C (“Normothermia”) → displays normal variations:
- Diurnal variation – ↑ in evening (37.3 °C) and ↓ in early morning (35.8 °C)
- Menstrual variation – ↑ 0.5 °C in latter half of cycle
- Refers to deep body temperature of main internal organs (in head, trunk, abdomen) →
- Variations in core body temperature:
- Normothermia → core body temperature 37 +/- 0.4 °C
- Hypothermia → core body temperature < 36 °C
- Hyperthermia → core body temperature > 37.5 °C
- Peripheral body temperature:
- Refers to body temperature peripherally (Ie. skin, arms, legs, superficial tissues of core
sites) → sites where heat loss occur - Temperature varies widely → always LESS than core body temperature
- Refers to body temperature peripherally (Ie. skin, arms, legs, superficial tissues of core
- Regulation of body temperature is done by balancing heat loss and heat production, predominantly through behavioural mechanisms and skin
Thermoneutral Zone
- The range of environmental temperatures in which the metabolic heat production (and oxygen consumption) is minimal and steady and where core temperature is maintained by vasomotor activity alone.
- 25-30 °C for a naked, upright man in still air
Interthreshold Range
- The range of core temprature over which no autonomic thermoregulatory responses occur
- Normally 0.2 -0.4 °C in a non-anaesthetized state
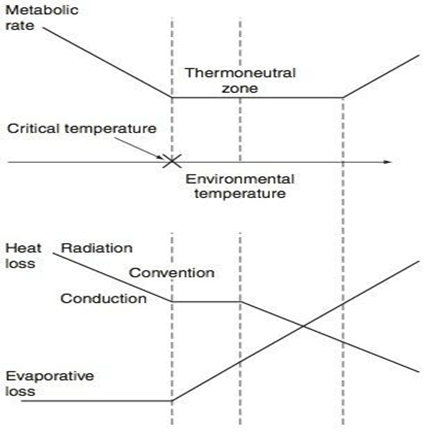
Regulation of Body Temperature
Temperature sensors are central and peripheral, whilst regulation occurs centrally, and has multiple
effectors
Cold | Warm | |
---|---|---|
Receptor | Bulbs of Krause | Bulbs of Ruffini |
Afferent nerve type | via Aδ and unmyelinated C sensory fibres | Unmyelinated C fibres |
Spinal synapse location | Rexed lamina 1,5 | Rexed lamina 1, 2 |
Ascending tract | Lateral spinothalamic tract in anterior spinal cord | Lateral spinothalamic tract in anterior spinal cord |
Brainstem synapse | Reticular system of medulla | Reticular system of medulla |
Central Processor | Hypothalamus (posterior) | Hypothalamus (anterior) |
Thresholds | No activity at 40 degrees ↑ing activity between 10-27 degrees (remember thermoneutral zone) | Activity with increasing between 30-45 °C Upper threshold limit = 46 °C |
Sensors:
- Central
- Deep tissues/viscera (Eg. in intestinal wall)
- Brain (anterior hypothalamus and extra-hypothalamic areas)
- Spinal cord
- Peripheral
- Dermis (main)
- Corneas
Central Controller:
Hypothalamus is the main body temperature regulatory centre
- Posterior hypothalamus responds to cold
- inputs from peripheral afferents
- responsible for the temperature set point
- ACh is the major neurotransmitter here.
- Anterior hypothalamus responds to heat
- both peripheral input and change in blood temperature
- Major neurotransmitters are Nad, 5HT, dopamine and prostaglandins.
Effector Mechanisms:
- Skin
- Sweating (evaporation)
- Secrete plasma-like fluid Na142, Cl- 104 (urea/K, etc v low amounts) osmolality controlled by rate of secretion and aldosterone
- loss via evaporation (latent heat of vapourisation of water)
- 1-2L in extreme exercise up to 10 L max per 25 hours.
- 0.54 kcal/gram of H2O evaporated
- Degree of evaporation determined by ambient temperature and relative humidity
- Blood Flow (radiation)
- 8 (300 mL/min) – 30% CO skin
- Vasodilation up to 30 fold increase
- Vasoconstriction up to 10 fold decrease
- vasoconstriction/dilation controlled by SNS (α1-mediated) vascular SM contraction and hypothalamic feedback
- Fat/Clothing
- Fat conducts heat 1/3 as readily as other tissues (decreased radiation)
- Clothes reduce conductive heat loss via private zone of air adjacent to skin and decreased convection air currents -50% and more if specialised
- Sweating (evaporation)
- Non-Skin
- Non-shivering thermogenesis
- Predominantly in brown fat
- Uncoupling of oxadative phosphorylation
- Increased heat gain without oxygen consumption/ATP production
- Shivering
- Increased heat gain by metabolism
- Behaviour
- For gain (exercise) or loss (submerging in water)
- Voluntary muscle contraction (Ie. ↑ activity with cold stress; ↓ with hot stress) – Affects heat production
- Body posturing (Ie. ↓ BSA with cold stress;↑ with hot stress) – Affects heat loss
- Clothing (Ie. ↑ clothing with cold stress; ↓ with hot stress) – Affects heat loss
- Appetite
- Cold stress stimulates food-induced thermogenesis → ↑ metabolic rate and heat production
- Thyroid hormone secretion
- Cold stress stimulates thyroid hormone secretion → long-term ↑ metabolic rate and heat production
- Non-shivering thermogenesis
Gladwin / JC 2019
Examiner Comments
2024A 16: 75% of candidates passed this question.
(a) The definition of temperature and heat varies considerably in texts, so reasonable leeway was granted in marking this part.
(b) In this section the following information was expected; body temperature range; thermoneutral zone; sensors (including receptors, fibres, and tracts); central processing (the role of the hypothalamus including anterior and posterior portions); interthreshold range; and a detailed description of the efferent responses for either increasing or decreasing body temperature.
17. (a) Define innate immunity including how it differs from adaptive immunity (10% of marks).
(b) Outline the components of the innate immune system including their role in the immune response (90% of marks).
CICMWrecks Answer
Definition
Innate immunity is the body’s first line of defense, present from birth, and provides immediate, non-specific protection against a wide range of pathogens. It does not require prior exposure to pathogens and lacks immunological memory, meaning its response does not improve with repeated exposure.
Feature | Innate Immunity | Adaptive Immunity |
---|---|---|
Presence | Present from birth | Develops after antigen exposure |
Specificity | Non-specific | Highly specific to antigens |
Response Time | Immediate | Delayed (days to weeks initially) |
Memory | No memory | Generates memory for faster response |
Receptors | Fixed pattern recognition receptors | Antigen-specific T and B cell receptors |
Cells | Macrophages, neutrophils, NK cells | T cells, B cells |
Duration | Short-lived | Long-lasting (due to memory cells) |
Components of Innate Immune System
Comprises of three components: Physicochemical barriers, Humoral defences, Cellular defences
1. Physiocochemical Barriers
prevent pathogenic factors from gaining access to body
- Epithelial barriers (skin, GIT, respiratory tract, genitourinary tract)
- Secretion of “antibacterial substances” (Eg. lysozyme in saliva, gastric HCl)
- High turnover of epithelial membranes
- High flow rates of urine, saliva and tears
- Acidic environment (especially low pH of stomach)
- Normal flora (prevents growth of foreign organisms)
- Mucous membranes secrete mucous that trap foreign particles and remove them via ciliary action
- Innate reflex mechanisms (Eg. coughing, sneezing, vomiting, diarrhoea, Etc.)
2. Humoral defences
- Complements
- Proteolytic system present in plasma.
- Group of serum proteins produced by hepatic parenchymal cells and also macrophages at sites of inflammation.
- Activated in a sequential manner via two separate pathways (classical and alternate), which come together in a final common pathway involving the breakdown of C3.
- Acute phase proteins – Plasma proteins produced by liver during acute
inflammation/infection (Eg. CRP, Fibrinectin, α1AT, α2-macroglobulin) → aids in
opsonisation, complement activation and regulation of the AIR - Proteolytic enzymes (Eg. lysozyme) – Break down bacterial cell wall → lysis
3. Cellular defences
a) Granulocytes
- Neutrophils → main defence against bacteria and fungi as follows:
- At site of infection, tissue damage releases mediators that activate
circulating PMNLs and vascular endothelium and causes them to express
adhesive proteins → results in “Margination” (PMNL to move along
margin of endothelium) and “Diapedesis” (PMNL migrate through
endothelium into tissues) - “Chemotaxis” – PMNL are drawn towards chemotactic factors (Eg. cytokines, complement, Etc.) in tissues
- “Phagocytosis” – PMNL recognise foreign material (Eg. bacteria) using “surface receptors” (Eg. LPS) → leads to cellular ingestion. This process is enhanced by “Opsonisation”, whereby the foreign material is coated with an “Opsonin” (Eg. IgG or C3b)
- “Killing/Digestion” – Phagocytosed material is incorporated into a “Phagosome” and destroyed by an O2-dependent process (via respiratory burst using H2O2, OH-and O2 generated from O2) and non-oxidative process (via lysosomal enzymes)
- At site of infection, tissue damage releases mediators that activate
- Eosinophils
- Mainly present in tissues (esp epithelial surfaces) cf. circulation
- Mainly implicated in allergic and parasitic responses → via release of “basic” granular material
- Mast cells and Basophils
- Basophils circulate in blood → become Mast cells when they enter tissue
- Mainly implicated in allergic and parasitic responses → degranulate and release mediators (histamine, 5-HT, SRS-A, Etc.) when IgE binds to it
b) Monocytes and Macrophages
- “Monocytes” enter blood from BM for 3 days before entering specific tissues (esp the reticuloendothelial system) → once in tissue, they can stay there for months-years as “Tissue Macrophage” (Eg. Liver (Kupffer Cells), Lung (Alveolar and Interstitial Macrophages), Bone (Osteoblast), CNS (Microglia), Kidney (Mesangial), LN and Spleen (sinus macrophages), Skin (Langerhans cells))
- Actions of tissue macrophages
- Phagocytosis and killing/digestion of IC pathogens (Eg. Listeria), mycobacteria, parasites (Eg. Trypanosomes), and fungi
- Ag presentation to T-cells
c) NK Cells
- Large granular lymphocytes (resemble lymphocytes morphologically but lack TCR, CD3 and Ag clonal specificity)
- Actions:
- Lyse viral-infected cells, tumour cells, and allogenic (graft) cells:
- Possess receptors that allow it to identify target cells – recognises (i) downregulation of MHC I (Eg. viral infected cell) and (ii) IgG bound to target cells
- Causes cell lysis via – (i) Apoptosis (nucleus of target cell is rapidly fragmented by endonucleases), and (ii) Release of Perforin/Granzyme granules onto the target cells
- Release of cytokines (IFN-γ and TNF) that activate phagocytes and recruit T-cells
- Lyse viral-infected cells, tumour cells, and allogenic (graft) cells:
Bianca’s notes
Examiner Comments
2024A 17: 17% of candidates passed this question.
Expectations for a high scoring answer were:
(a) a definition of innate immunity featuring its presence since birth, the non-specificity and lack of memory when compared with the adaptive immune system,
(b) an outline of different components of innate immune system including; physicochemical (skin, mucus, cilia, gastric acid), humoral (lysosome, complement, acute phase proteins) and cellular (neutrophils, macrophages, natural killer cells, mast cells). In addition to listing a number of these examples, a brief explanation (“outline”) of their function should be included.
18. Compare and contrast the use of external ventricular drains with intraparenchymal fibreoptic pressure monitors to measure intracranial pressure.
CICMWrecks Answer
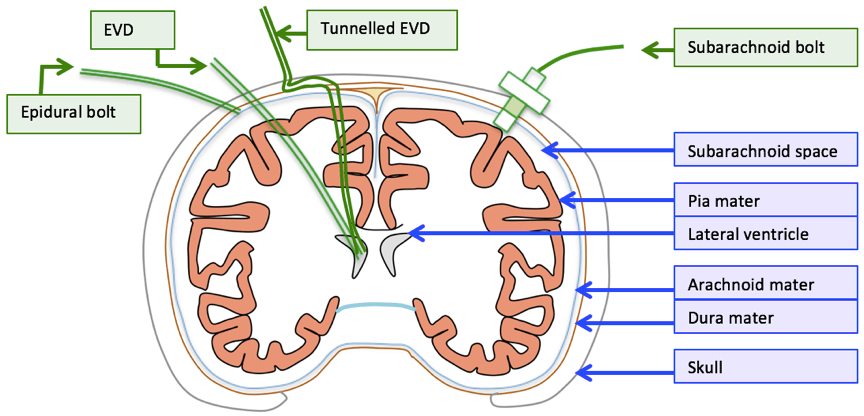
EVD | Intraparenchymal fiberoptic | |
Description | • A flexible plastic catheter placed inside the lateral ventricles. • Can be either unilateral or bilateral placement • Gold standard for ICP monitoring | A think catheter inserted into parenchyma of the brain |
Indication | • Hydrocephalus • Post surgical/trauma intracranial haemorrhage • Meningitis | same |
Location of tip | Lateral ventricles | Below the dura – usually a few cms |
Component | • Plastic catheter • Wheatstone bridge • Fluid filled non-compressible tubing • Pressurized fluid bag • Drainage bag • Monitor | • Microsensor – intracranial • Fiber Optic cable • Monitor |
Principle | Pressure is transmitted to a Wheatstone bridge transducer via fluid filled non-compressible tubing | Changes in ICP move a displaceable mirror at the tip of the sensor to alter the intensity of light reflected back along the fiber optic cable |
calibration | Able to be zero’d post insertion | Cannot be zero’d post insertion |
Sources of error | • Migration of catheter • Blockage • Incorrect leveling to tragus • Damping and resonance | • “Drift” • Increased inaccuracy >72hrs • Only measures ICP locally |
Advantage | • Has diagnostic values (CSF sampling, elevated ICP, new haemorrhage) as well as therapeutic values (drainage of excessive CSF, administration of medication) • Global measurement of ICP • Can be converted to cerebral shunt – long term treatment • Cheap | • Less expertise required for insertion • Less traumatic • Able to be placed in patients with small collapsed ventricles • Less infection risk • No risk of blockage |
Disadvantage | • More skill required for insertion • More traumatic compared to bolt • Increased risk of ventriculitis • May be blocked • Complications: bleeding, malplacement, obstruction, migration, infection | • Only local ICP is measured • No therapeutic value: CSF cannot be drained • Less accurate due to drift and unable to be calibrated post insertion • Expensive |
Guo 2021
Examiner Comments
2024A 18: 38% of candidates passed this question.
This question required an accurate explanation of the mechanism of measurement for each device. A head to head comparison of the differences and similarities in utility, accuracy, global versus regional measurements, calibration, drift and dampening should follow. A comparison of potential complications was also expected.
19. Describe the pharmacology of amiodarone using the following headings:
(i) Indications for use (5% of marks)
(ii) Dose (10% of marks)
(iii) Mechanism of Action (20% of marks)
(iv) Pharmacodynamics including adverse effects (25% of marks)
(v) Pharmacokinetics (25% of marks)
(vi) Drug Interactions (15% of marks).
Examiner Comments
2024A 19: 65% of candidates passed this question.
The headings were provided to candidates to ensure that information was limited to these domains. This question required specific detail about amiodarones action at K+, Na+ , B and Ca channels, including second messenger systems and effects on SA / AV and myocyte action potential. Simply stating that there is hepatic metabolism and renal excretion is not enough information for pharmacokinetics and some detail was expected regarding the mechanism of metabolism, presence (or absence) of active metabolites and organs involved in clearance with rough figures for half life, Vd, loading dose and clearance values. It is recommended to include the mechanisms by which side effects occur rather than just listing them.
20. Outline the important similarities and differences between unfractionated heparin and enoxaparin using the following headings (20% of marks each):
(i) Mechanism of action
(ii) Pharmacokinetics
(iii) Adverse effects
(iv) Dosing
(v) Monitoring and reversal
Examiner Comments
2024A 20: 27% of candidates passed this question.
These are commonly used and important drugs in intensive care practice and a high level of detail was expected. Unfractionated heparin and enoxaparin have specific differences that influence how we use them in practice and this information was required for full marks. This question was answered well by providing the detail on each domain for both heparin and enoxaparin and then highlighting if this factors into how they are used.
VIVAs
A. Pharmaceutics | |
B. Pharmacokinetics | Bioavailability, curve |
C. Pharmacodynamics | |
D. Variability in Drug Response | |
E. Cellular Physiology | |
F. Respiratory | Work of breathing and components, diagram Terminal respiratory unit, structure, functions FRC – factors between and within healthy individuals |
G. CVS | Arterial BP waveform from aorta to periphery Cardiac cycle, ECG+CVP |
H. Renal | Renal – K handling |
I. Body Fluids and Electrolytes | |
J. Acid Base | ABG |
K. Neuro | Perception of pain following a noxious stimulus |
L. Musculoskeletal | NMJ, NM blockers, TOF |
M. ANS | |
N. Liver | Liver, bile |
O. GIT | GI secretions |
P. Nutrition and Metabolism | |
Q. Haematology | TEG, diagram Haem – Group and Screen |
R. Thermoregulation | |
S. Immunology | |
T. Microbiology | |
U. Endocrine | Adrenal gland physiology, pharmacology, ACTH |
V. Obstetrics | O2 delivery to placenta and fetus |
W. Measurement and Monitoring | |
X. Procedures |
Recent Comments