SAQs
1. Describe the surface anatomy of the anterior neck (30% of marks) and the underlying structures relevant to performing a tracheostomy (70% of marks).
CICMWrecks Answer
Tracheostomy – insertion of a tube through the anterior portion of the neck into the trachea to facilitate ventilation
Trachea Course:
- Larynx connects to the superior part of the trachea at C6 into the thorax and terminates at the level of the sternal angle, where it divides into the right and left mainstem bronchi.
- Initially anterior, then moves posteriorly as it descends to move behind the sternal notch
Tracheal Structure:
- A fibrocartilaginous tube 10cm long, approx 5cm in neck
- Supported by incomplete cartilaginous tracheal rings, which keep the trachea patent.
- The tracheal rings are joined by fibroelastic tissue.
- They are deficient posteriorly where the trachea lies anterior to the oesophagus; the posterior gap is spanned by the involuntary smooth trachealis muscle
Relationships:
- Lateral – carotid sheaths (common carotid arteries, vagus and internal jugular veins), thyroid lobes, inferior thyroid arteries, recurrent laryngeal nerves
- Inferior to the isthmus of the thyroid gland are the inferior thyroid veins
- Posterior – oesophagus, vertebral column
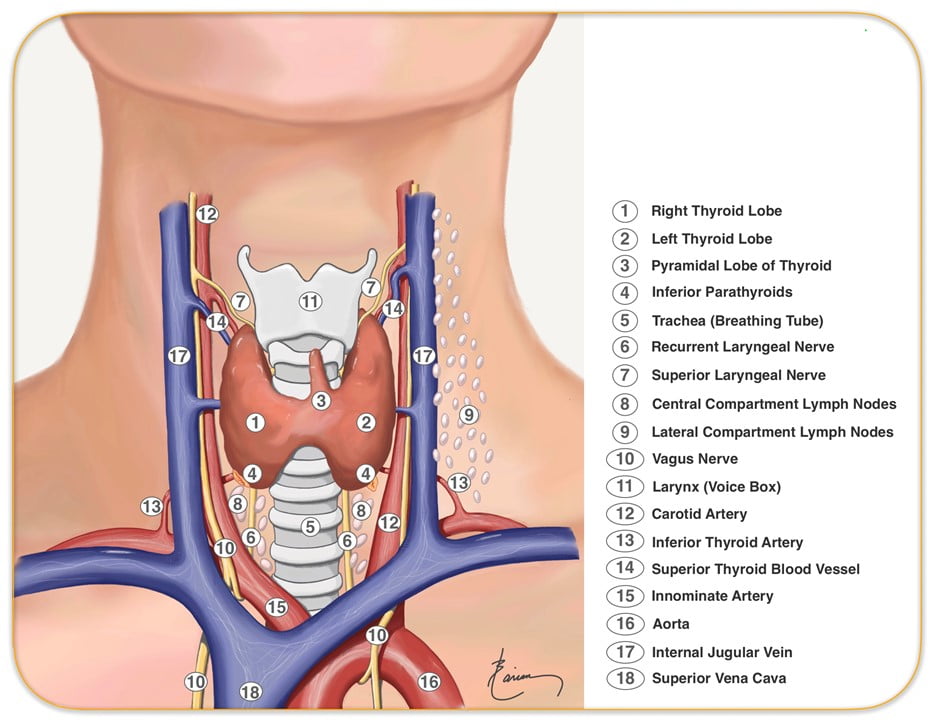
Relevant surface anatomy (in midline of neck):
- Hyoid bone (at level of C3)
- Thyroid cartilage
- Cricothyroid membrane
- Cricoid cartilage (at level of C6)
- Thyroid gland
- Sternohyoid muscle just lateral to midline structures, overlies sternothyroid and thyrohyoid muscles
Layers of dissection in tracheostomy:
- Skin
- Subcutaneous tissue
- Fat
- Pretracheal fascia (superficial and deep)
- Passage through the fibroelastic tissue in between the 1st and 2nd rings (common in perc trache) or 2nd /3rd or 3rd/4th (surgical trache)
- Trachea
Gladwin 2016
Examiner Comments
2018B 01: 79% of candidates passed this question.
Answers required a description of the surface anatomy outlining the midline structures including the hyoid bone and cartilages. The tissue layers should have been mentioned as should the relevant tracheal anatomy. The anterior, posterior and lateral relations of the trachea should also have been included along with the relevant nerves and blood vessels. Diagrams were not essential but could have been included.
Candidates should note that marks were not awarded for a description of how to perform a tracheostomy.
2. Compare and contrast amiodarone and digoxin.
Examiner Comments
2018B 02: 82% of candidates passed this question.
Most candidates had a good structure for answering this question; a table was commonly used.
Marks were awarded for indications and an explanation of the mechanism of action of both drugs, which was generally well explained. The pharmacodynamic effects were often listed in a general manner and more detail would have achieved a higher mark, including a list of the ECG effects. Some detail on the pharmacokinetics and adverse effects of the drugs was expected and this section was generally well answered. Better answers noted digoxin levels and potential drug interactions.
3. Explain the causes of the differences between measured end tidal and arterial partial pressures of carbon dioxide (CO2).
CICMWrecks Answer
PaCO2
- Factors affecting PaCO2
- Rate of metabolism and CO2 production
- FiCO2 (usually negligable)
- Alveolar ventilation
- Increased alveolar ventilation decreased PaCO2
End-Tidal CO2
- CO2 contained in gas at the end of tidal expiration
- Contains:
- Alveolar CO2
- High CO2 diffusion – PACO2 = PaCO2
- Only 0.7mmHg Alveolar-arterial CO2 gradient for 10% shunt
- Alveolar Dead Space CO2
- Anatomical – conducting airways
- Physiological – West zone 1 / Apex
- Varies with posture and pathology
- Alveolar CO2
- Measured by capnometer
- In-line or side-stream
- Used IR absorbance at 4.23 μm
- Side-stream: lag in CO2 detection due to increased dead space between respiratory tract and analyzer
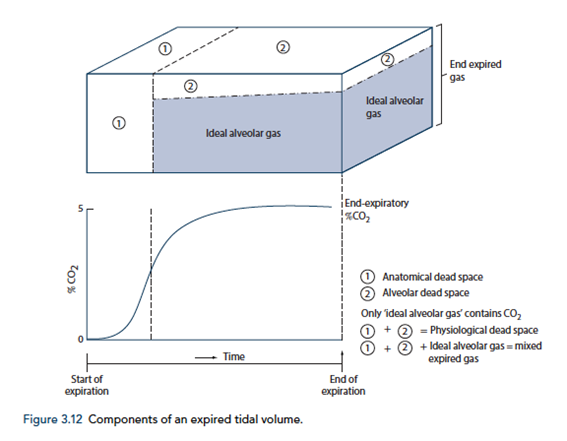
End-tidal to Arterial PaCO2 difference
- Normal ETCO2 – PaCO2 ~5mmHg
- Can be due to Artificially low ETCO2 or Artificially High PaCO2
Artificially low ETCO2
- Dead space (VD)
- Assumed PCO2 however usually low pCO2 but non 0 pCO2
- Contributes to ETCO2-PaCO2 ~5mmHg
- Pathological ↑VD
- ↑alveolar dead space (normal individuals = 0)
- Mixing of gas between perfused and non-perfused alveoli → ETCO2 < PaCO2
- Equivalent to ↑ West Zone 1
- → PE, hypotension, excess PEEP/IPPV
- COAD – poor perfusion of hyper-expanded alveoli
- ↑alveolar dead space (normal individuals = 0)
- Closing capacity (CC):
- Usually 40 CC may exceed FRC
- ↑ Airways collapse/gas trapping → airways closure → ↑alveolar pCO2 within alveoli not exhaling
- Exacerbated by
- low volume ventilation close to FRC
- High FiO2 for extended periods → denitrogenation → loss of airways splinting = CC closer to FRC
- Usually 40 CC may exceed FRC
- Sampling line problems:
- Too far from trachea (ie ↑ mechanical dead space)
- Too long – unable to adequately sample as gas is trapped in line
- Air leakage/line blockage leading to measurement error with entraining of room air, loss of expired air
- Machine:
- Loss of calibration of sampling unit
- Excess H2O in water trap interfering with measurement
- Interference from other gases (N2O) artificially raises ETCO2 due to interference with IR absorber “Collision broadening”
- Incorrect timing of measurement
- Inadequate expiration time → failing to reach plateau = incomplete alveolar expiration = falsely low ETCO2
Artificially high PaCO2
- Should be measured from arterial sample
- Venous sampling will artificially ↑pCO2
- Loss of calibration of machine
Gladwin / Sakurai 2016
Examiner Comments
2018B 03: 29% of candidates passed this question.
The answer required an explanation of the causes of the difference between the PaCO2 and ETCO2. This required recognising how the end point of phase 3 of the capnograph trace corresponds with end tidal CO2. The difference is caused by the alveolar dead space. The difference is normally very small in healthy adults with the ETCO2 being lower than the PaCO2. It is increased with increasing alveolar dead space. Many incorrectly attributed anatomical dead space as a contributor to the PaCO2-ETCO2 gradient. Discussion of the various types of dead space did not score marks. Marks were awarded for the processes that cause an increased gradient e.g. low cardiac output and pulmonary embolism. Recognising physiological factors such as increasing gradient with increasing age scored marks. Marks were not awarded for descriptions on how dead space is measured.
4. Compare and contrast ketamine and midazolam.
Examiner Comments
2018B 04: 62% of candidates passed this question.
In addition to the key PK and PD properties of each drug, a clear comparison was required to score well (why choose one drug over the other?). When a table was used the addition of a comparison column was helpful.
A good answer covered the following: ketamine has analgesic properties whilst midazolam does not; ketamine preserves airway reflexes and does not cause respiratory depression unlike midazolam; whilst ketamine increases cerebral blood flow and CMRO2, midazolam decreases it; ketamine has a direct myocardial depressant effect which is often offset by an increase in sympathetic tone, whilst midazolam has no direct cardiac depressant effects but may reduce BP due to reduced SVR; midazolam has anticonvulsant properties, ketamine does not; ketamine is a bronchodilator; both drug effects are offset by redistribution; midazolam is lipophillic at body pH and will accumulate with prolonged infusions, ketamine will not; both are metabolised in the liver; midazolam can be reliably reversed by flumazenil, whereas there is no reliable complete reversal of ketamine; midazolam exhibits tolerance, dependence and withdrawal, whereas patients will only experience tolerance to the analgesic properties of ketamine.
“Drugs in Anaesthesia and Intensive care” chapters on midazolam and ketamine outline the key facts to include in this answer; interpretation and comparison of these facts will help achieve a good mark.\
5. Describe the carriage of carbon dioxide (CO2) in the blood.
CICMWrecks Answer
CO2
- End-product of aerobic metabolism
- Produced almost entirely in mitochondria
- Normal production approx. 200ml/min
- Total CO2 in blood
- Venous 52mL/dL
- Arterial 48mL/dL
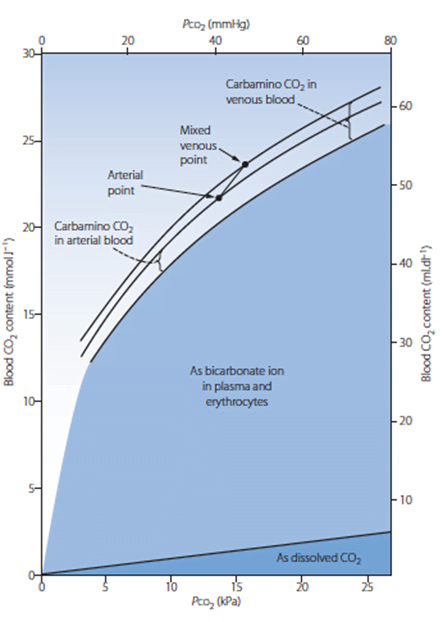
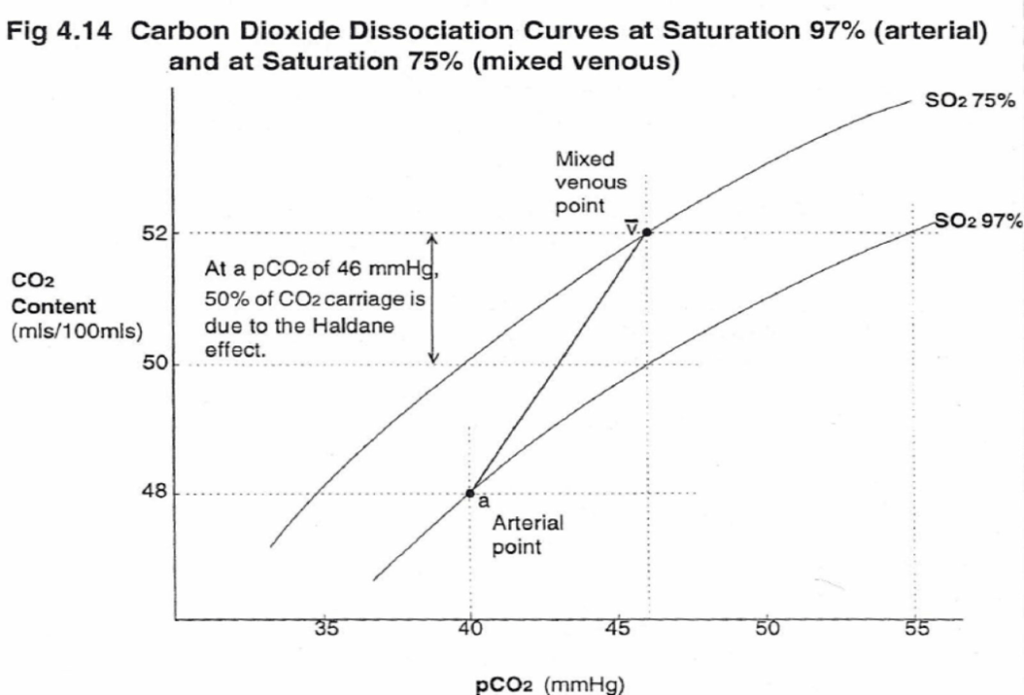
CO2 Carriage in 4 forms:
Arterial % | % of A-V diff | Details | |
---|---|---|---|
1. Bicarbonate | 90 | 60 | Main form of CO2 delivery in blood Via Hendersen-hasselbach: CO2 + H2O ⇔ H2CO3 ⇔ H+ + HCO3– Process 1. CO2 diffuses into RBCs 2. Carbonic anhydrase converts it to Bicarb 3. Hydrogen ions are buffered by binding to Hb molecules inside RBCs (30% Haldane.) Higher percentage in venous blood as: • Deoxygenated pKa 8.2 • Oxygenated pKa 6.6 • decreased pH = greater increase in [A-]/[HA] of DeoxyHb rel to OxyHb thus greater ability to accept protons 4. Bicarb is transferred out of cell in exchange for Cl ion by an exchange transporter called Band 3. (Hamburger Shift) |
2. Carbamino compounds | 5 | 30 | CO2 able to bind amino end of proteins Hb largely responsible as in much greater concentrations than any other protein (15 g/dL vs 7 g/dL) in serum CO2 combines with terminal amine to form carbamic acid dissosciates → at physiological pH to carbamate Affinity of Hb for CO2 increases in venous blood via Conformational change (70% Haldane): • Unbinding of O2 causes a conformational change in the beta chain • CO2 binding site at the N-terminus moves 1nm • Affinity for CO2 is increased. • Deoxy-Hb is 2-3x better at forming carbamino compounds than oxy-Hb |
3. Dissolved | 5 | 10 | CO2 ~20 x more soluble than O2 thus via Henry’s Law more CO2 is dissolved PvCO2 ~46mmHg (PaCO2 40mmHg) |
4. Carbonic Acid | <1% | <1% | Carried as dissolved H2CO3 Conversion to CO2 via Hendersen-Hasselbach equation above has pKa 6.1 At physiological pH Carbonic acid is ~96% dissociated Reaction is catalysed by Carbonic Anhydrase Not present in plasma, present in high concentrations in erythrocytes and pulmonary capilaries |
Gladwin / Sakurai 2016
Examiner Comments
2018B 05: 65% of candidates passed this question.
A definition of arterial and venous CO2 content (mls and partial pressure) and an outline of the 3 forms of CO2 in the blood and their contribution to the AV difference, followed by a detailed explanation of each form of carriage was required for this question. A good answer included a table of the contribution of each form of carriage to arterial and venous content and the AV difference; explained the concepts of chloride shift when describing carriage as HCO3-; detailed the Haldane effect and its contribution to carbamino carriage and referenced Henry’s law when describing dissolved CO2.
West’s Chapter 6 on gas transport details the key information to score well on this question.
6. Outline the determinants of venous return to the heart.
CICMWrecks Answer
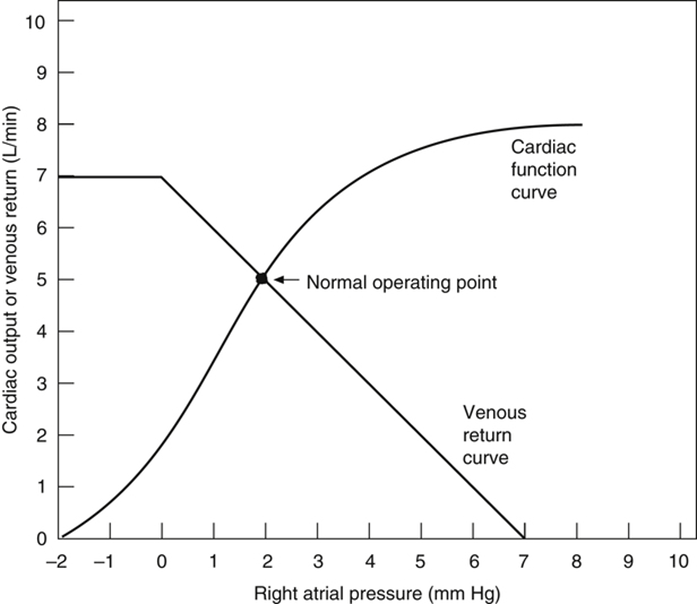
Vascular function (venous return) curve shows the effect of increases in venous return or CO (independent variable) on RA
pressure (dependent variable)
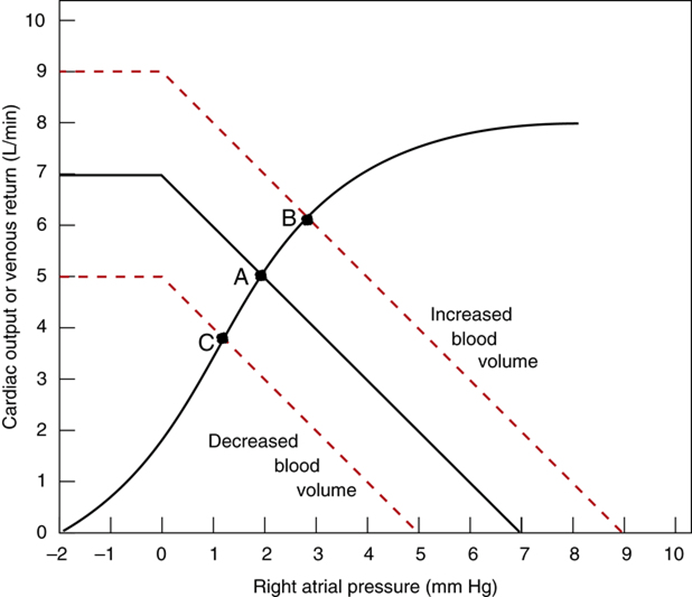
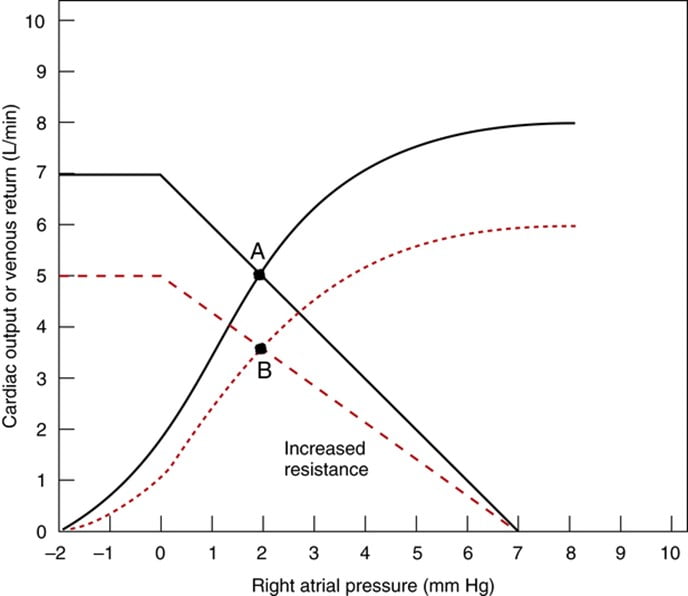
Factors that determine venous return back to the heart
The factors that influence VR are captured in 2 formulae:
The three variables that influence venous return are:
- Mean Systemic Filling pressure (MSFP)
- MSFP is the theoretical pressure present in the systemic circulation at equilibrium
- MFSP is the main driving pressure moving blood back towards the RA – It is the MAIN factor determining venous return (and thus CO)
- MSFP can be used to assess the degree of filling of systemic circulation: It is normally 7 mmHg (0-20 mmHg)
- Two factors influence MSFP:
- Blood volume ↑ → ↑ MSFP
- Venomotor tone (venous capacitance) ↑ → ↑ MSFP
- RA pressure (RAP)
- Resistance to venous return (RVR)
Therefore, the factors that affect venous return influence one or more of the aforementioned variables:
- Blood volume
- Blood volume is proportional to MSFP and thus venous return
- Venomotor tone
- ↑ venomotor tone (Eg. due to SNS activity) ↓ vein compliance and capacity → ↑ MSFP → ↑ venous return
- ↓ venomotor tone (Eg. due to SAB) ↑ vein compliance and capacity → ↓ MSFP → ↓ venous return
- This has a greater effect on venous return when
- (i) venous pressures are normal, and
- (ii) veins are circular (not collapsed and contain large volumes of blood)
- Venous valves
- Veins have one-way valves that prevent retrograde flow
- Skeletal muscle pump
- Alternating contraction and relaxation of limb skeletal muscle forces blood out of the veins towards the heart (thus, increasing MSFP)
- During contraction, veins compress to expel blood towards the heart, then during relaxation, veins distend and fill with blood
- With exercise, this pump function enhances net venous return
- Respiratory pump
- During inspiration, venous return is ↑ due to
- (i) ↓ RAP (associated with fall in PINTRAPLEURAL), and
- (ii) ↑ IAP (due to diaphragmatic contraction)
- During expiration, the effects are reversed
- Note that if RAP is < 0 mmHg, the respiratory pump will NOT have any effect on venous return as the thoracic veins would have collapsed at subatmospheric pressures
- During inspiration, venous return is ↑ due to
- Posture
- When going from supine to erect, there is ↓ venous return due to venous pooling in the lower extremities
- Normally, there is reflex vasoconstriction to prevent this BUT this reflex is delayed and less effective in the elderly
- Effect of ventricular contraction and relaxation
- During rapid ejection phase of ventricular systole, atrial pressure falls sharply (to zero or –ve values) as the ventricle contraction pulls the atrioventricular fibrous ring downwards and increases the atrial volume – This causes net blood flow into atria and increases venous return
- During early diastole, ventricles fill rapidly causing both ventricular and atrial pressures to decline – This facilitate blood flow into atria and increases venous return
- Intrapericardial pressure
- ↑ intrapericardial pressure (Eg. tamponade) can ↑ RAP, thus impeding venous return
- Afterload
- Changes in the dimension of the resistance vessels (arterioles) has a small effect on MSFP as only 2% of blood volume is in arterioles (cf. venous capacitance vessels) – Instead, it has an impact on “Resistance to venous return”
- ↓ afterload (such as decreased SVR due to arteriolar vasodilation) → ↓ RVR → ↑ VR
- ↑ afterload (such as increased SVR due to arteriolar vasoconstriction) ↑ RVR → ↓ VR
Bianca 2020
Examiner Comments
2018B 06: 31% of candidates passed this question.
Answers should have included a description of the need for a pressure gradient for flow and a discussion on right atrial pressure, mean systemic filling pressure and resistance to blood flow. The discussion of each of these factors included definitions, normal values, factors affecting them and the direction of change on venous return. Diagrams were not essential, but their use assisted some candidates in explaining the effects of RAP on venous return
7. Describe protein binding and its significance in pharmacology.
CICMWrecks Answer
Proteins and drugs may be bound together by weak bonds. These include ionic bonds, van der Waal’s forces, and hydrogen bonds.
Protein Binding:
- Refers to the degree to which drugs attach to proteins in the body.
- most drugs which are protein bound, bind either albumin or a1-acid glycoprotein according to their pKa
- some bind specialized proteins e.g. steroid binding globulin, transcortin etc
- increases Vd and reduces free fraction of drug
- reduces renal clearance by filtration but not active secretion
- is a source of interactions
- E.g: Phenytoin 95%, Warfarin 97%
Drugs may bind to proteins in:
- Plasma
- Albumin: Binds acid and neutral drugs.
- High capacity
- Three major binding sites (six total)
- Site I: warfarin, bilirubin, salicylates, phenytoin, sulfonamides
- Site II: benzodiazepine, NSAIDs, penicillin
- digoxin, verapamil, quinidine
- level is reduced by
- catabolic states: burns, malignancy, renal/hepatic disease, pregnancy, old age, neonates
- α1-acid glycoprotein: Binds basic drugs (e.g. lidocaine, propranolol, TCA’s)
- Single binding site
- Low capacity
Typically results in lower total binding (compared to albumin) of alkaline drugs, despite its increased affinity. - level increased in catabolic states: burns, renal transplant, malignancy, trauma, inflammatory diseases: RA, UC, Crohn’s, myocardial infarct
- level decreased in pregnancy, neonates
- Lipoprotein E.g. Steroid binding globulin, transcortin
For lipid soluble drugs. - Alpha, beta and gamma globulins
- Albumin: Binds acid and neutral drugs.
- Tissue
- Receptor
Protein binding is important as:
- Only unbound drugs are able to:
- Cross cell membranes
- Interact with receptors
- Undergo metabolism
- Reduced protein binding increases clearance of drugs with low extraction ratios.
- Be filtered by the kidney
- Highly tissue bound drugs:
- Have a long duration of action
- Have a high volume of distribution, prolonging their elimination
- May build up in tissues, leading to adverse effects
e.g. Corneal deposition, lung fibrosis.
Protein binding is affected by:
- Affinity of drug for protein
- Ionised drugs do not bind to protein
pH. - Competition between drugs for binding sites
- Ionised drugs do not bind to protein
- Amount of protein
- Disease: Due to:
- Hypoalbuminaemia: Negative acute phase reactant.
- Increased α1-acid glycoprotein: Acute phase reactant.
- Competition: Source of pharmacokinetic interactions.
- Disease: Due to:
Protein binding typically:
- Correlates with lipid solubility
- Is important only when it is very high
- Results in a decreased VDss when plasma binding is high
- Results in an increased VDss when tissue binding is high
- Is important in duration of action as it also relates to affinity for tissue proteins
JC 2019
Examiner Comments
2018B 07: 19% of candidates passed this question.
Descriptions of protein binding were generally too brief (e.g. a statement saying that drugs and hormones bind to proteins in the plasma rather than a description of usually reversible binding with a drug-protein equilibrium).
It was expected that the factors which determine protein binding would be described. Marks were attributed if proteins, along with characteristics of the drugs they bind, were named. Candidates achieved better marks if they named the pharmacological parameters affected by protein binding and explained how and why change occurs along with the significance of those changes. Few candidates differentiated between tissue and plasma protein binding and the different effects on the volume of distribution
8. Describe gastric emptying (40% of marks) and outline its regulation (60% of marks).
CICMWrecks Answer
Physiology of Gastric Emptying
Gastric emptying
- Fluids have half-time of 30mins in stomach
- Solids have half-time of 2 hours in stomach
Gastric receipt of food bolus:
- Peristaltic wave moves down the oesophagus, propelling food bolus
- Controlled by mesenteric plexus with input from vagus nerve
- Upon swallowing, the lower oesophageal sphincter and stomach relax until the oesophageal peristaltic wave has passed
- Following food bolus passage, the LOS tone increases to prevent reflux
- Food bolus reaching the stomach causes a vagal-mediated relaxation of the stomach, to accommodate gastric distension and food storage
Gastric mixing and emptying:
- Spontaneous mixing waves move down the stomach every 15-20 seconds
- Stronger waves propel gastric contents towards the pylorus (pyloric pump)
- High pyloric sphincter tone
- Allows through well-mixed liquid chyme
- Restricting gastric emptying of solids, causing mixing (retropulsion)
Control
Gastric emptying is controlled by the balance between stimulatory gastric factors and inhibitory duodenal factors
Factors promoting gastric emptying:
- Increased stomach wall stretch
- Stimulates pyloric pump
- Reduces pyloric tone
- Parasympathetic vagal stimulation
- Stimulates pyloric pump
- Gastrin
- Stimulates pyloric pump
- From G cells in antrum
- In response to presence of protein (esp. meat) in stomach
- Motilin
- Stimulates pyloric pump
- During fasting
- Stimulates pyloric pump
- From M cells in duodenum (external antigen stimulus)
- Carbohydrate: rapid emptying
Factors inhibiting gastric emptying:
- Nervous reflexes via enteric nervous system, local sympathetic trunk, and the vagal nerve to the brainstem
- in response to local conditions in the duodenum: Distension, Irritation, Acidity of chyme, Hyper- or hypo- tonicity of chyme, Breakdown products, Especially AA and FA
- Inhibit pyloric pump
- Increase pyloric sphincter tone
- Hormones (inhibit pyloric pump)
- Cholecystokinin (CCK)
- From I cells of duodenum
- In response to presence of fat and proteins
- Secretin
- From S cells of duodenum
- In response to presence of acids
- Gastric inhibitory peptide (GIP)
- From K cells of duodenum
- In response to presence of fats, proteins and carbohydrate
- Cholecystokinin (CCK)
- Other Factors :
- Sympathetic stimulus:
- Decreases contractility and reduces gastric emptying
- Dopamine:
- Decreases intragastric pressure and lower oesophageal sphincter tone
- reduces gastric emptying
- Sympathetic stimulus:
Alternate: This section can also be approached as:
- Local factors
- Gastric Factors: pump, Stomach wall distension, Amino acid
- Duodenal Factors: Type of food, stretch of wall, irritation, hyperosmolaroty, acidity, amino acid and FA content
- Neural factors
- Symptathetic
- Parasympathetic
- Hormonal factors
- Dopamine
- Gastrin
- Motilin
- Secretin
- CCK
- Somatostatin
- GIP
Mooney / Sakurai / JC 2019
Examiner Comments
2018B 08: 24% of candidates passed this question.
Candidates were required to provide a description of gastric emptying (40% marks). Although the question showed the allocation of marks, many candidates did not provide sufficient detail for this section. This required some description of what gastric emptying is (the co-ordinated emptying of chyme from the stomach into the duodenum).
Better answers provided detail regarding the process of gastric emptying in the fed and fasted state and differentiated between liquids, solids, carbohydrate, protein and fats. Factors regulating emptying included an outline of peristaltic waves, the basal electrical rhythm and its modulation, the migratory motor complex (MMC) and its modulation, neural input, stretch and hormonal control.
Many candidates erred by answering the question “the regulation of gastric secretions” rather than the question (the regulation of gastric emptying). Although they scored well for hormonal control, they missed out on marks for the other factors relevant to the regulation of gastric emptying.
9. Describe the renal handling of water including the modulation of water excretion.
CICMWrecks Answer
- Total body water = 60% body weight (approx. 42L in 70kg male)
- Intracellular fluid = 2/3 Total body water (approx. 28L)
- Extracellular fluid = 1/3 Total body water (approx. 14L)
- Plasma compartment = 1/4 Extracellular fluid (approx. 3L)
- Renal blood flow
- 1.25l/min
- Filtration fraction
- 0.2
- Glomerular filtration rate
- 180l/day
- >4x total body water
- Therefore renal water reabsorption important in H2O homeostasis
Water reabsorption
Mechanisms:
- difference in osmolality lumen : interstitial fluid. This created by reabsorption solutes (Na, Cl, glucose, AA)
- solute reabsorb actively ⇒ ↓luminal osmolality & ↑interstitial osmolality ⇒ gradient to drive water reabsorb across cells +/- tight junctions
Locations of Water Reabsorption:
PCT
- Highly permeable to water
- 65% H2O reabsorption → follows Na+ reabsorption via osmosis
LoH
- Thin descending limb
- Highly permeable to water
- 20% H2O reabsorbed
- Follows ions via osmosis
- Thick ascending limb
- Impermeable to water
- Countercurrent mechanism to increase papillary osmolarity to ~1400mosm/l
- Important for further H2O reabsorption
DCT/CD
- Variable permeability
- Controlled by aldosterone and vasopressin
If decreased total body water
- Decreased renal blood flow (beyond autoregulatory capabilities)
- Decreased glomerular filtration
- Decreased Na reaching DCT macula dense
- Release of Renin from Juxtaglomerular apparatus → Angiotensin II → Aldosterone
Angiotensin II
- Direct upregulation of proximal tubular Na reabsorption
- Efferent arteriolar vasoconstriction (as well as weak afferent arteriolar constriction) → increased filtration
- Increased thirst sensation
- Stimulates aldosterone release
- Stimulates vasopressin release
- Potentiates post-ganglionic sympathetic stimulation
Aldosterone
- Upregulates CD Na/K ATPase and inserts ENaC channels to increase Na reabsorption
- H2O follows Na via osmosis
Vasopressin (ADH)
- Stimulated by Angiotensin II, Osmoreceptors and low-volume baroreceptors
- Causes insertion of aquaporin 2 via V2 receptor stimulation
- Increased permeability of CD to H2O
- H2O reabsorbed due to high interstitial osmolarity created by counter-current mechanism and reabsorption of urea
If increased total body water
- Atrial stretch → production of natriuretic peptides (ANP, BNP)
- Leads to decreased reabsorption of Na in PCT
- Leads to decreased reabsorption of H2O
Sakurai 2016
Examiner Comments
2018B 09: 37% of candidates passed this question.
This question required a brief introduction of the role the kidney plays in water balance; a more detailed description of how water is handled as it passes through the various segments of the nephron (glomerulus, PCT, Loop of Henle, DCT and Collecting Duct); the modulation of water excretion by the kidney due to ADH (vasopressin) and how this operates; and the stimuli (osmotic and non-osmotic) for ADH secretion. Although worth mentioning in the context of the effect they have on water movement through the kidney, detailed explanations of Starling’s forces in the glomerulus, and of the operation and maintenance of the counter-current mechanism, were not required. More important was describing the control of water reabsorption in the collecting ducts (and thus modulation of water excretion by the kidney) under the influence of ADH.
10. Compare and contrast the pharmacology of vancomycin and flucloxacillin.
Examiner Comments
2018B 10: 49% of candidates passed this question.
Most candidates structured their answers well. Expected information included: the class of antibiotic of each agent, their respective pharmaceutics, pharmacodynamics, pharmacokinetics, indications and adverse effects. Better answers provided pharmacodynamic and pharmacokinetic information relevant to each drug rather than generic statements. Good answers also included the common resistance mechanisms for both agents.
11. Describe the anatomy relevant to the insertion of an intercostal catheter.
CICMWrecks Answer: Anatomy relevant to ICC Insertion
- Triangle of safety
- Lateral border of pectoralis major
- Anterior border of latissimus dorsi
- Superior to the 5th intercostal space – be aware the diaphragm (and liver/spleen) may be unexpectedly superior
- Layers (Superficial → Deep)
- Skin
- Subcutaneous connective tissue
- Fat
- Ribs and intercostal muscles
- Neurovascular bundle (intercostal artery, vein and nerves) lie in intercostal groove on infero-interior surface of the ribs
- External intercostal muscle
- Internal intercostal muscle
- Innermost intercostal muscle
- Parietal pleura
- Potential pleural space (pneumothorax)
- Visceral pleura
- Lung
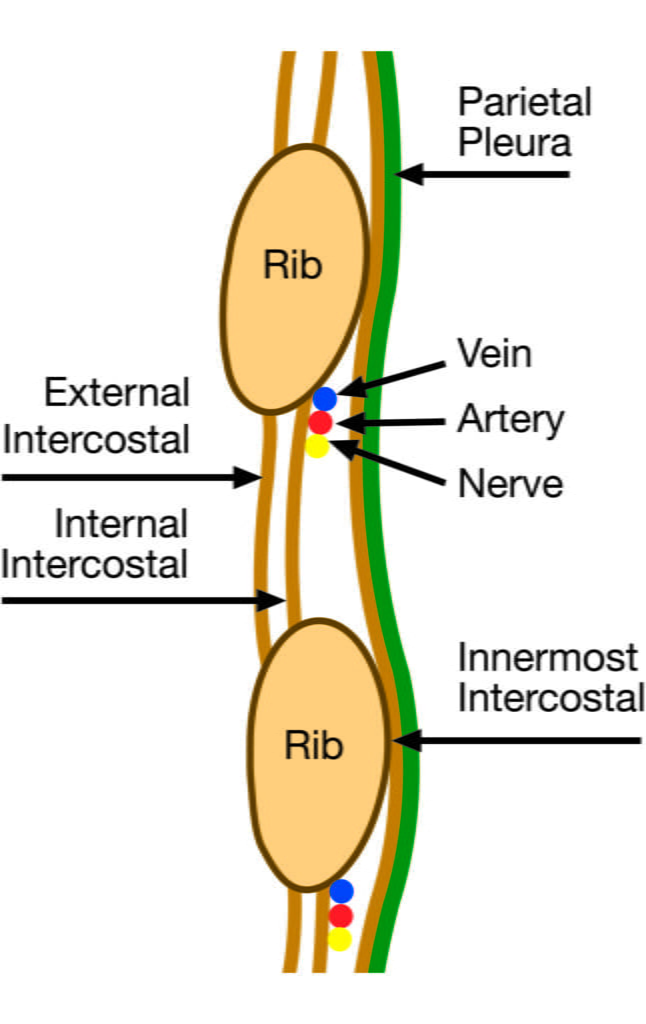
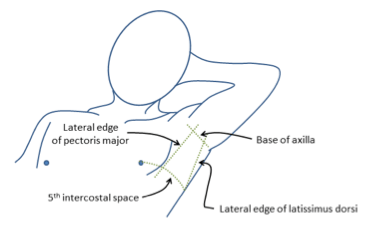
Sakurai / Mooney 2016
Examiner Comments
2018B 11: 56% of candidates passed this question.
An anatomy question expects the use of anatomical nomenclature to describe relationships.
Good answers defined the “safe triangle” for the lateral approach, soft-tissue layers passed through from skin to pleura and relationship of the neurovascular bundle to the ribs and intercostal muscles. Additional marks were gained for describing the anterior approach and related structures. Common omissions included description of deeper structures (relations) including intrathoracic and intra-abdominal organs and level of the diaphragm with regard to rib space.
No marks were awarded for a description of intercostal catheter insertion
12. Outline the control of blood glucose.
CICMWrecks Answer
General
- Normal BSL 4-6mmol/L
- Tight BSL control important as:
- ↓ BSL: disrupt normal function of brain, retina, gonads (obligate glucose users)
- ↑ BSL: ↑ osmolality, osmotic load on kidneys → diuresis + cellular dehydration, loss of electolytes/ substrate → tissue damage
BSL control via feedback mechanism
- Sensors: pancreatic islets of Langerhans
- Central regulator: lateral (feeding) and ventromedial (satiety) centres of hypothalamus
- Effectors:
- Behavioural (feeding)
- Hormonal: insulin vs. glucoagon balance (act on liver, muscle, adipocytes)
- Renal (excretion)
- Modulated by: catecholamines, cortisol, thyroid homrones
- short term regulation: via secretion or inhibition of insulin + glucagon from pancreatic islets
- long term: neuronal mechanisms (SNS activation) + hormones (cortisol, GH)
Sensors
- Pancreatic beta cells → sense ↑ BSL
- Secrete insulin in biphasic pattern: initial rapid ↑ → prolonged slow ↑
- 1st phase of insulin secretion: ↑ BSL → glucose enters via GLUT2 → converted to pyruvate → enters TCA → generate ATP → inhibit ATP sensitive K channel → ↓ K efflux → depolarisation → open voltage gated Ca2+ channels → exocytosis of insulin granules
- 2nd phase of insulin secretion: glutamate produced as by-product of TCA → maturation of other insulin granules
- Pancreatic alpha cells → sense ↓ BSL
- ↑ glucagon release
Effectors
- insulin: secreted in response to ↑ BSL → following effects to ↓ BSL
- ↑ GLUT4 insertion into cell membrane → ↑ glucose uptake into cells esp. muscle + fat
- ↑ glycogen synthesis
- ↑ glucose utilisation + ↑ fat and protein synthesis
- ↓ glycogenolysis / ↓ gluconeogenesis
- glucagon: secreted in reponse to ↓BSL → following effects to ↑ BSL
- ↑ glycogenolysis / ↑ gluconeogenesis
- minimal effect on adipose tissue and muscle
- Adrenaline: stimulated by ↓ BSL, stress
- Inhibit insulin
- Liver: ↓ glycogenesis, ↑ glucose release, ↑ KB
- Fat: ↑ FFA release, ↓ glucose uptake
- Muscle: ↓ glucose uptake, ↑ FFA metabolism
- Sustained ↓ BSL stimulates GH + cortisol release
- ↓ glucose utilisation + ↑ fat utilisation → limiting further ↓ BSL
- ↓ protein synthesis / ↑ aa release / ↑ FFA metabolism
- Neuronal Mechanisms:
- Hypothalamus directly stimulated by hypoglycaemia → ↑ SYNS activity → adrenaline release → stimulates hepatic glucose release
Kerr 2016
Examiner Comments
2018B 12: 53% of candidates passed this question.
A definition of normal glucose levels was expected, mentioning how it is regulated despite variable intake. Most answers incorporated the roles of insulin/glucagon and the glucostat function of the liver. Sufficient detail regarding the mechanism of insulin release was often lacking. Extra marks were awarded for description of the role of the satiety centre in the hypothalamus, glucokinase and processes in fasting and starvation that maintain blood glucose levels.
Marks were not awarded for describing effects of insulin and glucagon unrelated to glucose control.
13. Compare and contrast rocuronium and cisatracurium.
Examiner Comments
2018B 13: 32% of candidates passed this question.
This question was best answered using a tabular format outlining class of drug, pharmaceutics, pharmacokinetics, reversibility and side effects. Better answers commented on the significance of the differences between the two agents and its relevance to ICU practice. Many candidates confused these muscle relaxants with each other and with depolarising muscle relaxants.
14. Explain the detection and response to hypoxaemia.
CICMWrecks Answer
Hypoxaemia
Hypoxaemia = Acute ↓ PaO₂ = (low partial pressure of oxygen in arterial blood)
Overall response: Hypoxaemia → ↑ peripheral vasosmotor tone, ↑rate and depth of respiration
Local response to ↓ PaO₂:
- ↑ local vasodilation → ↓ SVR and ↓ BP
- ↓ BP → Baroreceptors → SNS activation → ↑ HR and ↑ C.O.
- PaO2 < 50-60 mmHg
- → peripheral chemoreceptors stimulation (see below)
- → ↑ventilation
- If ↓ PaO₂ is Gradual ↑ MV PaO2 < 500mmHg,
- If ↓ PaO₂ is Rapid ↑MV PaO2 < 50 mmHg
- Severe hypoxaemia
- ↓ HR and BP → shock
- ↑ CBF (regardless of PaCO2)
Chemoreceptor (CR) Reflex:
Two types of CR:
- Central CR
- CR location: Below the ventral surface of the medulla
- Stimuli: Δ ECF [H+] whereby ↑ ECF [H+] stimulates ↑ ventilation
- Rapid Response:
- CO2 rapidly diffuses into CSF
- Low CSF protein and HCO3 (c.f. plasma)
- Poor buffering capacity
- ΔCSF pH per ΔPaCO2 is GREATER than that of blood
- Peripheral CR
- CR location:
- “Carotid bodies” (more important)
- at bifurcation point of common carotid arteries
- supplied by CN IX
- “Aortic bodies” (less important)
- above and below the aortic arch
- supplied by CN X
- “Carotid bodies” (more important)
- Composition:
- PCRs found in “Glomus cells”
- ↓ PaO2 – via inhibition of O2 sensitive K-channels
- ↑ PaCO2 and/or ↓pH – via effect on pH sensitive K-channels
- Type I cells (rich in NAd, DA, ACh)
- Hypoxia causes release of NTs
- NAd/ACh – ↑ AP firing rate of AB or CB afferent fibres
- DA – Damping of type 2 cell responses
- Hypoxia causes release of NTs
- Type II cells (rich in capillary supply)
- ↓ PaO2, ↑ PaCO2 and/or ↓pH
- ↓ IC [ATP] which leads to ↑ NT production and release
- ↑ AP firing rate of AB or CB afferent fibres
- PCRs found in “Glomus cells”
- Response:
- ↓’d perfusion → ↑[H]/↓PaO2 → CR stimulation
- Afferent signal → Sinus nerve (of herring) / Vagus Nerve → Chemosensitive area of medulla
- Vasomotor area → ↑ peripheral vasoconstriction
- Respiratroy center → ↑rate and depth of respiration → ↑ venous return
- CR location:
Chronic Response
- Adrenal medullary hypoxia → EPO
- ↑ erythropoiesis (chronic)
Gladwin 2016
Examiner Comments
2018B 14: 34% of candidates passed this question.
A logical approach to answering this question included a definition of hypoxaemia and then a discussion of the sensors, integrators and effectors involved. It was expected that candidates would cover the peripheral chemoreceptor response (including the respiratory, cardiovascular and autonomic effects), time course of the ventilatory response, hypoxia-inducible factors, vascular effects (hypoxic vasoconstriction in the pulmonary circulation and vasodilatation in the systemic circulation) and metabolic changes (switch to anaerobic metabolism). No marks were awarded for discussing the causes of hypoxaemia. Many candidates incorrectly stated that hypoxaemia is detected by the central chemoreceptors.
15. Outline the production / absorption (30% of marks), composition (30% of marks) and function of cerebrospinal fluid (CSF) (40% of marks).
CICMWrecks Answer
Formation / Production of CSF
- 60-70% of the CSF is formed by the choroid plexuses
- 30-40% by the cerebral vessels lining the ventricular walls
- Normal rate is 20mL/hour
- Formation independent of ventricular pressure
- Mechanism
- From Coroid Plexus by net transport of Na+, K+, Cl-, HCO3- and water, from plasma to ventricles
- Na down Conc grad
- Others down electro chem grads
Distribution / Circulation of CSF
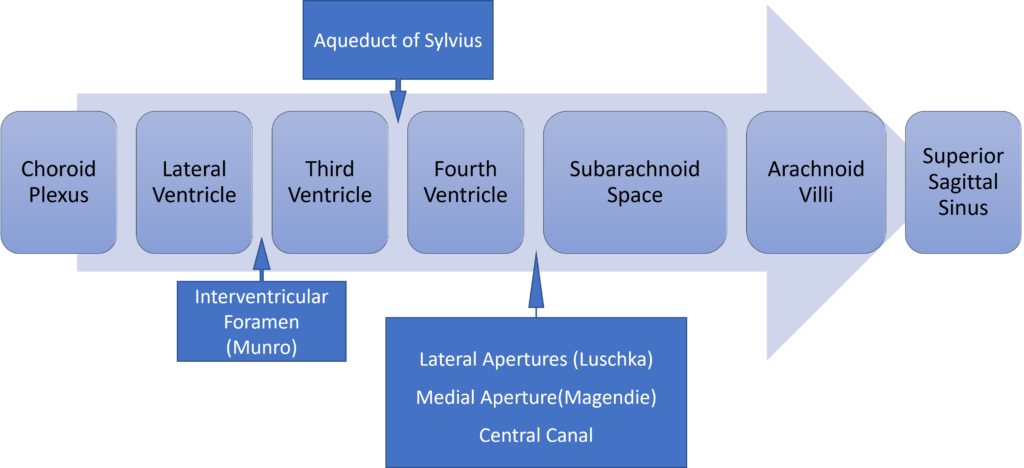
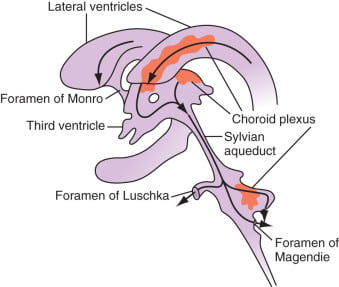
Absorption of CSF
- Absorbed through the arachnoid villi into the cerebral venous sinuses
- Absorption by bulk flow, is proportional to ventricular pressure
- If pressure < 7 cmH2O, CSF absorption ceases
- Above 7cmCSF absorption is linear
- At approximately 11 cmH2O, CSF Absorption = Formation
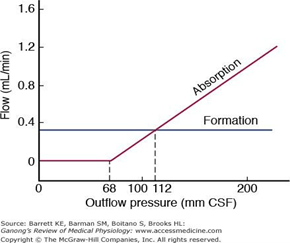
Composition of CSF
IDENTICAL to brain ECF, but differs in several manners from plasma
Compared with plasma:
- ↑ pCO2 (50 mmHg)
- ↓ pH (7.33)
- ↓ protein content
- 0.5% of plasma; 20 mg/dL
- poor acid-base buffering capacity
- ↓ content of glucose (by 60%) and cholesterol
- ↑ [Cl-] (by 7-14%) and ↑ [Mg2+] (by 40%)
- ↓ [K+] (by 40%), ↓ [Ca2+] (by 50%) and ↓ [Pi] (by 20-30%)
- ↑ creatinine (by 25%) but ↓ urea
- IDENTICAL osmolality (295), [Na+] (145), and [HCO3] (25)
Role / Functions of CSF
- Protective role (main function)
- Water bath effect
- Attributed to the low specific gravity of CSF (1.007)
- Causes brain to be buoyant
- ↓ its effective net weight from 1400 g to 50 g
- Mechanical cushion against acceleration/deceleration forces
- Buffer ↑ ICP by CSF translocation to extracranial subarachnoid space
- Abrupt ↑ ICP buffered by translocation of CSF within the vault to extracranial compartments
- Water bath effect
- Maintains constant ionic environment conducive to neuronal electrical activity CSF
- Supply role of nutrients (Eg simple sugars, amino acids) and O2 to brain
- Excretion of toxic substances, metabolic by-products, and CO2 from brain
- “Lymph-type” function → interstitial proteins in brain ECF return to circulation by
- CSF absorption across arachnoid villi
- Acid-base regulation → due to content, CSF allows for tight respiratory control
- Endocrine transport function → transports hormones to other brain regions
Gladwin / JC 2020
Examiner Comments
2018B 15: 71% of candidates passed this question.
This question was generally well answered. Better answers noted production including an amount, site and mechanism. Similarly, absorption included the site, the rate and factors which affect the rate. The electrolyte and pH and how they compare to extracellular fluid should have been included in the section on composition.
16. Describe the forces that result in fluid exchange across capillary membranes.
CICMWrecks Answer
Introduction
Capillaries contain semipermeable membranes to allow the movement of fluid and solutes.
- it is normally impermeable to large protein
- Plasma ultrafiltrate is filtered by bulk flow through the capillary wall by the action of opposing hydrostatic and oncotic pressures
Starling Forces
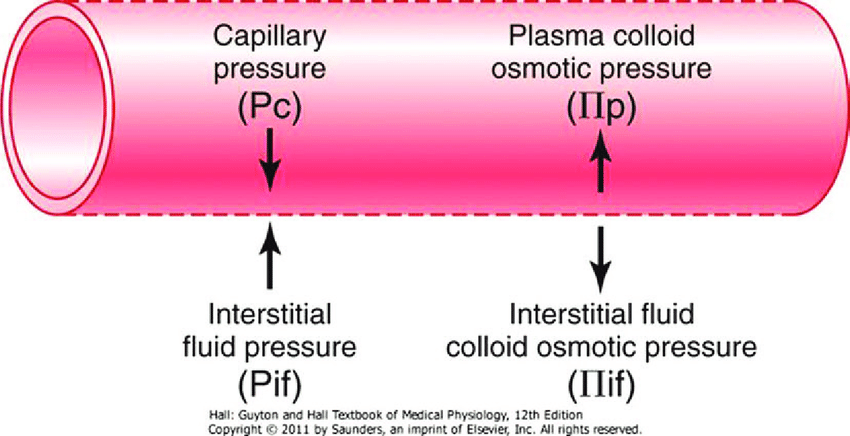
- The NET flux across the membrane is the balance of hydrostatic pressure and oncotic pressure, as defined by the Classic Starling Equation:
where
Jv is the trans endothelial solvent filtration volume per second
( [ Pc – Pi ] – σ [ πp – πi ] ) is the net driving force
P = hydrostatic pressure
π = oncotic pressure
σ = Staverman’s reflection coefficient ie. Permeability of membrane to protein
κ = filtration constant = LpS = Hydraulic conductivity x Surface Area
- Typically quoted values for the variables in the classic Starling equation:
Hydrostatic pressure | Oncotic pressure |
---|---|
Pressure moving fluid | pressure exerted by proteins which draw water into and keep it within a compartment |
Pc ~35 → 15mmHg (Arterial → venous) Capillary hydrostatic pressure Pressure moving fluid out of capillary | πp ~ 20mmHg Plasma oncotic pressure Pressure keeping fluid within capillary |
Pif = 5mmHg Interstitial hydrostatic pressure Pressure moving fluid into capillary | πif ~ 0mmHg Interstitial fluid oncotic pressure Pressure keeping fluid out of capillary |
- In general, at the arterial end of capillary NFP is positive (filtration) +10mmHg
- At the venous end NFP is negative (absorption) -10mmHg
- Approx. 24L fluid filtered / day
- 85% reabsorbed into capillaries
- Rest reabsorbed via lymphatics (~3.5L/day) = Net fluid loss from filtration
Role of plasma oncotic pressure
- largely fixed as capillary is impermeable to oncoproteins (1° albumin)
- If ↓πc, ↑net movement of fluid into interstitium
- Once this exceeds the drainage capacity of lymphatics → oedema occurs
- Especially dangerous in areas where minimal interstitial distance is required b/n capillary and cell to continue normal functioning (e.g. lung)
JC 2019
Examiner Comments
2018B 16: 57% of candidates passed this question.
The expected answer included a clear explanation of Starling’s forces, including an understanding of the importance of the relative difference along the length of the capillary, with approximate values and examples of factors that influence them. Some explanation of what contributed to the hydrostatic or osmotic pressure gained more marks than merely stating there was a pressure. Several candidates digressed to Fick’s law of diffusion or intracellular flow of ions which was not directly relevant to capillary flow.
17. Describe ketone bodies including their synthesis and metabolism.
CICMWrecks Answer
Ketone bodies
Introduction:
- Ketone bodies are three water-soluble molecules (acetoacetate, beta-hydroxybutyrate, and their spontaneous breakdown product, acetone) containing the ketone group
- provide an alternative source of substrate for energy production
- Ketones can only be produced by the liver, and only used as a substrate by the kidney, as well as skeletal and cardiac muscle
- Production of ketones is accelerated by glucagon and adrenaline
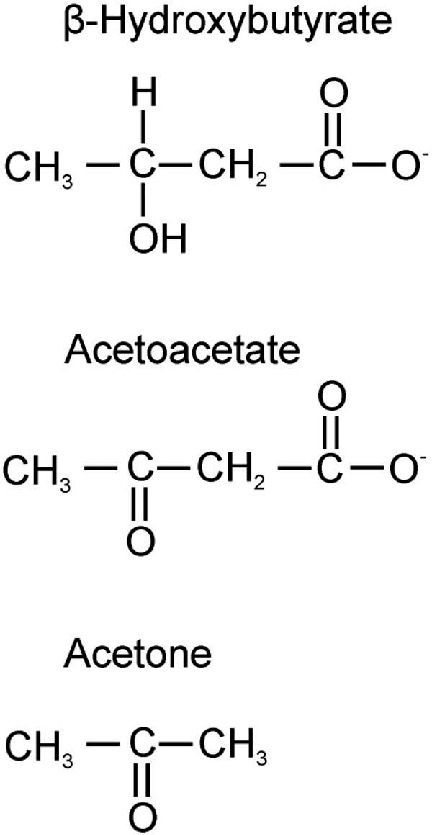
Ketogenesis:
- They are produced by the mitochondria in liver cells from fatty acids
- β-oxidation of fatty acids in the liver produces acetyl-CoA
- Acetyl-CoA usually enters the citric acid cycle to produce ATP
- When large amounts of acetyl CoA are produced, they may instead condense to form acetoacetate, which can then be reduced to β-hydroxybutyrate (These three molecules are called ketone bodies)
Ketone bodies
- Acetoacetate: When two acetyl-CoA molecules lose their -CoAs, (or Co-enzyme A groups) they can form a (covalent) dimer called acetoacetate.
- Beta-hydroxybutyrate: Beta-hydroxybutyrate is a reduced form of acetoacetate, in which the ketone group is converted into an alcohol (or hydroxyl) group. Both are 4-carbon molecules, that can readily be converted back into acetyl-CoA by most tissues of the body, with the notable exception of the liver.
- Acetone: Acetone is the decarboxylated form of acetoacetate which cannot be converted back into acetyl-CoA except via detoxification in the liver where it is converted into lactic acid, which can, in turn, be oxidized into pyruvic acid, and only then into acetyl-CoA.
Circumstances:
- Ketogenesis takes place in the setting of low glucose levels in the blood, after exhaustion of other cellular carbohydrate stores, such as glycogen.
- low food intake (fasting), carbohydrate restrictive diets, starvation, prolonged intense exercise, alcoholism
- State of Low insulin and high glucagon is what stimulates the liver
- It can also take place when there is insufficient insulin (e.g. in type 1 (but not 2) diabetes), particularly during periods of “ketogenic stress” such as intercurrent illness.
- untreated (or inadequately treated) type 1 diabetes mellitus.
Transport:
- Ketone bodies are readily transported into tissues outside the liver and converted into acetyl-CoA, which then enters the citric acid cycle and is oxidized in the mitochondria for energy.
- In the brain, ketone bodies are also used to make acetyl-CoA into long-chain fatty acids.
Metabolism
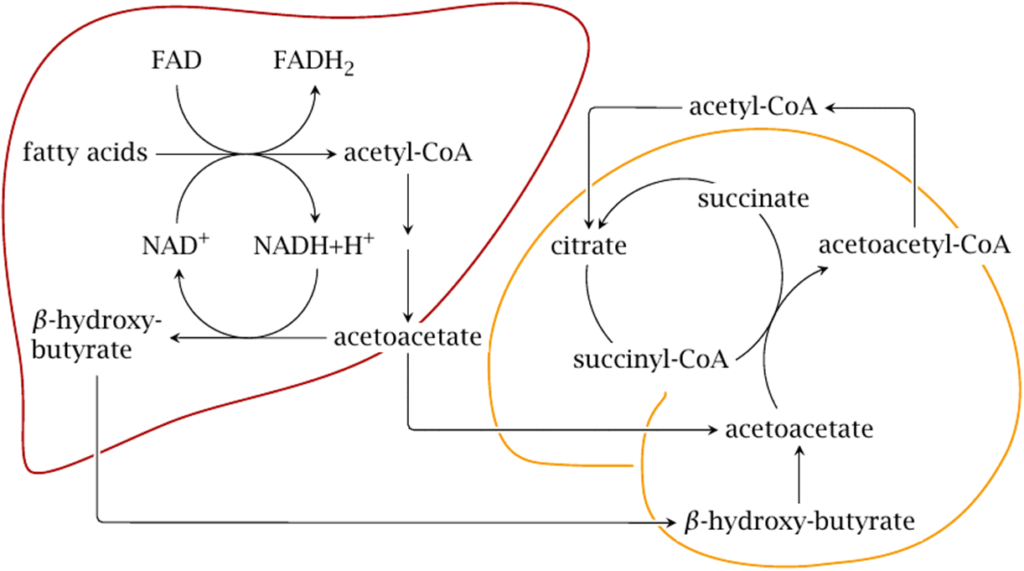
- Ketone bodies can be utilized as fuel in the heart, brain and muscle, but not the liver. They yield 2 GTP and 22 ATP molecules per acetoacetate molecule when oxidized in the mitochondria.
- Liver: cannot use them for energy because it lacks the enzyme thiophorase (β-ketoacyl-CoA transferase). Acetone is taken up by the liver in low concentrations and undergoes detoxification through the methylglyoxal pathway which ends with lactate. Acetone in high concentrations, as can occur with prolonged fasting or a ketogenic diet, is absorbed by cells outside the liver and metabolized through a different pathway via 1,2-propanediol. Though the pathway follows a different series of steps requiring ATP, 1,2-propanediol can eventually be turned into pyruvate
- Heart: Preferentially utilizes fatty acids as fuel under normal physiologic conditions. However, under ketotic conditions, the heart can effectively utilize ketone bodies for this purpose
- Brain:
- The brain gets a portion of its fuel requirements from ketone bodies when glucose is less available than normal (e.g., during fasting, strenuous exercise, low carbohydrate, ketogenic diet and in neonates).
- In the event of a low glucose concentration in the blood, the brain has an obligatory requirement for some glucose.
- After the diet has been changed to lower blood glucose utilization for 3 days, the brain gets 25% of its energy from ketone bodies.
- After about 24 days, ketone bodies become the major fuel of the brain, making up to two-thirds of brain fuel consumption.
Examiner Comments
2018B 17: 35% of candidates passed this question.
Whilst most candidates understood that ketones provided an alternative source of substrate for energy production, many lacked a basic understanding of their synthesis and metabolism.
Important facts included what ketone bodies are, where they were synthesised, where they were taken up and used as fuel, under what circumstances they are used and the integral role of insulin. Many candidates accurately reproduced the glycolytic and/or the TCA cycle, but this was not being examined, and did not score additional marks. Many candidates incorrectly stated that ketone production was the result of anaerobic metabolism.
18. Describe the factors affecting left ventricular function.
CICMWrecks Answer
LV Systolic Function
LV systolic function is a function of its contractility.
Contractility = the change in force generated independent of preload
Factors affecting Contractility
Contractility is primarily dependent on intracellular Ca2+. Determinants include:
- Metabolic:
- substrate supply – glucose, fat, protein
- metabolic/electrolyte homeostasis
- hormones – thyroid, insulin/glucagon
- Physiological:
- integrity of myofilaments
- co-ordinated depolarisation
- functional muscle mass
- autonomic tone – sympathetic and parasympathetic
- Drugs: Digoxin, b agonists, PDE3 inhibitors
- Minor increases in contractility occur as an intrinsic response to:
- increased afterload
- Anrep Effect: Autoregulation method in which myocardial contractility increases with afterload.
- increased heart rate
- Bowditch Effect: Autoregulation method by which myocardial tension increases with an increase in heart rate. Also known as the Treppe phenomenon, Treppe effect or staircase effect .
- (Contractility improves at faster heart rates. This is because the myocardium does not have time to remove calcium, so it accumulates intracellularly.)
- increased afterload
- Disease
- Ischaemia / Coronary Blood flow
Reduced ATP production secondary to hypoxia, which impairs sarcoplasmic reticulum Ca2+ function. Further exacerbated by intracellular acidosis from anaerobic metabolism. - Heart Failure
Impaired contractility reserve, i.e. minimal increase in contractility with sympathetic stimulation.- Reduced peak Ca2+and sarcoplasmic reticulum uptake of Ca2+
- Ischaemia / Coronary Blood flow
LV Diastolic Function
LV diastolic function is determined by its compliance. LV compliance is primarily determined by myocardial characteristics and load.
Factors affecting LV diastolic function:
- Normal HR and rhythm
- LV systolic function
- Wall thickness
- Chamber geometry
- Duration, rate and extent of myocyte relaxation
- LV untwisting and elastic recoil
- Magnitude of diastolic suction
- LA-LV pressure gradient
- Passive elastic properties of LV myocardium
- Viscoelastic effects (rapid LV filling and atrial systole)
- LA structure and function
- Mitral valve structure and function
- Pulmonary venous blood flow
- Pericardial restraint
- RV loading conditions and function
- Ventricular interdependence
- Coronary blood flow and vascular engorgement
- Compression by mediastinal masses
JC 2019
Examiner Comments
2018B 18: 12% of candidates passed this question.
Candidates often misinterpreted the question and described determinants of cardiac output. The answer should have focussed on factors affecting/contributing to normal LV function – not pathological states. Some answers showed a lack of appreciation that normal left ventricular function is afterload independent, due to compensatory reflexes. Answers needed to consider intrinsic and extrinsic factors affecting LV function – the latter (e.g. SNS, PSNS, hormones, drugs) was often left out. Answers needed to consider both systolic and diastolic function. An excellent answer included physiological phenomena such as the Treppe effect, Anrep effect and baroreceptor and chemoreceptor reflexes. Mention of normal conduction and pacing as well as blood supply limited by diastole scored additional marks.
19. Describe toxicity of local anaesthetic agents.
CICMWrecks Answer
Background
- toxicity = unwanted harmful effect of a drug
- LA= drugs that reversibly inhibit transmission of neural impulses in the applied region without affecting consciousness
- Toxicity from LA can be classified into local or systemic
- Local toxicity: mechanism of delivery + reaction to PABA
- Systemic toxicity = due to excess plasma concentration determined by rate of drug entrance relative to its redistribution and clearance by metabolism
Maximum recommended doses:
Dose in mg/kg | Toxic plasma levels | CC:CNS ratio | |
---|---|---|---|
Lignocaine | 3mg/kg without adrenaline 7mg/kg with adrenaline | 5microg/ml | 7 |
Bupivacaine | 2mg/kg up to 140mg +/- adrenaline | >1.5microg/ml | 3 |
Ropivacaine | 3mg/kg +/- adrenaline | >4microg/ml | 5 |
Toxicity Mechanisms and Effects
Local toxicity
- High epidural or spinal blockade
- β fibres = small myelinated fibres readily blocked by LAs
- large doses of epidural/spinal LA can result in inappropriately high block
- High SY chain block
- Vaso + veno dilation → ↓SVR + ↑venous capacitance → ↓↓MAP
- Venodilation → ↓VR → ↓RAP → ↓HR
- Blockade of cardioaccelerator (T1-T4) fibres → ↓↓HR
- o Brainstem block
- Blockade of resp centre → profound resp depression
- Blockade of autonomic centre → CVS collapse (↓↓MAP + ↓↓HR)
- Neurotoxicity
- epidural/ intrathecal injection → neurotoxicity: mechanism: lignocaine ↑intracellular [Ca2+] → neurotoxic via unknown mechanism
- radicular irritation → transient neurological symptoms that resolve over 1-7days
- cauda equina syndrome → diffuse lumbosacral plexus injury → sensory, bladder + bowel dysfunction, paraparesis
- anterior spinal artery syndrome → lower limb paresis + dysaesthesia ?2o
anterior spinal artery spasm
Systemic toxicity
- CNS toxicity
- Biphasic effect
- Excitatory phenomena: depression of inhibitory interneurons → circumoral tingling, tongue numbness → restlessness, tinnitus, vertigo → skeletal + facial muscle twitching → seizure
- CNS depression: depression of central neurons → coma, apnoea
- Seizure ?2o inhibition of inhibitory GABAergic pathways → unopposed excitatory activities → seizure
- Biphasic effect
- CVS toxicity
- ↑↑dose required to produce CVS toxicity than CNS toxicity
- Main effects: hypotension + bradyarrhythmias
- Mechanism:
- Cardiac Na channel blockade LAs → slow conduction of cardiac impulses → PR prolongation + QRS widening → VTs
- Inhibit Ca2+ and K+ ion channels
- Inhibit cAMP synthesis (minor)
- Relaxation of arteriolar vascular smooth muscle
- CC:CNS ratio: ratio of the dose required to cause CVS collapse and the dose required to cause CNS toxicity: indicates that CNS is ↑vulnerable to LA than CVS
- Hypersensitivity reaction
- Rare
- LA or additive can → hypersensitivity reaction
- Ester LAs metabolised to para-aminobenzoate (↑rates allergy)
- Hepatotoxicity
- Continuous epidural infusion of bupivacaine found to rarely cause hepatic enzyme derangement + hepatoxicity
- Mechanism unclear
- Methaemoglobinaemia
- Prilocaine metabolised in liver to o-toluidine → oxidises Fe2+ of haem to Fe3+ → ↑methaemoglobin (unable to carry O2) → tissue hypoxia
Factors affecting risk of toxicity
- Drug factors
- Dose
- Route + location of administration
- Potency; pKa
- Isomerism (R-enantiomer more toxic than S)
- Rate of metabolism
- PB (↑PB → ↓free drug → ↓toxicity)
- Intrinsic vasodilator activity
- Patient factors
- Acidosis → ↑ionised → ion trapping → ↑toxicity
- Pregnancy → ↑progesterone → competitive binding to AAG → ↑unbound LA → ↑toxicity
- Hepatic/renal dysfunction
- Heart failure → ↓perfusion + ↓VD → ↓elimination
- Plasma cholinesterase activity
Prevention of LA toxicity
- Frequent aspirations
- Slow injection
- Test dose
- Total dose administered < max recommended dose
- Awake patient
- Monitoring
- USS guided
- Vasoconstrictors
Kerr 2016
Examiner Comments
2018B 19: 28% of candidates passed this question.
Most questions lacked a systematic approach to the question and specific detail. The relationship between systemic toxicity (CNS and CVS) and plasma levels should be described.
Many candidates did not clearly state that CNS toxicity occurs at lower plasma levels that CVS toxicity. Factors that affect toxicity (e.g. drug factors, patient factors, interactions) needed to be elaborated with some detail. Patient factors such as age, pregnancy, acidosis, hyperkalaemia, hepatic failure were often omitted. Finally, marks were also awarded for noting methaemoglobinaemia as possible toxicity and the existence of specific therapy (intralipid).
20. Describe the pharmacology of heparin highlighting important differences between unfractionated and fractionated (low molecular weight) heparin.
Examiner Comments
2018B 20: 71% of candidates passed this question.
Better answers were tabulated and included sections on pharmaceutics, indications and an explanation on how the difference in molecular weight influenced pharmacodynamics and pharmacokinetics. Knowledge of adverse effects was limited to bleeding and HITTS, often without consideration of relative risk from LMWH. Many candidates did not know the t1/2 of UFH or LMWH.
VIVAs
A. Pharmaceutics | |
B. Pharmacokinetics | |
C. Pharmacodynamics | |
D. Variability in Drug Response | |
E. Cellular Physiology | |
F. Respiratory | Oxygen Cascade FRC Neonatal Physiology, Airway vs adult Spirometry |
G. CVS | Catecholamines, Adrenaline, structural components LV P-V loop Coronary circulation |
H. Renal | Diuretics – pharmacology, MoA Renal blood flow, JXG apparatus |
I. Body Fluids and Electrolytes | |
J. Acid Base | |
K. Neuro | Opioids, MoA CBF – flow, distribution |
L. Musculoskeletal | NM function, NM blockers, Peripheral nerve stimulator |
M. ANS | |
N. Liver | |
O. GIT | |
P. Nutrition and Metabolism | |
Q. Haematology | Thromboelastography, label the trace |
R. Thermoregulation | Heat and Temperature |
S. Immunology | |
T. Microbiology | Microbial pathogen classification |
U. Endocrine | |
V. Obstetrics | Maternal oxygenation, normal ABG values for 38 wk pregnant |
W. Measurement and Monitoring | |
X. Procedures |
Recent Comments