SAQs
1. Describe the physiological consequences of the oral ingestion of 1 litre of water in a young adult.
CICMWrecks Answer
Water
- Water is distributed across all body compartments
- It is hypotonic compared to all body compartments
- It theoretically gets distributed to ECF (Plasma and ISF) + ICF
- Absorption of ingested water occurs in proximal small intestine.
- Absorption rate depends on rate of gastric emptying and creation of suitable osmotic gradient across the intestinal mucosa.
- 1L Oral ingestion of water is rapidly absorbed in the small intestine.
Mechanism of Absorption of water
- Sodium is absorbed from the intestinal lumen by several mechanisms, most prominently by cotransport with glucose and amino acids, and by Na+/H+ exchange, both of which move sodium from the lumen into the enterocyte.
- Absorbed sodium is rapidly exported from the cell via sodium pumps – when a lot of sodium is entering the cell, a lot of sodium is pumped out of the cell, which establishes a high osmolarity in the small intercellular spaces between adjacent enterocytes.
- Water diffuses in response to the osmotic gradient established by sodium – in this case into the intercellular space. It seems that the bulk of the water absorption is transcellular, but some also diffuses through the tight junctions.
- Water, as well as sodium, then diffuses into capillary blood within the villus.
Water compartments and Responses
- TBW is one-third ECF & two-thirds ICF
- ECF is one-quarter plasma & three-quarters ISF
- The threshold of the volume receptors is 7-10% change in blood volume
- The osmoreceptors are sensitive to a 1-2% change in osmolality.
- Plasma osmolality is normal prior to the transfusion (ie 287-290 mOsm/kg)
Effect of 1L oral intake
- Absorption of water causes a drop in plasma osmolarity without much effect on plasma volume.
- There is a brief period of hypervolemia, increase in arterial blood pressure and an associated physiological reflex response mainly by osmoreceptors
- There will also be an associated increase in renal perfusion and stimulation of intrarenal receptors (e.g. juxtaglomerular apparatus).
- Sensor: Osmoceptors in hypothalamus
- CPU: Hypothalamus
- Effector: ADH (and thirst, not significant in current scenario)
- Anti-diuretic hormone (ADH)
- peptide hormone synthesised in the hypothalamus
- released from the posterior pituitary
- acts on the kidney via GPC V2 receptors
- increases the synthesis of aquaporins
- ADH secretion is decreased,
- ➔ water permeability in the nephrons is increased
- ➔ leads to less water reabsorption
- ➔ larger amount of dilute urine excretion
- Consequence is homeostasis by excretion of the 1L of water quite rapidly.
JC 2019
Examiner Comments
2019B 01: 28% of candidates passed this question.
It was expected candidates would provide details the consequences of water ingestion from its rapid absorption in the small intestine to the resultant impact on plasma osmolarity and the minimal impact of plasma volume of this volume. Some detail on the mechanisms of absorption (transcellular vs osmosis) was expected and the distribution of water across body fluid spaces.
Many candidates accurately described the small drop in plasma osmolarity that is sufficient to trigger osmoreceptors with better answers providing details of the locations and mechanisms involved. The physiological consequences of inhibition of ADH, including the renal effects of decreased water permeability in distal renal tubules and collecting ducts. The volume load after distribution would be lower than the plasma volume triggers for the circulatory reflex responses.
2. Describe renal blood flow and its regulation (80% of marks).
Outline the impact of adrenoreceptor agonists on renal blood flow (20% of marks).
CICMWrecks Answer
Regulation of Renal Blood Flow
- Renal blood flow Autoregulated between 80~170mmHg
- Blood flow maintained by modulating resistance based on pressure
- Renal vascular resistance maintained by interlobular arteries, afferent arterioles and efferent arterioles, amenable to external regulation
- GFR approx 180l/day – Autoregulated by tubuloglomerular feedback – relatively constant in response to fluctuating renal blood flow
INTRINSIC Regulation (Autoregulation) of GFR and RBF:
- Renal blood flow (and consequently GFR) Autoregulated between MAP range of 75~170mmHg
- Blood flow maintained by modulating resistance of AFFERENT based on pressure
- Efferent arteriole is NOT involved in autoregulation!
- Autoregulation of GFR and RBF can be overridden by external influences (Eg. hormones and SNS neurons), even when renal perfusion pressure is between MAP 75-170 mmHg!
Mechanisms of Autoregulation:
- Myogenic autoregulation (Myogenic stretch response):
- In response to vascular wall stretch (due to increased intraluminal pressures), stretch dependent Ca influx occurs causing vasoconstricion of arterioles → increased resistance according to Poisuille-Hagen Equation → Decreased flow
- In response to shear stress (due to increased flow), Endothelial derived relaxation factors released (such as NO) → NO acts on guanylyl cyclase → increased cGMP → smooth muscle relaxation → arteriolar vasodilation
- Tubuloglomerular feedback (TGF)
- Negative feedback – Links the rate of GFR to concentration of salt in tubular fluid at macula densa
- Macula densa in wall of Ascending limb of loop of Henle Detects change in tubular flow (by the changing salt concentrations)
- As a consequence of decreased blood flow → GFR decreases → Tubular flow rate decreases → Increased uptake of [Na] in Ascending LoH → Reduced [Na+] and [Cl-] reaching the DCT and macula densa → Juxtaglomerular apparatus releases prostaglandins (PGE2) → vasodilation of afferent arteriole → increased resistance to glomerular blood flow
- With increased GFR → Increased tubular flow rate → Decreased [Na] uptake by the LoH → Increased [Na+] in macula densa → Juxtaglomerular apparatus secretes adenosine → Vasoconstriction of the afferent arteriole → Decreased blood flow
Note: Flow to Juxtamedullary nephrons is not autoregulated. High blood pressure increases juxtamedullary flow, increasing GFR and impairing renal concentration, resulting in a pressure diuresis.
Extrinsic Control of GFR and RBF:
- Hormonal regulation of blood flow
- Afferent arterioles:
- Dilation → PGE-2, PGI-2, DA, ANP, NO, kinins
- Constriction → High dose AII, NAd, ET-1, Adenosine, ADH, Thirst
- Efferent arterioles:
- Dilation → Inhibition of AT-II
- Constriction → Low dose AT-II
- Mesangial cell → Contracts due to AT-II, ADH and NAd (contraction response inhibited by ANP, DA, PGE2, PGI2)
- Note: Angiotensin II:
- At physiological (low) doses → it maintains GFR by efferent arteriolar vasoconstriction (at expense of RBF)
- With ↑ AT-II levels → causes:
- BOTH afferent and efferent arterioles constriction→ ↓ GFR
and RBF - Mesangial cell contraction in renal corpuscle → ↓ KF → ↓
GFR
- BOTH afferent and efferent arterioles constriction→ ↓ GFR
- Afferent arterioles:
- Neural regulation of renal blood flow (SNS, noradrenergic)
- All renal vescles richly innervated by sympathetic nerves
- 2 Mechanisms:
- Constricts BOTH afferent and efferent arterioles → ↓ RBF >>> GFR
- Stimulates renin secretion (via β1 receptors on JG cells) → ↑ Angiotensin II production → afferent and efferent arteriolar vasoconstriction → ↓ RBF and GFR
- Starling resistors
- Increased intra-abdominal pressure will decrease blood flow in starling resistor model
- Increased intra-capsular pressure will decrease renal blood flow
- High blood amino acid/ glucose level
- High filtered AA/ glucose load → reabsorption in PT with Na → ↓NaCl reaches distal tubules → macula densa → ↓adenosine → vasodilation → ↑RBF
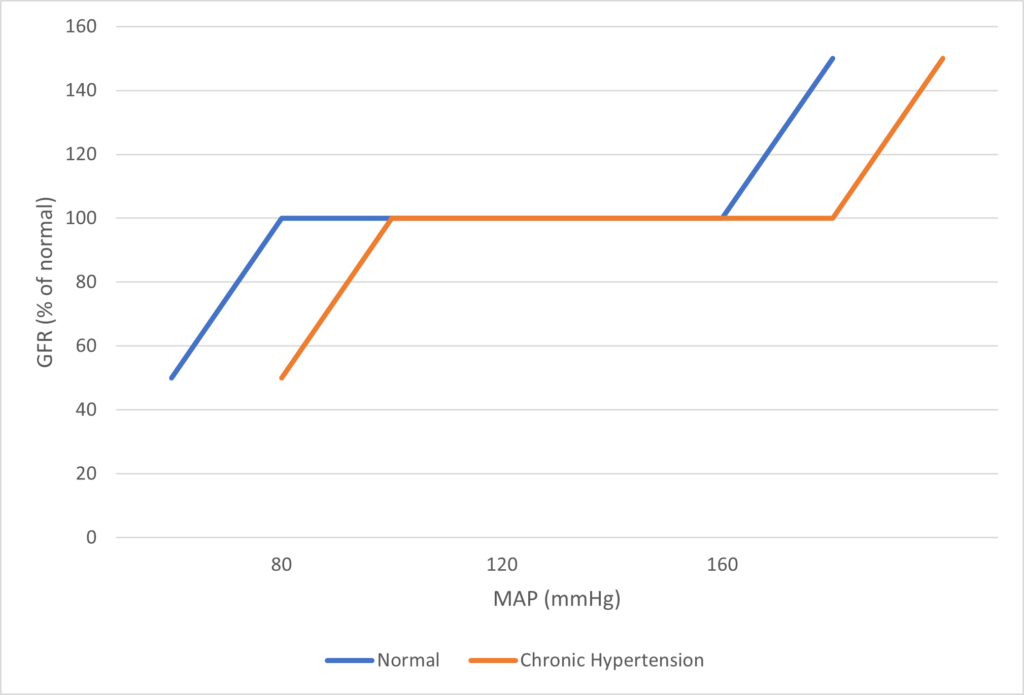
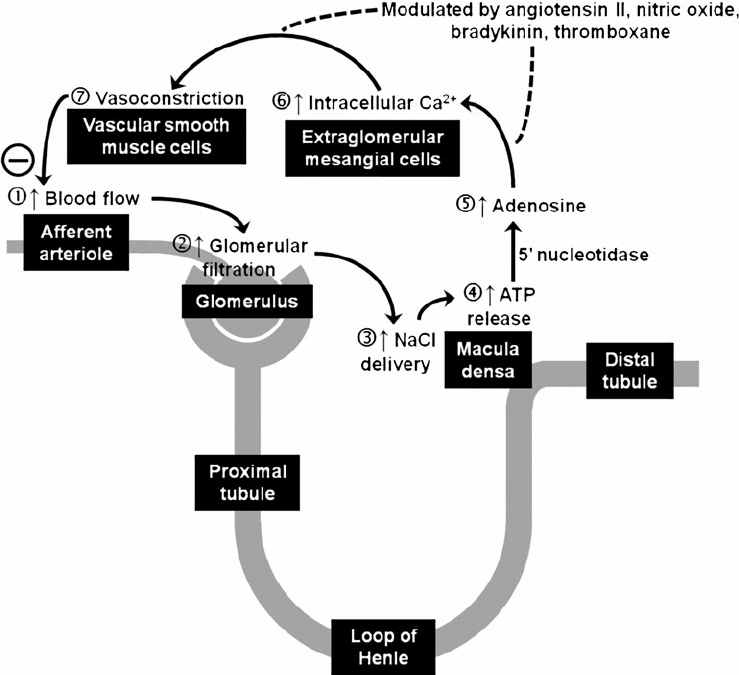
Image Source
Effect of Adrenoreceptor agonists on Renal Blood Flow
The impact of adrenoreceptor agonists is varied based on the amount of direct action in the kidney, indirect effect of altered cardiac output on Renal blood flow.
- Generally sympathomimetic agents will vasoconstrict and therefore increase renovascular resistance and result in a decrease renal blood flow.
- The relative impact on afferent vs efferent arteriolar tone may alter glomerular perfusion pressure.
Receptor stimulation effects:
- Alpha1 and Alpha2 stimulation → renal vasoconstriction → Decreased renal blood flow and GFR
- Beta1 stimulation:
- Renin release → Angiotensinogen to Angiotensin I → AT1 to AT2 by ACE → constriction of the afferent and efferent arterioles → reduces medullary blood supply
- Can cause positive inotropic effect which increases Cardiac output and can increase renal blood flow
*Note: Dopamine can cause renal vasodilatation in low doses to improve renal blood flow and increase GFR, but this action is via Dopamine DA1 receptors, not adrenergic receptors
JC / Mooney / Sakurai 2019
Examiner Comments
2019B 02: 64% of candidates passed this question.
This question was well answered by most candidates. The description of renal flow involves a brief comment of the anatomy including interlobar, arcuate, interlobular arteries, then afferent and efferent arterioles – 2 sets of capillaries and then corresponding veins and better answers made the distinction better cortical and medullary flow and went on to detail the consequence of this. Renal blood flow is autoregulated and most candidates describe well the various mechanisms around myogenic and tubuloglomerular feedback.
Additional marks were gained with by discussing renal vascular resistance and how this may be varied. The impact of adrenoreceptor agonists is varied but generally sympathomimetic agents will vasoconstrict and therefore increase renovascular resistance and result in a decrease renal blood flow. The relative impact on afferent vs efferent arteriolar tone may alter glomerular perfusion pressure.
3. Describe the relationship between muscle length and tension (50% of marks).
Outline the physiologic significance of this relationship in cardiac muscle (50% of marks).
CICMWrecks Answer
Muscle length and tension
- during isometric stimulation the total tension can vary with muscle length (total tension)
- also when unstimulated, tension can vary with muscle length (passive tension)
- this relationship can be studied in a muscle preparation study
- muscle length can be varied by changing the distance between its two attachments -> passive tension measured.
- muscle then electrically stimulated -> total tension measured.
- the difference between the two values at any length is the amount of tension generated by the contractile process -> the active tension.
Passive tension curve = tension exerted by this skeletal muscle at each length when not stimulated.
Total tension curve = tension developed when the muscle contracts isometrically in response to a maximal stimulus.
Active tension = difference between the two.
- the length at which the active tension is maximal is usually = resting length
- when muscle fibers contract isometrically -> tension developed is proportionate to the number of cross-linkages between the actin & myosin molecules.
- when muscle stretched -> overlap reduced -> potential tension reduced
- when muscle shorter -> distance filaments have to move is reduced -> potential tension reduced.
- Optimal length = resting length
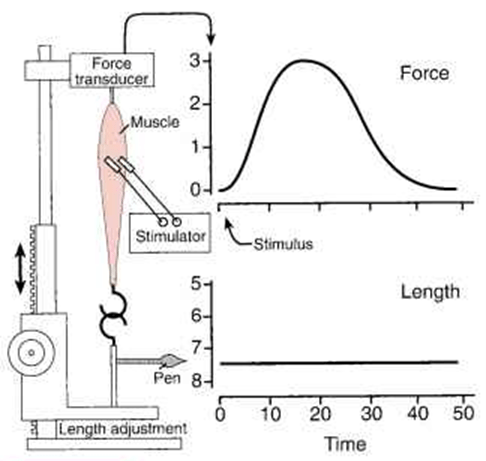
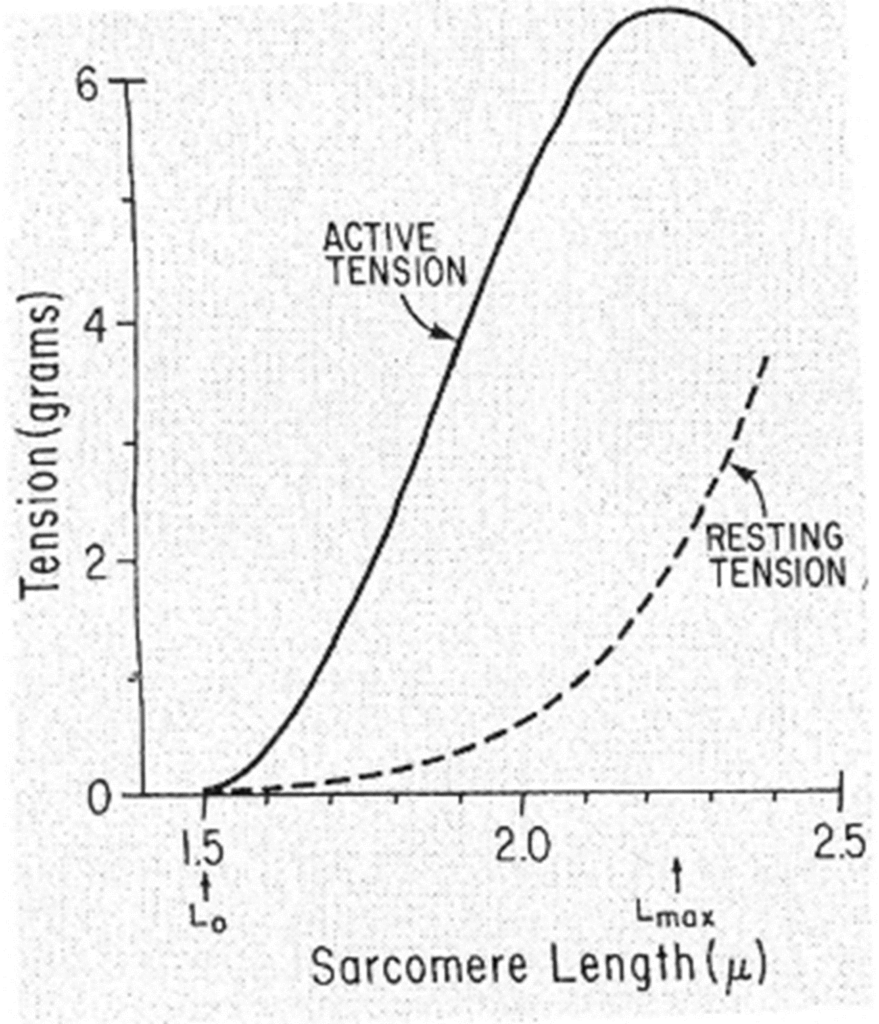
Frank-Starling Law
“energy of contraction is proportional to the initial length of the cardiac muscle fiber.”
- For the heart, the length of the muscle fibers (ie, the extent of the preload) is proportionate to the end- diastolic volume.
- Increased venous return increases the end-diastolic volume and therefore preload, which is the initial stretching of the cardiac myocytes prior to contraction.
- Myocyte stretching increases the sarcomere length, which causes an increase in force generation. Increasing the sarcomere length increases troponin C calcium sensitivity, which increases the rate of cross-bridge attachment and detachment, and the amount of tension developed by the muscle fiber. The effect of increased sarcomere length on the contractile proteins is termed length-dependent activation.
- This ability of the heart to change its force of contraction and therefore stroke volume in response to changes in venous return is called the Frank-Starling mechanism.
- The mechanism is very important for:
- Rapidly responding to acute changes in venous return
- Keeping the right and left ventricle outputs exactly equal
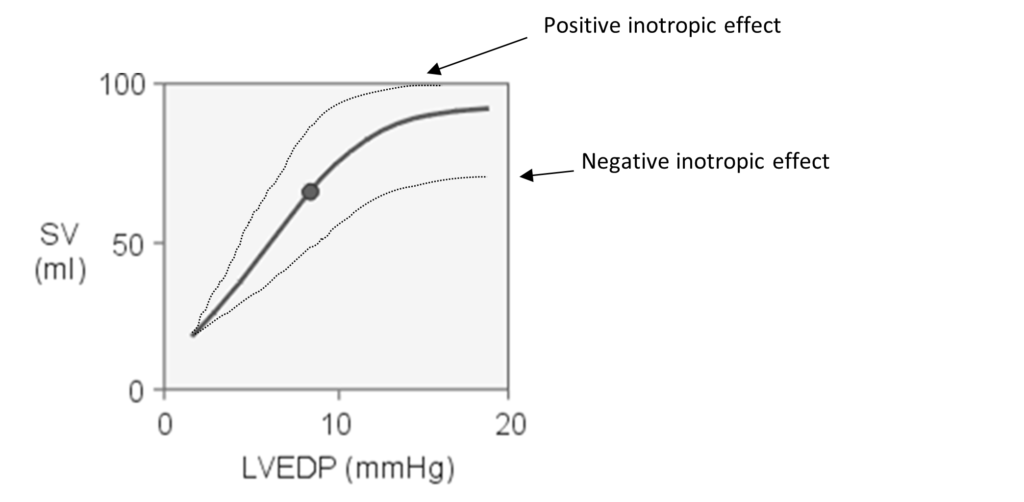
Frank-Starling curve: Relationship between ventricular stroke volume and end-diastolic volume
JC 2019
Examiner Comments
2019B 03: 41% of candidates passed this question.
Some detail was expected on a general description that tension is variable with the length of muscle. It was expected answers would describe that there is a resting length at which tension developed on stimulation is maximal.
Many candidates omitted that differences exist between muscle types with smooth muscle behaving differently. Additional credit was given for the distinction about active tension vs resting tension. It was expected a description of the potential mechanism would be included with discussion of sliding filament theory, overlapping fibres and optimal sarcomere length. Some candidates utilised a diagram effectively to convey understanding and more detail was rewarded with additional marks.
The second half of the question involved describing how this relationship is particularly important in cardiac muscle and underpins the Frank Starling relationship and all the cardiac physiology that follows. Initial length of fibres is determined by the diastolic filling of the heart, so pressure developed is proportionate to the total tension developed. The developed tension increases as diastolic volume increases to a maximum (the concept of Heterometric regulation). Better answers appreciated that the physiology may be different for a while heart rather than isolated muscle fibres.
4. Outline the pharmacology of intravenously administered magnesium sulphate.
Examiner Comments
2019B 04: 55% of candidates passed this question.
Overall answers were well structured. However, a lack of detail and inaccurate pharmacokinetics was common. Better answers included a discussion of the mechanism of action of Mg++ including Ca++ antagonism, presynaptic cholinergic effects and NMDA receptor antagonism. Adverse effects were not discussed in detail by many candidates and contraindications were commonly omitted.
5. Describe the anatomical course and relations of the trachea and bronchial tree (to the level of the segmental bronchi).
CICMWrecks Answer
Tracheobronchial Tree
Trachea →
Primary main bronchi →
Lobar bronchi →
segmental bronchi (each supplies one bronchopulmonary segment) →
divide dichotomously→→→→
terminal bronchioles →
respiratory bronchioles →
2-11 alveolar ducts →
alveoli
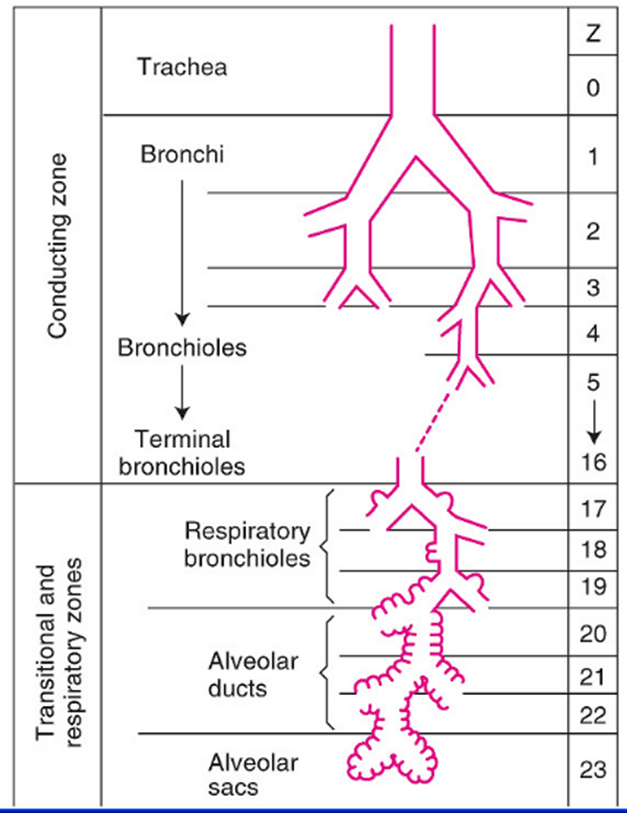
Trachea
- Midline. 10-12 cm long 15-20mm diameter.
- From Cricoid (C6 level) to bifurcation (T4-T6 level)
- 16-20 C-shaped cartilage rings
- Vertical fibroelastic tissue, and posterior trachealis muscle
- Arterial supply: Upper 2/3 – Inferior thyroid artery, Lower 1/3 Bronchial artery
- Venous drainage: Inferior thyroid vein
- Lymphatics: Drain into deep cervical, pre-tracheal, paratracheal lymph nodes
- Nerve supply: Recurrent laryngeal nerve, Sympathetic fibres from middle cervical ganglion
Bronchi
- Supplied by bronchial arteries which run along the bronchi (with vessels from pulmonary circulation)
- Drains into bronchial veins
- Similar innervation as trachea
- A bronchopulmonary segment is a portion of lung supplied by a specific segmental bronchus and arteries.
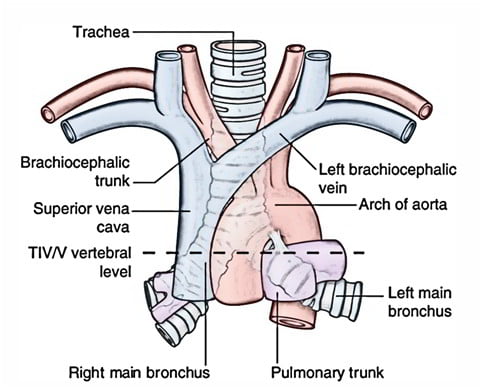
Right main bronchus
2cm long, I.D. 10-16mm
Shorter, wider, more nearly vertical than left
Branches:
- Right upper lobe
- Apical
- Anterior
- Posterior
- Bronchus intermedius
- Middle lobe
- Medial
- Lateral
- Lower lobe
- Superior
- Medial basal
- Anterior basal
- Lateral basal
- Posterior basal
- Middle lobe
Left main bronchus
4-5cm long, I.D. 8-14mm
Crosses anterior to the esophagus, which it indents.
Branches:
- Left upper lobe
- Apicoposterior
- Anterior
- Lingular
- Superior
- Inferior
- Left lower lobe
- Superior
- Anterior basal
- Lateral basal
- Posterior basal
Lining Epithelium
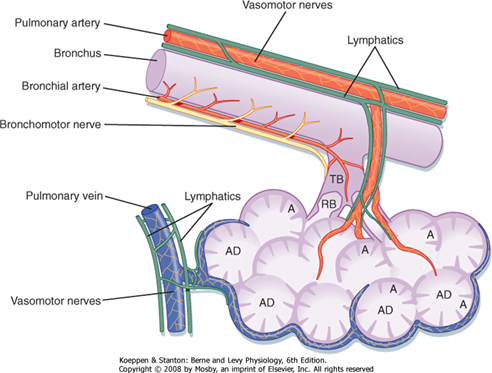
Trachea → Terminal bronchioles: ciliated pseudostratified columnar epithelium
Respiratory bronchioles → alveolar ducts → alveoli: Non ciliated cuboidal epithelium
Sources: Nunn’s Applied Respiratory Physiology, West’s Respiratory Physiology, Berne and Levy Physiology
JC 2019
Examiner Comments
2019B 05: 14 % of candidates passed this question.
To pass this question, the following were required for each section (trachea and main bronchi): landmarks; basic structural anatomy; and important relations (major vessels; major nerves; major structures). Marks were also allocated for innervation, and blood supply and venous drainage of the
trachea. Most unsuccessful answers did not address a number of these areas. Overall, the answers were better for tracheal anatomy compared to bronchial anatomy. A structured approach to anatomy questions works well and this was again the case (i.e. relations / blood supply / etc.
6. Outline the factors that determine central venous pressure and explain how it is measured.
CICMWrecks Answer
CVP
- Central venous pressure (CVP) is mean vena caval or right atrial pressure
- In the absence of tricuspid stenosis, equals right ventricular end-diastolic pressure.
- expressed in mmHg or cmH2O (1.36 cm water = 1.0 mm Hg)
- Normal 0-6mmHg in spontaneously breathing non-ventilated patient
- Venous Return, VR = (MSFP – RAP or CVP) / Resistance to venous return
- CVP is a major determinant of RV filling pressure (RV Preload)
- This regulates stroke volume through Frank-Starling mechanism
- CVP increased in disorders that increase Rt sided diastolic pressures
- left heart disease, lung disease, primary pulmonary hypertension, and pulmonic stenosis
- CVP is determined by an interplay of various factors
- Mainly influenced by volume of blood and compliance of central compartment
Factors affecting the measured CVP:
- Central venous blood volume (Inc volume à inc flow à inc CVP)
- Venous return/cardiac output
- Vena cava compression (Obesity/Valsalva/pregnancy/inc IAbd pressure – dec VR à dec CVP)
- Total blood volume
- Regional vascular tone
- Venous return/cardiac output
- Compliance of central compartment (inc Comp à inc return and venous pressure)
- Vascular tone
- Right ventricular compliance
- Myocardial disease
- Pericardial disease
- Tamponade
- Tricuspid valve disease
- Stenosis (inc mean CVP)
- Regurgitation (transient inc CVP)
- Cardiac rhythm (dec CVP with no atrial contraction, inc CVP when RA contracting against closed TV)
- Junctional rhythm
- AF
- A-V dissociation
- Reference level of transducer (inc or dec)
- Positioning of patient
- Intrathoracic pressure (inc pressure à inc CVP)
- Respiration (Inspiration makes intrathoracic pressure more negativeà increases CVP)
- Intermittent positive pressure ventilation (IPPV)
- Positive end-expiratory pressure (PEEP)
- Tension pneumothorax
Measurement
- Invasive:
- by inserting central venous catheter into Internal Jugular or Subclavian Vein
- Non-Invasive:
- Height of JVP provides visual estimate
- peripheral vein collapse on the dorsum of the hand or antecubital fossae
- Bedside ECHO and doppler techniques: IJV, Hepatic veins, IVC assessment
Invasive Measurement:
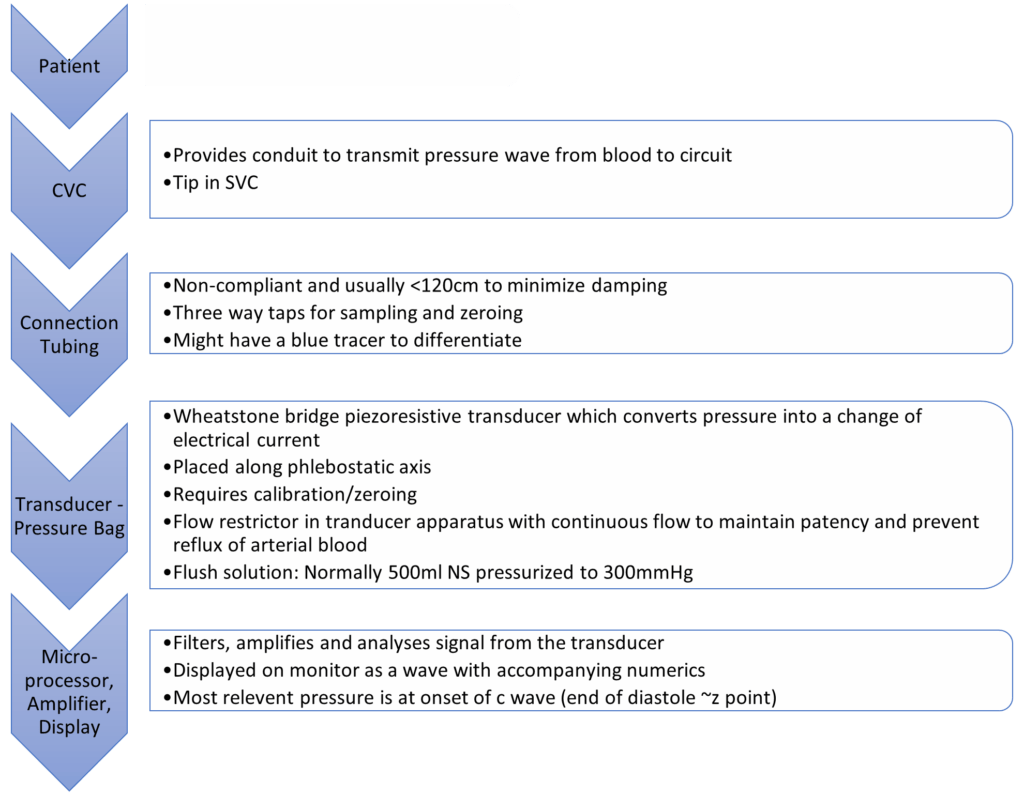
Complications of CVP Monitoring
- Mechanical complications:
- Arterial puncture and cannulation, Hematoma, Hemothorax, Surrounding nerve injuries, Pneumothorax, Embolization of broken catheter or guide wire, Air embolus, Arrhythmias, Lymphatic system injury
- Infectious complications:
- Sepsis, Endocarditis
- Thrombotic complications:
- Venous thrombosis, Pulmonary embolism
CVP Waveform
(not required for this answer)
Mechanical | ECG | Clinical | |
---|---|---|---|
a wave | Atrial contraction | Starts just after p wave ends | Closely parallels increase in RVEDP |
z point | Just before closure of TV (just at onset of c wave) | Coincides with middle of QRS | Good indicator of RVEDP esp when a waves not visible (AF) |
c wave | Closure of tricuspid valve Crest – bulging of TV | Correlates with end of QRS complex | |
x descent | Fall in intra atrial pressure during atrial relaxation | Before T wave | |
v wave | Passive atrial filling Crest – opening of TV | Usually after t wave | |
y descent | Decreased atrial pressure as RA empties into RV | Before p wave |
Changes in waveform:
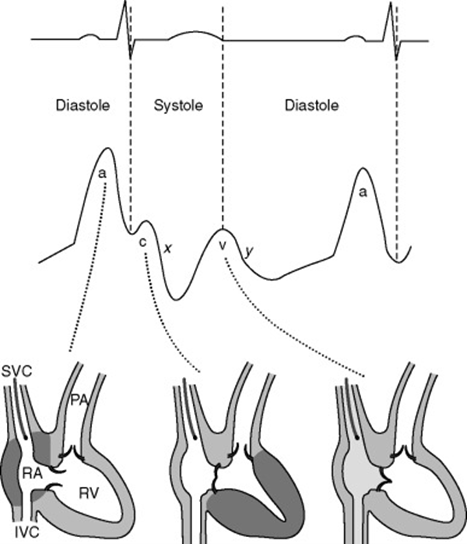
AF | a wave lost, c wave more prominent. If coarse AF, fibrillation waves may be visible |
A-V dissociation / Junctional rhythm | tall canon a waves (due to atrial contraction against closed Tricuspid valve) |
TR | large fused c-v wave (due to blood ejected back during ventricular systole) |
TS | attenuated a-wave and y-descent (increased pressure required to overcome TS) |
RV compliance decreased | a wave accentuated |
Pericardial constriction | short steep y-descent |
Cardiac tamponade | CVP monophasic with a single x-descent |
Sources:
Daniel Saddawi-Konefka, Critical Care Secrets
Shay McGuinness Cardiothoracic Critical Care 2007
Richard E. Klabunde, CVphysiology.com
T. Smith, Central Venous Pressure: Uses and Limitations
JC 2019
Examiner Comments
2019B 06: 18% of candidates passed this question.
It was expected that answers include central venous blood volume, central venous vascular compliance, intrathoracic pressure and tricuspid valvular function. Good answers outlined how each of these factors determine CVP and whether it was increased or decreased. Many candidates incorrectly described the effect of venous return.
7. Define closing capacity (10% of marks). Describe the factors that alter it (30% of marks),
its clinical significance (30% of marks) and one method of measuring it (30% of marks).
CICMWrecks Answer
Closing Capacity
- “Lung volume at which small airways and alveoli in the dependent parts of the lung first begin to close”
- Closing capacity = Closing volume + Residual volume
Pathophysiology and clinical significance of closing capacity
- Airways and alveoli in the dependent parts of the lung are much smaller (cf. nondependent regions), thus with expiration to low lung volumes below FRC these dependent airways and alveoli begin to collapse at “closing capacity” and trap gas distally, thereby causing “atelectasis”
- During atelectasis, a shunt forms (V/Q = 0) as the alveoli affected are not ventilated but remain perfused. This leads to impaired gas exchange that results in arterial hypoxaemia
Factors increasing closing capacity
- Age:
- CC=FRC at 44yrs in supine position, CC=FRC at 66yrs in upright position
- FRC depends on position, CC is independent
- Increasing abdominal pressure
- Decreased pulmonary blood flow
- Pulmonary parenchymal diseases which decrease compliance
- Obstructive airway diseases
- LV failure
- Surgery
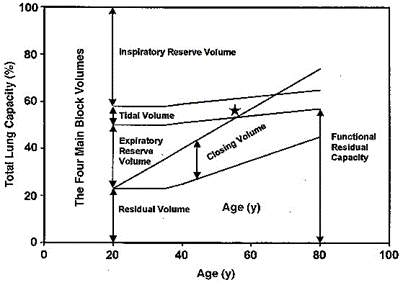
Zaugg M, Lucchinetti E. Respiratory function in the elderly. Anesthesiol Clin North America 2000; 18: 47–58
Measuring closing capacity
Closing volume is determined using the “Single breath N2 test” (similar to Fowler’s method):
- Following a VC breath of 100% O2, the patient slowly exhales and a expired [N2] is measured with a rapid N2 analyser
- A plot of [N2] vs volume of gas expired is made:
- Phase 1: N2 in anatomical dead space
- Phase 2: N2 from anatomical dead space and alveolar gas
- Phase 3: Alveolar plateau is formed by N2 in pure alveolar gas
- Phase 4: Late in expiration when AW closure starts of occur, expired [N2] begins to rise above the alveolar plateau. The volume expired from the start of this to the end of maximal expiration is the “Closing volume”
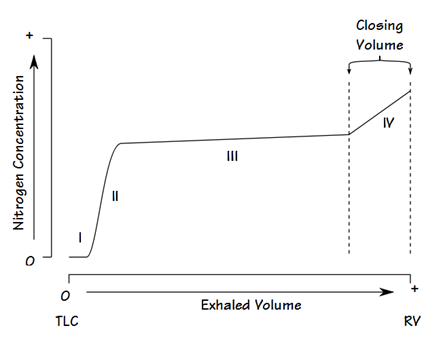
- Basis for phase 4:
- Basal AW closure is indicated by a rise of [N2] from N2-rich apical alveolar gases. Apical alveolar are rich in N2 because:
- During initial inspiration from RV, the first part of inspired gas (which is the anatomical dead space gas rich in N2) goes mainly into the apical alveoli
- Apical alveoli are larger and more poorly ventilated (cf. basal alveoli). Thus, the [N2] of apical alveolar gases are less diluted when breathing in 100% O2
- Basal AW closure is indicated by a rise of [N2] from N2-rich apical alveolar gases. Apical alveolar are rich in N2 because:
Residual volume (RV) cannot be measured directly but is calculated as follows: the FRC is measured using one of three methods: helium dilution, nitrogen washout or body plethysmography. The expiratory reserve volume (ERV) may be measured using standard spirometry. Using the measured FRC and ERV we may calculate RV from the equation: RV = FRC – ERV. Then CC = RV + CV.
Closing capacity is determined by summating “closing volume” and “residual volume”
Source: Bianca’s notes, anaeskey.com
JC 2019
Examiner Comments
2019B 07: 49% of candidates passed this question.
Many candidates confused the factors that affect closing capacity (CC) with factors which affect functional residual capacity (FRC). Some candidates confused airway closure with expiratory flow limitation secondary to dynamic airway compression. A good answer would have included the following: Small airway closure occurs because the elastic recoil of the lung overcomes the negative intrapleural pressure keeping the airway open. Thus, airway closure is more likely to occur in dependant parts of the lung where airways are smaller.
Normally closing capacity is less than FRC in young adults but increases with age. Closing capacity becomes equal to FRC at age 44 in the supine position and equal to FRC at age 66 in the erect position. Closing capacity is increased in neonates because of their highly compliant chest wall and reduced ability to maintain negative intrathoracic pressures. In addition, neonates have lower lung compliance which favours alveolar closure.
Closing capacity is also increased in subjects with peripheral airways disease due to the loss of radial traction keeping small airways open. The consequences of airway closure during tidal breathing include shunt and hypoxaemia, gas trapping and reduced lung compliance. In addition, cyclic closure and opening of peripheral airways may result in injury to both alveoli and bronchioles.
Closing volume (CV) may be measured by the single breath nitrogen washout test or by analysis of a tracer gas such as xenon during a slow exhaled vital capacity breath to residual volume. Residual volume (RV) cannot be measured directly but is calculated as follows: the FRC is measured using one of three methods: helium dilution, nitrogen washout or body plethysmography. The expiratory reserve volume (ERV) may be measured using standard spirometry. Using the measured FRC and ERV we may calculate RV from the equation: RV = FRC – ERV. Then CC = RV + CV.
8. Outline the pharmacology of drugs used to treat asthma.
CICMWrecks Answer
Asthma:
- Airway obstruction that is reversible (completely or partially) either spontaneously or with treatment,
- Airway inflammation (oedema and hypersecretion)
- Increased airway responsiveness to a variety of stimuli
General Measures:
- Oxygen.
- Repeated assessment
- ABGs
Specific Pharmacology:
Class | Example | MoA |
---|---|---|
Adrenergic Agonists | Salbutamol Adrenaline | Predominantly acting at the β2-adrenergic receptor Gs-PRC → ↑AC → ↑cAMP → ↑PLC activity – ↓ [Ca] via ↑ uptake and removal from cytoplasm – Active uncoupling of actin-myosin via i) Phosphorylation of MLCK ii) Phosphorylation of MLCK Phosphatase – ↑ K channel activation → hyperpolarisation → SMC relaxation Mast cell stabilisation (adrenaline) Improved mucocillary function. |
Methyxanthines (PDE3 inhibitors) | Theophylline Aminophylline | Multiple Actions ↓ PDE acitons → ↑ cAMP in bronchial SM → sim to β2 effect Adenosine receptor antagonism → ↓ adensosine related bronchoconstriction |
Antimuscarinics | Tiotropium, Ipratropium | M3 receptor antagonists Normally ACh stimulates M3 → Gq-PRC → ↑ DAG/IP3 → ↑ bronchiolar tone Antagonism → ↓ Vagally mediated Decreased Gq mediated effects |
Steroids | Prednisone, Hydrocortisone | Reverse the activating effect of pro-inflammatory transcription factors → decrease inflammation – Inhibit the formation of cytokines secreted in asthma by T-lymphocytes, macrophages, and mast cells – Decreased vascular permeability – Inhibitory effect on mucus glycoprotein secretion – ? enhance B2 effects. |
Other | Mag Sulphate | Acts as a bronchodilator by decreasing cytosolic Ca2+ concentrations. |
Heliox | Decreased density – thus increased air flow via Hagan Pouiselle | |
Ketamine | Bronchial smooth muscle relaxant by inhibiting Ach mediated SM constriction | |
Sevoflurane | Direct beta agonism and inhibition of histamine release from mast cells. |
Gladwin 2016
Pharmacopeia Table
Examiner Comments
2019B 08: 29% of candidates passed this question.
Answers should have included the most important aspects of the pharmacology of the most commonly used drugs e.g. class, mechanism of action, pharmacodynamics and important adverse reactions. More information on beta-agonists and corticosteroids (mainstays of management) was expected than drugs like magnesium, ketamine and other adjunctive treatments.
9. Compare and contrast the pharmacology of propofol and midazolam.
Examiner Comments
2019B 09: 77% of candidates passed this question.
Highlighting important similarities and differences between the drugs scored higher marks than listing the pharmacology of each drug separately. More pharmacokinetic information was required than simply stating both drugs “are metabolized in the liver and excreted by the kidney”.
10. Describe the principles of capnography, including calibration, sources of error and limitations.
CICMWrecks Answer
Principles
- Beer Lambert law: At a given wavelength, the amount of infrared radiation absorbed by gas is proportional to the concentration of gas present
- CO2 is a heteronucleic molecule, and so absorbs infra-red light
- Infrared light shone across a sample of gas
- Narrow band light emitted from infrared source
- Band frequency chosen which fits the peak absorption frequency of CO2
- 4.23micrometres
- Shone across gas, and absorbed at a detector
- Detector emits signal to analyser -> screen
- CO2 reading outputted is inversely proportional to CO2 present in sample, as per Beer-Lambert Law
- Narrow band light emitted from infrared source
- Gas can be sampled at the patient within the ventilation circuit (in-line), or in a sample of gas diverted away to a separate analysing chamber (sidestream)
Sources of error and limitations
- Sampling
- Entrainment of atmospheric gas if leak in sidestream line
- Occlusion of sidestream line causes loss of gas sampling
- Water condensation absorbs IR light -> erroneously high ETCO2
- Modern capnography includes a water trap and heater (to reduce condensation)
- Calibration
- Incorrect calibration of analyser
- Interference
- Other gases (notably N2O) have a similar absorption spectrum
- Presence may falsely elevate measured ETCO2 (esp. if infrared frequency band too broad)
- Presence of other gases causes ‘collision broadening’
- Absorption spectrum of CO2 is broadened
- Pressure
- Partial pressure, rather than percentage composition, is measured
- If pressure ↓ (e.g. by suction drawing gas into sampling chamber), erroneously low measured ETCO2
- Sampling chamber
- If too large, mixing of gas between respiratory cycles -> compression of waveform and erroneously low measured ETCO2
Mooney 2016
Examiner Comments
2019B 10: 31% of candidates passed this question.
Answers that scored well followed the structure outlined in the question and explained the principles of each component of the question
11. Outline the composition of plasma (50% of marks).
Describe the functions of albumin (50% of marks).
CICMWrecks Answer
Plasma
- Plasma is the liquid (non-cellular) component of blood, in which the red blood cells, white blood cells, and platelets are suspended.
- 93% water, 6% proteins and 1% other solutes
- Makes up 60% of blood volume
- Makes up 18% of extracellular fluid (or 5% of bodyweight)
Plasma Constituents
- Water
- Proteins
- Albumin (60%)
- Globulins (35%)
- α1 (α1 anti trypsin, α1-fetoprotein, serum amyloid A)
- α2 (haptoglobin, ceruloplasmin, Protein C, thyroxin-binding globulin)
- β (transferrin, plasminogen, β2 microglobulin, C-reactive protein)
- γ (immunoglobulins)
- Fibrinogen (4%)
- Regulatory proteins (<1%)
- Coagulation factors
- Complement proteins
- Other Solutes:
- Nutrients – vitamins, glucose
- Gases – oxygen, CO2, nitrogen
- Hormones
- Electrolytes – Na, K, Cl, Mg
- Lipids and other products of metabolism – urea, creatinine, nitrogenous wastes
Functions of Plasma Proteins
- Maintenance of fluid compartments/oncotic pressure
- starling forces, gibbs-donnan control bulk flow.
- Carrier/transport functions
- transferrin → Fe2+; albumin → free fatty acids.
- Role in acid-base balance and CO2 transport
- buffer e.g. carbamino compounds.
- Immunity
- antibodies e.g. IgG.
- Proteolytic enzymes
- complement, kinins, coagulation, fibrinolytic system.
- Metabolism of xenobiotics
- e.g. plasma cholinesterase.
- Anticoagulant proteins
- vWF; antithrombin III.
Functions of Albumin
- Osmotic pressure
- supplies 80% of total plasma Colloid Osmotic Pressure
- retards fluid efflux from plasma and oedema formation
- Transport and metabolism functions
- Transports thyroid hormones
- Transports other hormones, in particular, ones that are fat-soluble
- Transports fatty acids (“free” fatty acids) to the liver and to myocytes for utilization of energy
- Transports unconjugated bilirubin
- Transports many drugs; serum albumin levels can affect the half-life of drugs
- Extra-cellular acid-base buffer
- Detoxification
- solubilizes bilirubin and neutralizes its toxic effects
- Anti-oxidant effects
- Blocks Cu2+-mediated LDL oxidation
- Blocks Free-radical-mediated haemolysis
- anticoagulant effect
- protein store – for signalling molecules and nitric oxide
- Immunomodulation
- Other
- Competitively binds calcium ions (Ca2+)
- Prevents photodegradation of folic acid
- marker of an inflammatory state
- prevents apoptosis of proximal renal tubular cells
- stimulates proliferation of proximal renal tubular cells
JC / Gladwin 2020
Examiner Comments
2019B 11: 30% of candidates passed this question.
A good answer began with a definition of plasma and then listed the components – water, albumin, globulins, fibrinogen and other proteins before mentioning the lipid content, nutrient content, wastes and electrolytes. Frequently the breakdown of the globulin component was inaccurate. A common omission was dissolved gas components. Descriptions of the calculation of oncotic pressure and GFR were not asked and hence did not attract marks. The functions of albumin may be subdivided into: Osmotic pressure, transport function, acid-base buffer, anti-oxidant, anticoagulant effect, protein store, metabolism and ‘other’.
12. Define pain. Outline the processes by which pain is detected in response to a peripheral noxious stimulus.
CICMWrecks Answer
Pain is an unpleasant sensory and emotional experience, associated with actual or potential tissue damage, or described in terms of such damage
Anatomical and physiological components of the response to pain
- Primary afferent nociceptors
- Pseudo-Unipolar
- Cell body in dorsal root ganglion
- Free nerve endings or specialized terminal structures
- Pacinian corpuscles
- Location
- Skin
- Connective tissue
- Muscle
- Blood vessels
- Viscera
- Responds to simuli
- Mechanical
- Chemical
- Thermal
- Pseudo-Unipolar
Nociception
- Transient Receptor Potential (TRP) Ion Channels
- Capsaicin
- Heat
- Acid
- Inflammation
- Ischaemia
- Acid-Sensing Ion Channel (ASIC)
- 5-HT3 receptors
- On activation of nociceptors
- Na channels open
- Depolarization from < -60mV to -40mV
- Rapid membrane depolarization
- Voltage Dependent Calcium Channels (VDCC) open and increase intracellular Ca
- Substance P and Calcitonin Gene-Related Peptide (CGRP), Neurokinin A released in response to Ca
- Mediate inflammation → macrophages, neutrophils, mast cells activated
- Increase excitability of sensory and sympathetic fibres
- Vasodilation, extravasation of plasma proteins
- Inflammatory cells release substances
- Histamine
- Bradykinin
- Serotonin
- Nitric Oxide
- Peripheral sensitization → Primary hyperalgesia
- to dampen pain response
- Silent nociceptors – Do not respond to external stimulus, however activated by inflammatory mediators
- Voltage-gated Potassium Channels stabilize the membrane potential
Signal Pathway
- Signal transduced via C fibres (0.5~2m/sec) or Aδ fibres (6~30m/sec) to dorsal horn
- Aδ activation → short, pricking pain
- C activation → burning, aching pain
- Primary afferent enters spinal cord at the spinal level, or above or below the spinal level via lissauer tracts and synapse with interneurons
- Via C fibres or Aδ fibres
- Signal relay via spinal interneurons
- Signalling via Glutamate and NMDA or AMPA receptors
- Nociceptor-specific
- Respond only to pain
- Wide Dynamic Range (WDR)
- Respond to nociceptors and other stimulus
- Central sensitization → allodynia
- Inhibitory interneurons and descending pathways modulate pain
- “Gate control theory of pain” → Aβ fibres carrying signals from benign stimuli inhibit noxious interneuron transmission
- Signal transduction to thalamus via anterolateral spinothalamic tract
- Small but significant proportion of signal transduction also occurs ipsilaterally
- Signal distributed to cerebral cortex via thalamus
- Putamen
- Hypothalamus
- Amygdala
- Periaquaductal grey matter
- Hippocampus
- Cerebellum
- Somatosensory cortex
Descending inhibitory pathways
- Noradrenaline
- Serotonin
- Substance P
- CCK
- GABA
Sakurai 2016
Examiner Comments
2019B 12: 33% of candidates passed this question.
Starting with the WHO definition of pain, followed by a brief description of the nature of noxious stimuli (thermal, mechanical, chemical) then proceeding to mention the nature of the cutaneous receptors would have been a very good start to this question. Following this, a description of the various substances involved in pain (K, prostaglandins, bradykinin, serotonin, substance P) and outlining the types of nerve fibres involved in pain transmission and how they synapse in the spinal cord and cortex was expected. The presence and nature of the descending inhibitory pathways was mentioned by very few.
13. Describe the exocrine functions of the pancreas.
CICMWrecks Answer
Exocrine pancreas
- produces 1.5L/day pancreatic juice
- Pancreatic juice – digestive enzymes and bicarbonate
- Enzymes: synthesized and secreted from the exocrine acinar cells
- Bicarbonate: secreted from epithelial cells lining small pancreatic ducts
Contents
- Digestive Enzymes:
- Proteases: trypsin and chymotrypsin: proteolysis
- Pancreatic Lipase: Hydrolysis of triglycerides
- Amylase: Hydrolysis of carbohydrates
- Other Pancreatic Enzymes: Ribonuclease, deoxyribonuclease, gelatinase and elastase
- Bicarbonate and water:
- Bicarbonate neutralizes acid coming into small intestine from stomach
- Produced by carbonic anhydrase → secreted into the lumen of the duct → pancreatic juice
Control of pancreatic exocrine secretions
- Vagus nerve:
- Low level stimulus via Ach in response to anticipation of meal
- Enteric endocrine system:
- Cholecystokinin:
- secreted in duodenum
- Stimulated by partially digested proteins and fats
- CCK released into blood → binds to pancr acinar cells
- secreted in duodenum
- Secretin:
- Secreted in proximal small intestine
- In response to acid in duodenum
- Stimulates duct cells to secrete water and bicarb
- Secreted in proximal small intestine
- Gastrin:
- Secreted by stomach
- in response to gastric distension and irritation
- Stimulates acinar cells to secrete digestive enzymes
- Secreted by stomach
- Cholecystokinin:
Occurs in three phases: cephalic → Gastric → Intestinal
JC 2019
Examiner Comments
2019B 13: 33% of candidates passed this question.
Most candidates were able to mention some pancreatic enzymes, though often in insufficient detail to attract full marks. The amount, type, pH, etc. of pancreatic secretions was often not included. Many candidates did not describe the stimuli for pancreatic secretion. Better answers described the cephalic, gastric and intestinal phases of pancreatic secretion.
14. Outline the classification and effects of beta-blocking drugs with examples (50% of marks).
Compare and contrast the pharmacokinetics of metoprolol with esmolol (50% of marks).
CICMWrecks Answer
Beta receptor antagonists (Beta blockers)
- bind to beta-adrenoceptors and block the binding of noradrenaline and adrenaline
- Inhibits normal sympathetic effects that act through these receptors (Sympatholytic)
- mostly competitive antagonists, there is some evidence of partial agonist activity (Labetalol)
- Some (partial agonists) show intrinsic sympathomimetic activity (ISA): partially activate receptor + prevent noradrenaline from binding to the receptor.
- Some possess membrane stabilizing activity (MSA) similar to sodium-channels blockers
- variable specificity for beta 1 versus beta 2 receptors
- important clinically due to different effects
- often dose related, may be B1 selective at low doses but non-selective at higher
- lipid solubility determines speed of onset
- most resemble isoproterenol
Effects of β-adrenergic blockade
Type | Main site of action | Effects of β-adrenergic blockade |
---|---|---|
β1 | Heart | Anti-ischemic effect: β1 blockade → ↓ heart rate and ↓ cardiac contractility → ↓ BP and ↓ oxygen consumption by the heart → anti-ischemic effect |
Antiarrhythmic effect: β1 blockade → ↓ AVN conduction, ↑ AVN refractory time, and ↓ heart rate → anti-arrhythmic effect | ||
Anti-remodeling effect | ||
Kidneys | β1 blockade of the juxtaglomerular cells → ↓ renin release → ↓ angiotensin II conversion → ↓ H2O resorption → ↓ BP | |
β2 | Smooth muscle | Vasculature: vasoconstriction Bronchioles: bronchoconstriction |
Ciliary body of the eye | ↓ Aqueous humor production → ↓ intraocular pressure | |
Pancreatic beta cells | ↓ Insulin release → hyperglycemia and new-onset diabetes | |
Skeletal muscle | ↓ Glucose uptake (↓ insulin sensitivity) | |
Liver | ↓ Hepatic glycogenolysis → hypoglycemia (esp. in diabetics) | |
Lipoprotein lipase enzyme | Inhibits lipoprotein lipase → ↑ triglycerides and ↓ HDL → hyperlipidemia | |
β3 | Adipose tissue | ↓ Lipolysis → weight gain |
Classification
- β1-selective (cardioselective):
- e.g. esmolol, nebivolol, bisoprolol, atenolol, metoprolol
- is used for rate control in tachycardia and hypertension management
- side effects: hypotension, heart block, bronchoconstriction at higher doses
- Non-selective β-blockade
- e.g. propranolol
- used for hypertension, to reduce bleeding risk in oesophageal varices, tremor, and as migraine prophylaxis. It is the treatment of choice in thyrotoxicosis as it stops conversion of T4 to T3, reduce ocular pressure in glaucoma
- side effects: rapid withdrawal may precipitate tachycardia, can cause bronchoconstriction and is not recommended in patients with obstructive respiratory disease, issues with hypoglycaemia
- Non-selective α- and β-blockade
- e.g. labetalol, carvedilol
- Potent vasodilators because of their α-blocking action
- Improve endothelial function and vascular re-modelling
- Others
- e.g. sotalol : also acts on K+ channels as class III antiarrhythmic
METOPROLOL | ESMOLOL
PHARMACOKINETICS
Metoprolol | Esmolol | |
relatively selective beta blocker with no intrinsic sympathomimetic activity. | Cardio-selective beta blocker with rapid onset and offset. | |
PK – A | bioavailabilty Absoption is rapid and complete, however there is extensive first pass metabolism. BA 50% routes of admin PO or IV dose Oral in 12.5mg increments, IV in 1-2mg boluses | bioavailabilty Only available as IV therefore 100% routes of admin IV dose In 10mg increments titrate to effect |
D | volume of distrib 5.5 L/Kg protien binding 10-20% to albumin lipid solubility is high so it crosses the BBB | volume of distrib 3.5 L/Kg protien binding 60% to albumin lipid solubility is high so it crosses the BBB |
M | hepatic or renal Extensively hepatic via CYP2D6 | neither hepatic or renal! by red blood cell esterases to a mostly inactive metabolite |
E | half life 3-8hours excretetion In urine 5-10% unchanged | half life 10 minutes excretion In urine |
Sources: CVPharmacology
JC 2019
Examiner Comments
2019B 14: 47% of candidates passed this question.
Beta-blocking drugs were generally well classified. Selectivity, membrane stabilising activity and ISA should have been mentioned. Many candidates omitted or poorly answered the ‘effects’ of 6 beta blockers. Candidates who performed well answering the pharmacokinetics of metoprolol and esmolol provided a table of the two drugs. Superficial statements such as “hepatic metabolism and renal excretion” attracted minimal marks. The mechanism of action of beta blockers was not requested.
15. Define clearance and hepatic extraction ratio (30% of marks).
Describe the role of the liver in drug clearance with examples (70% of marks).
CICMWrecks Answer
Clearance (Cl)
Defined as the volume of plasma from which drug is completely removed per unit time (units – mL/min)
- Drug can be cleared from plasma via two routes:
- “Elimination” from the body – Drug excreted unchanged (via renal or biliary routes) and/or metabolised (by liver or other organ)
- Non-compartmental model: Cl = Dose / AUC
- Compartmental model: Cl=Vd x Kel = Vd x (ln2/t1/2)
- “Intercompartmental clearance” (* occurs only in multi-compartment model *) – Drug distributes from the central to peripheral compartment(s) → determined by the rate constant for intercompartmental transfer (k12, k21; k13, k31; Etc.)
- “Elimination” from the body – Drug excreted unchanged (via renal or biliary routes) and/or metabolised (by liver or other organ)
- Significance of clearance:
- Determines maintenance dose rate needed to achieve a plasma [drug] at steady state
- “Maintenance dose rate” = Cl x desired [ ]PLASMA
Hepatic Extraction Ratio
Hepatic extraction ration (HER) – Fraction of drug that is irreversibly removed during 1st pass of blood through the liver → determined by intrinsic clearance (enzyme activity) and (ii) unbound % of drug
where
QH = Hepatic Blood Flow
ERHep = Hepatic Extraction Ratio
FU = fraction of drug unbound in plasma
ClInt = hepatic enzymatic capacity
Hepatic clearance
“Hepatic clearance” → determined by Hepatic Blood Flow and Hepatic Extraction Ratio
- Hepatic blood flow (HBF) – Rate at which drug is delivered to the liver
- Hepatic extraction ration (HER) – Fraction of drug that is irreversibly removed during 1st pass of blood through the liver → determined by intrinsic clearance (enzyme activity) and (ii) unbound % of drug
Hepatic clearance = HBF x HER = HBF x [(% unbound) x (intrinsic clearance)]
Important to note:
- Drugs with HER > 0.7 (↑ enzyme activity or “flow-limited”), such as GTN:
- Hepatic drug clearance is dependent on HBF (“perfusion-dependent elimination”)
→ ↑ HBF will lead to ↑ hepatic drug clearance - Changes in HER (intrinsic enzyme activity or unbound %) have minimal effect on hepatic drug clearance as heaps of drug is already removed at a given time
– i.e. Hepatic clearance ≈ HBF
- Hepatic drug clearance is dependent on HBF (“perfusion-dependent elimination”)
- Drugs with HER < 0.3 (↓ enzyme activity or “capacity-limited”), such as diazepam:
- Hepatic drug clearance is dependent on protein binding and intrinsic enzyme activity (“capacity-dependent clearance”)
→ ↑ enzyme activity and/or ↓ protein binding will ↑ hepatic drug clearance - Changes in HBF will have minimal effect on hepatic drug clearance as only a small % of drug is ever removed at a given time
– i.e. Hepatic clearance ≈ HER = (unbound %) x (intrinsic clearance)
- Hepatic drug clearance is dependent on protein binding and intrinsic enzyme activity (“capacity-dependent clearance”)
Other roles of liver in drug pharmacokinetics
Absorption (First pass metabolism):
- Drugs absorbed from GIT (except buccal and rectal mucosal) enter portal venous blood and pass through liver before entering systemic circulation
- They are metabolised by enzymes within the (i) liver (main) and (ii) gut wall (minor)
- FPM is a main reason why plasma [ ] after an oral dose is less cf. similar IV dose → as a result, it is a key determinant of oral bioavailability
- Significance – Drugs with ↓ FPM are either well-absorbed, stable in GIT, and/or have minimal hepatic metabolism → thus, have ↑ oral bioavailability (and ↑ plasma [ ]). The opposite is true for drugs with ↑ FPM
where,
FB = Bioavailable fraction
FA = Fraction absorbed
FG = Fraction remaining after gut mucosal metabolism
FH = Fraction remaining after hepatic metabolism
Metabolism:
Metabolism → process of chemically altering a drug within the body
Mainly occurs in liver (by hepatic microsomal enzymes), and few other sites
Effects of metabolism:
- ↓ drug activity (main effect): converts a “pharmacologically active” form of drug (Ie. non-polar and lipid soluble) into a “pharmacologically inactive” form (Ie. more polar and water-soluble) that can be excreted from the body (esp in bile or urine)
- ↑ drug activity: “Prodrug” → active moiety (Eg. enalapril → enalaprilat; parecoxib → valdecoxib)
- Produce metabolites with equal activity to parent compound (Eg. diazepam, propranolol)
Phases of metabolism:
- Phase I (functionalisation or non-synthetic):
- Alter drug reactivity for phase II reaction and to ↑ drug polarity/water-solubility
- Oxidation Including Hydroxylation (Eg. propofol), desulphation (Eg. STP), dealkylation (Eg. vecuronium), dehalogenation (Eg. volatiles), deamination, Reduction, Hydrolysis
- Phase II (conjugation or synthetic)
- ↑ water solubility of drug or its metabolite by conjugating it to a polar endogenous moiety (Eg. sulphate, glucuronyl, methyl, Etc.) → permits excretion in urine or bile
- Glucuronidation via glucuronosyltransferase (Eg. morphine, propofol)
- UDP-glucuronic acid is conjugated to the drug → conjugate is inactive and water-soluble → excreted in urine/bile
- Conjugated undergoes “enterohepatic recirculation” if eliminated in bile → intestinal bacterial glucuronidases hydrolyses glucuronide → liberates free drug which is reabsorbed back into circulation → results in prolonged drug action
- Other reactions: Sulphation, Acetylation, Methylation, Glutathione via glutathione-S-transferase (Eg. EtOH)
- Note – All these reactions involve non-microsomal enzymes, EXCEPT for glucuronidation (requires hepatic CYP450 microsomal enzymes)
Source: Bianca’s notes
JC 2019
Examiner Comments
2019B 15: 70% of candidates passed this question.
Clearance was generally well answered. It is the volume of plasma cleared of a drug per unit time, not the mass of drug cleared.
An equation was helpful in identifying the relevant components of hepatic clearance. ClHep=QH X ERHep ERHep= FU x ClInt / QH + FU x ClInt QH = hepatic blood flow ERHep = hepatic extraction ratio FU = fraction of drug unbound in plasma ClInt = hepatic enzymatic capacity
Many candidates did not describe the effects of hepatic blood flow and intrinsic clearance on drugs with high and low hepatic extraction ratios. Some discussion of Phase I and II reactions was also expected.
16. Compare the structure, function and coronary circulation of the right and left ventricles.
CICMWrecks Answer: Anatomy (Rt vs Lt)
RIGHT
VENTRICLE
LEFT
VENTRICLE
Pathway | |
RA → tricuspid valve → RV → pulmonary valve → Pulmonary trunk | LA → Mitral valve → LV → Aortic valve → Aorta |
Valves | |
TV: 3 triangular cusps (medial anterior, inferior) attached by the base to fibrous ring of TV orifice. Papillary muscles connect trabeculae to cusps PV: 3 cusps (posterior, right anterior, left anterior) | MV: large anterior and small posterior cusp Attach to 2 large papillary muscles (anterolateral, posteromedial) by chordae tendinae AV: 3 semilunar cusps (Rt posterior, left posterior, anterior). Aortic sinuses above |
Portions | |
Septal portion: 1) the inflow tract: supports the tricuspid valve, Inferior aspect have ‘trabeculae carnae’ 2) Infundibuloventricular crest: muscular, lies between AV and pulmonary orifices – separates inflow and outflow 3) the outflow tract (infundibulum) – smooth walled, directed up+right towards pulm trunk | Portions: 1) Large trabeculated sinus portion with MV 2) Small smooth outflow tract that supports AV located anterior to the anterior mitral leaflet and is part of the atrioventricular (AV) septum. |
Rest | |
Rest of RV wall is trabecular Moderator band: muscular bundle crossing ventricular cavity from Intrerventricular septum to anterior wall – conveys right branch of AV bundle to the ventricular muscle | Wall: thick trabeculae carnae mostly. Exception of fibrous vestibule immediately below aortic orifice The free wall and apical half of the septum contain fine internal trabeculations. |
Dimension | |
The wall of the right ventricle is thinner than that of the left (1/3rd) Thickest at base, thinner towards apex Cavity size 85ml (=LV) | Wall 3x the size of RV Cavity size equal |
Functions | |
Receives deoxygenated blood from RA Pumps into pulmonary circulation | Receives oxygenated blood from LA Pumps into aorta → systemic circulation |
Main Function | |
maintain adequate pulmonary perfusion pressure to deliver desaturated venous blood to the gas exchange membranes of the lungs maintain low systemic venous pressure to prevent tissue and organ congestion | Maintain adequate systemic perfusion pressure to deliver oxygenated blood to tissue |
Pressures | |
RV Sys 15-30 | LV Sys 100-140 |
RV Dias 4-12 | LV Dias 3-12 |
RVEDD 10-26mm | LVEDD 36-56mm |
RVESD 10-26mm | LVESD 20-40mm |
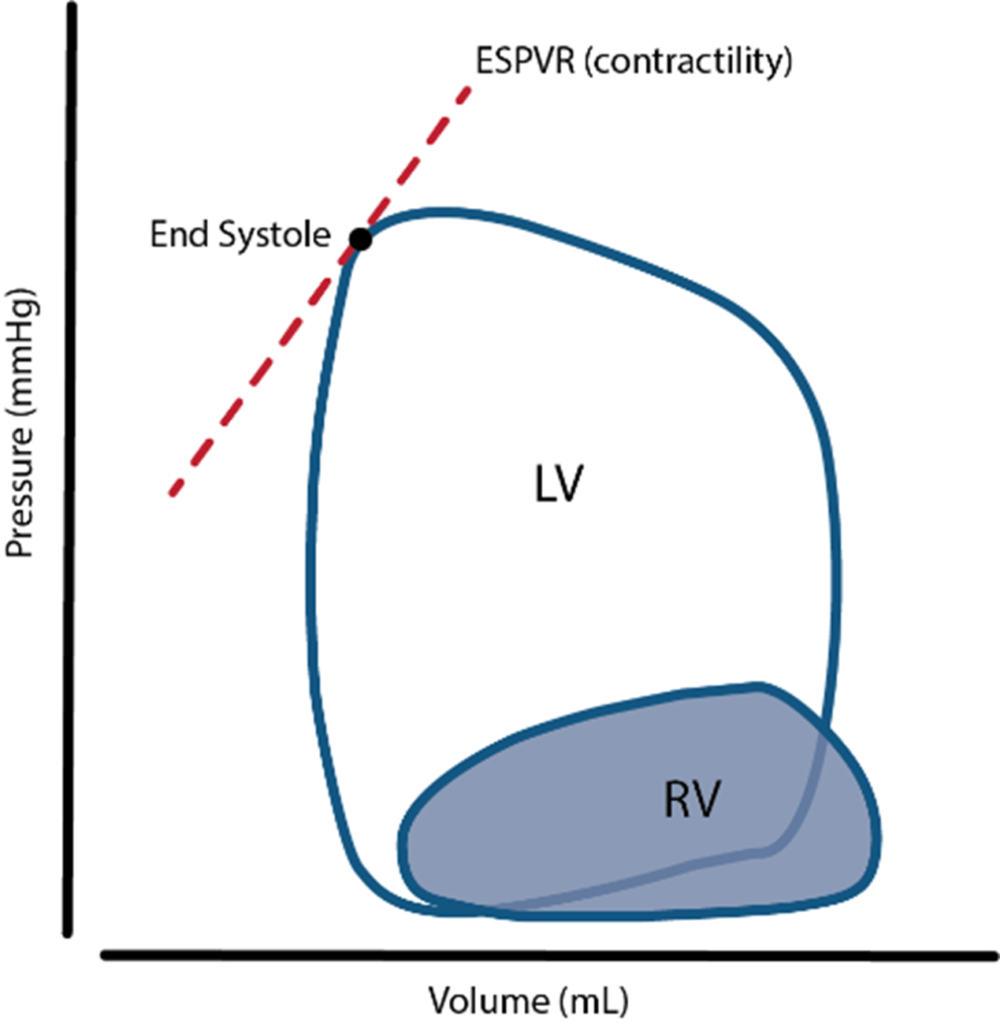
Sources: Last’s Anatomy, Aneskey.com, Anatomy for anaesthetists
JC 2019
CICMWrecks Answer: Coronary Circulation (Rt vs Lt)
RIGHT
VENTRICLE
LEFT
VENTRICLE
- Supply:
- Right coronary artery and its branches
- conus artery supplies the infundibulum
- acute marginal arteries supply the anterior free wall
- posterior descending artery via septal branches supply the posterior 1/3 IV septum (PDA arises from RCA in Right dominant circulation and LCx in Left dominant circulation
- the other 2/3 of the IV septum is supplied by septal branches of the left anterior descending artery
- Supply: Left Main Coronary
- LAD (supplies the free wall and most of the papillary muscles)
- Diagonals
- Septal perforators
- LCx: inferolateral LV wall
- Obtuse Marginal branches
- LAD (supplies the free wall and most of the papillary muscles)
- RV Coronary blood flow
- RV pressures are low during both systole and diastole.
- flow during both systole and diastole
- LV Coronary blood flow
- The pressure inside the left ventricle is slightly higher than in the aorta during systole.
- Flow predominantly during diastole.
- Subendocardial flow ceases during systole.
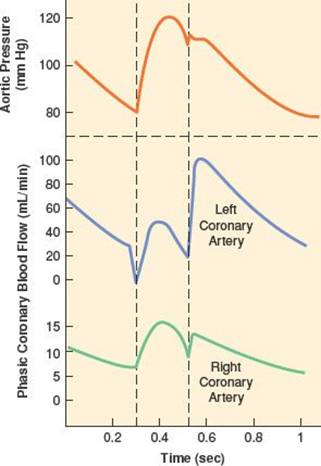
Sources: Last’s Anatomy, Aneskey.com, Anatomy for anaesthetists
JC 2019
Examiner Comments
2019B 16: 27% of candidates passed this question.
The question sought information on the structure (anatomy), function (physiology) and vascular supply of the right and left ventricle. Good answers provided detail in each section e.g. values for ventricular pressure rather than simply stating “high- and low-pressure systems”. Many marks may be gained by a simple anatomical description & labelled PV loop for each ventricle. Many candidates focussed solely on the coronary circulation, to which only a proportion of the marks were allocated.
17. Explain respiratory compliance and outline the factors that affect it.
CICMWrecks Answer

Lung compliance
- Change in lung volume per unit change in transmural pressure
- Normal lung compliance ~200ml/cmH2O
- Static compliance (Cs)
- ” Compliance of lung measured when lung held at constant lung volume”
- no pressure componet due to resistance
- is a function of: Elastic recoil of the lung, Surface tension of alveoli
- Dynamic compliance (Cd)
- ” Compliance of lung measured during cycles of inspiration and expiration”
- includes the pressure required to generate flow by overcoming resistance forces
- is a function of: respiratory rate
- Dynamic Compliance usually < Static Compliance due to the degree of time dependence in the elastic behaviour of a particular lung
- Specific compliance
- Compliance per unit volume lung
- Used to compare different lungs
- Hysteresis
- any process where the future state of a system is dependent on its current and previous state
- Compliance of the lung is different in inspiration and expiration
- In dynamic compliance curves:
- Airways resistance is a function of flow rate. Flow rate (therefore resistance) is maximal at the beginning of inspiration and end-expiration.
- In static compliance curves:
- There is no resistive component. Hysteresis is due to viscous resistance of surfactant and the lung.
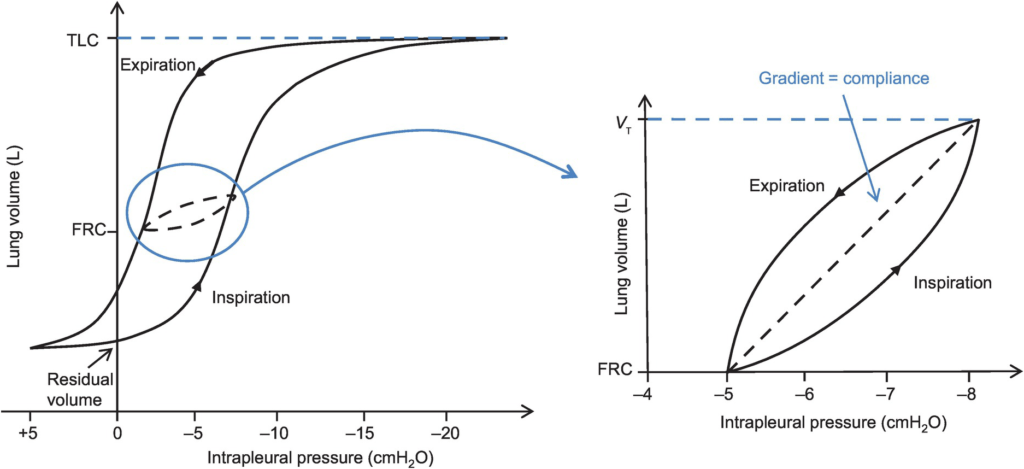
Factors Affecting Compliance
Lung Compliance
- Surfactant
- increases lung compliance
- decreases surface tension at alveolar air – water interface
- prevents small alveoli from collapsing
- accounts for most of hysteresis in intact lungs
- Lung volume
- Lung Compliance decreases at higher lung volumes
- Specific compliance (Compliance/FRC) remains constant
- Elephants have greater lung compliance than mice!
- Pulmonary blood volume
- Increased PBV decreases lung compliance
- Pulmonary venous congestion from L heart failure or mitral regurgitation
decreases lung compliance
- Bronchial smooth muscle tone
- Increased bronchial smooth muscle tone decreases compliance
- Decreased dynamic lung compliance by 50% in animal models of methacoline
challenge
- Disease
- ARDS, pneumonia decreases lung compliance
- pulmonary fibrosis → Impraired elasticity → decreases lung compliance
- Asthma, Emphysema increases lung compliance
Chest Wall Compliance
- Chest wall restriction – reduced chest wall compliance
- Obesity
- Spastic paralysis of chest wall musculature
- Ossification of costal cartilages
- Kyphosis/scoliosis
- Scarring/constriction (e.g. circumferential burns)
- Position: Prone (60% reduced compliance)
- Collagen Disorders: Increased Chest Wall Compliance
Measurement of Compliance
Dynamic Compliance
- ” Compliance of lung measured during cycles of inspiration and expiration”
- Measure:
- Measure with spirometer
- Using Volume/Pressure curve during normal rhythmic breathing at points of no flow
- Equation above then gives dynamic compliance
Static Compliance
- ” Compliance of lung measured when lung held at constant lung volume”
- Volume vs Pressure loops during inspiration and expiration demonstrate Hysteresis
- Measure:
- @ no flow in or out of the lung, AND time for pressure to equlibrate across the lung (>10sec).
- Patient exhales in steps holding the volume with open glottis
- Intrapleural pressure measured as oesophageal pressure
- Measured compliance secondary to viscoelastic properties and accounts for both short and long time-constant alveoli.
Specific Compliance
- Measure of the average compliance across all lung units
- Children = Adults ~ 0.05 cmH2O-1
Gladwin / Sakurai / JC 2020
Examiner Comments
2019B 17: 51% of candidates passed this question.
Answers were generally well structured. Better answers described lung and chest wall compliance and the pressures which are used to calculate compliance. Better answers displayed an understanding of dynamic, static and specific compliance and provided a reasonably comprehensive list of the physiological factors affecting chest and lung compliance.
18. Compare and contrast the pharmacology of metaraminol and noradrenaline.
Examiner Comments
2019B 18: 71% of candidates passed this question.
Marks were distributed across pharmaceutics, uses, dose & administration, mechanism of action, Pharmacokinetcs and Pharmacodynamics. Common omissions were doses/rates of infusion, effects other than on heart/SVR (e.g. splanchnic, renal blood flow), indirect effect of metaraminol, receptor effect of noradrenaline other than alpha 1 and tachyphylaxis.
19. Describe the pharmacology of atropine.
Examiner Comments
2019B 19: 53% of candidates passed this question.
Most candidates used a good structure to compose their answer. Better candidates understood that CNS effects occur as atropine is a tertiary amine that crosses the blood brain barrier. The mechanism of action was required. Indications for use should have included bradycardia, organophosphate poisoning, drying of secretions etc. Reasonably extensive details regarding pharmacodynamics was expected, including potential toxic effects. There was limited knowledge regarding pharmacokinetics.
20. Compare the pharmacology of piperacillin-tazobactam and ciprofloxacin.
Examiner Comments
2019B 20: 58% of candidates passed this question.
This question was most effectively answered using a tabular format. Only a minority of candidates demonstrated a comprehensive knowledge of these level 1 drugs and very few candidates compared the two in areas which lent themselves to comparison. The spectrum of activity generally lacked detail. Few candidates mentioned that piperacillin-tazobactam had superior gram-positive cover, both have extensive gram-negative cover including Pseudomonas.
Piperacillin-tazobactam is effective against anaerobes; whilst ciprofloxacin has some atypical cover against Mycoplasma. The mechanism of action was generally well described for piperacillin; many candidates incorrectly stated the mechanism of action for ciprofloxacin, confusing the drug with a macrolide.
Better answers included time- dependant and concentration-dependent killing. The concept of half-life was frequently confused with the dosing interval.
Minimal marks were awarded for “allergy” and “gastrointestinal side-effects”. Better candidates mentioned Liver function derangement, neutropenia, interstitial nephritis for piperacillin and tendonitis for ciprofloxacin.
VIVAs
A. Pharmaceutics | |
B. Pharmacokinetics | |
C. Pharmacodynamics | Drug action, graph Pharmacology dose-response curves, Oxygen |
D. Variability in Drug Response | |
E. Cellular Physiology | |
F. Respiratory | Monro-Kellie doctrine Spirometry |
G. CVS | Structure activity relationships: catecholamines P-V loop: Mechanical events Contractility |
H. Renal | Renal handling of sodium Functions of kidney |
I. Body Fluids and Electrolytes | 5L NS on acid base Total body potassium regulation |
J. Acid Base | Acid base balance, anion gap Buffer systems ABG interpretation (pH 7.27 pCO2 55 pO2 144 HCO3 24, BE 1), CO2 ABG interpretation (pH 7.50 pCO2 30 pO2 55 HCO3 22, BE -2), on 6L HM |
K. Neuro | PD of sedative drugs. Propofol 100mg: Bolus vs Slow infusion over 5 minutes Cerebral blood flow Opioid pharmacology |
L. Musculoskeletal | Pharmacology: NMBDs, LA |
M. ANS | Autonomic nervous system |
N. Liver | Blood supply of liver |
O. GIT | |
P. Nutrition and Metabolism | Basal metabolic rate, thyroid hormone |
Q. Haematology | Coagulation after tissue trauma, arterial pressure |
R. Thermoregulation | |
S. Immunology | |
T. Microbiology | |
U. Endocrine | Glucose metabolism, movement from intestinal lumen to blood |
V. Obstetrics | |
W. Measurement and Monitoring | |
X. Procedures |
Recent Comments