1. Describe the pharmacology of midazolam.
Examiner Comments
2022B 01: 88% of candidates passed this question.
Most candidates had a broad understanding of the indications, mechanism of action and the broad pharmacodynamic effects of midazolam. Those candidates who scored poorly demonstrated a lack of clarity and detail around the pharmacokinetics which are critical to its use and side effect profile. The examiners noted the details surrounding its bioavailability, protein binding was either almost universally absent or were generic statements that don’t score marks. Better candidates mentioned the changes in ring structure and lipophilicity associated with changes in pH and showed good understanding of clinically relevant facts regarding lipid solubility and active metabolites.
2. Compare and contrast fresh frozen plasma and prothrombin complex concentrate.
CICMWrecks Answer
Fresh Frozen Plasma (FFP) | Prothrombin Complex Concentrate (Prothrombinex) | |
Intro | Human plasma containing all coagulation factors including the labile plasma coagulation Factors VIII and V | Human plasma derivative containing concentrates of factors II, IX and X (500IU PCC has 500IU of II, IX, X each in a vial) The 3 factor prothrombin complex concentrate available in Australia (4 factor PCC in some European countries) |
Preparation | Prepared either via: Separation from whole blood Apheresis: Removal of a large volume (typically 800ml) of plasma from a single patient, with return of red cells to the donor. Once collected, it is frozen and rethawed in a water bath prior to use | developed through the process of ion-exchange chromatography from the cryoprecipitate supernatant of large plasma pools and after removal of antithrombin and factor XI |
Indications | reversal of warfarin anticoagulation (in setting of bleeding or need for invasive procedure) Bleeding and multiple coagulation defects (e.g. DIC with significant PT/PTT elevation) Correction of coagulation defects for which no specific factor is available Transfusion of more than one blood volume with evidence of active bleeding + coagulopathy accepted treatment for patients with thrombotic thrombocytopenic purpura often in conjunction with plasma exchange. Where PCCs are not available | Bleeding and warfarinized Bleeding from factor deficiency (either congenital or due to liver disease, and haemophilia) Prophylaxis |
Contraindications | Allergy to class/drug ABO incompatibility when you can correct coagulopathy effectively with specific therapy in plasma exchange procedures except for treatment in thrombotic thrombocytopenic purpura treatment of immunodeficiency states | Allergy to class/drug DIC known HIT (Heparin induced thrombocytopenia) patients with haemophilia B if a specific factor IX concentrate is available |
PC | Dose: 10-15ml/kg will increase factors by ~20-30% Transfuse at least 15 mL/kg at a time (4 units in 70-kg adult) INR >1.5 and needs invasive procedure INR >1.5 and actively bleeding (e.g. massive transfusion protocol, post-bypass surgery) INR of FFP is ~1.6; therefore transfusing for INR <1.7 is not advised | Dose: – 25-50 IU\kg (1 IU\kg of Factor IX raises the Factor IX by 1%) – Dose adjusted based on INR |
PD – MoA | restores factors II, VII, IX and X in the anticoagulated patient | provision of factors II, IX and X |
Side Effects | Serious Hemolytic transfusion reactions Febrile non-hemolytic reactions Transfusion-associated circulatory overload (TACO) Transfusion-related acute lung injury (TRALI) Transfusion-associated graft-versus-host disease Anaphylaxis Sepsis Common Headache, paraesthesia Nausea, Pruritus, urticaria | Serious Thromboembolic Events Common Allergy, anaphylaxis phlebitis vomiting, fever rash, urticaria SOB pain thrombocytopenia |
PK | IV Distributed and metabolized in the same way as endogenous coagulation factors | IV Distributed and metabolized in the same way as endogenous coagulation factors |
Advantages | cheap | small volume readily available reliable reversal avoids complications of FFP – immune reaction, TRALI, fever, infection risk familiarity cheap |
Disadvantages | ABO compatibilty a must (but crossmatch before transfusing not) Needs to be thawed – time delay Immune reaction, TRALI, fever, infection risk significant fluid load in patients that are elderly and frail, or have heart failure. | More expensive than FFP May cause thromboembolic complications |
Examiner Comments
2022B 02: 52% of candidates passed this question.
The safe and appropriate use of these products in ICU is a key area of critical care practice so a fair bit of detail was required for a pass. Most candidates had a decent understanding of the main constituents of the two products, although few mentioned that FFP may still contain some cells, or that prothrombin concentrates contain heparin. To gain full marks for this section the components and concentration/amounts would need to be accurately described. Production of the two products was generally well understood and articulated. A more comprehensive and specific list of indications than rather than “bleeding” or “coagulopathy” was expected. While using elements of the standard “compare/contrast” pharmacology structure was helpful, rigidly adhering to it, for instance, noting FFP’s “poor oral bioavailability” – did not garner marks. It is important to note that heparin is not reversed by FFP and FFP may in fact increase heparin’s effect. FFP does not cause dilutional coagulopathy, it is the treatment for dilutional coagulopathy. Most candidates recognised the need for ABO matching with FFP but not prothrombin concentrates, however few noted that Rhesus matching is not required. The larger fluid load of FFP in comparison to prothrombin concentrates was well recognised a major drawback of FFP use.
3. Briefly outline the major somatosensory pathways of the body (excluding cranial nerves)
CICMWrecks Answer
- Somatic senses: touch, proprioception, pain, temperature
- Somatosensory fibers:
- Non-myelinated fibers (type C): slowest; sense burning pain, hot temperature
- Small myelinated fibers (type Aδ): faster; sense sharp pain, gross touch, cold temperature
- Large myelinated fibers (type A-α; A-β): fastest; sense proprioception, vibration, fine touch
SOMATOSENSORY PATHWAYS
- Carry somatosensory input up spinal cord to brain
- Consist of 4-neuron relay
- 1st order neuron/afferent sensory neuron: has sensory receptors, converts stimuli into impulse
- 2nd order neuron: cell body in spinal cord or brainstem, synapses with 3rd-order neuron
- 3rd order neuron: cell body in thalamus, sends signal to somatosensory cortex
- 4th order neuron/cortical neuron: cell body in sensory cortex
- Includes medial lemniscal/posterior pathway, spinothalamic/anterolateral pathway
MEDIAL LEMNISCAL PATHWAY
- Carries information about fine touch, proprioception
- Large myelinated fibers of 1st order neurons run to spinal cord
- Neurons run through posterior/dorsal funiculus of spinal cord
- Via cuneate fascicle for arms, chest
- Via gracilis fascicle for trunk, legs
- 1st, 2nd order neurons synapse in medulla
- 1st synapse
- 2nd order neurons run to medial lemniscus, decussate; run through pons, midbrain to the thalamus
- 2nd, 3rd order neurons synapse in thalamus
- 2nd synapse
- 3rd order neurons run to sensory cortex in parietal lobe
- 3rd, 4th order neurons synapse in sensory cortex
- 3rd synapse
- Some 1st order neurons synapse with interieurons at posterior horn
- Axons run to anterior horn, synapse directly with motor neuron
- Important for reflexes
SPINOTHALAMIC (ANTEROLATERAL) PATHWAY
- Carries information about pain, temperature, crude touch
- Small/non-myelinated fibers of 1 order neurons run to spinal cord
- Small myelinated fibers: sharp pain, cold temperature
- Non-myelinated fibers: hot temperature, burning pain, crude touch
- 1st, 2nd order neurons synapse in posterior horn of spinal cord/1st synapse
- Small myelinated fibers: enter through dorsal root, bend upwards, travel through two vertebral segments
- Non-myelinated fibers: follow same pathway but synapse with interneurons first, AKA before reaching posterior horn
- 2nd order neurons decussate, cross to anterior horn through central canal
- Neurons then carried through one of two tracts to thalamus
- Lateral tract: carries information for pain, pressure, temperature through lateral funiculus
- Anterior tract: carries information for crude touch through anterior funiculus
- 2nd, 3rd order neurons synapse in thalamus/2nd synapse
- 3rd order neurons run to sensory cortex in parietal lobe
- 3rd, 4th order neurons synapse in sensory cortex /3rd synapse
SOMATOSENSORY RECEPTORS
- Perceive general somatic senses. Includes:
- Mechanoreceptors: for touch
- Proprioceptors: for proprioception
- Thermoreceptors: for temperature
- Nociceptors: for pain
MECHANOSENSORS | Meissner/ tactile corpuscles | – Sensitive to light touch – Encapsulated; located in dermis of hairless skin – Fast adapting; small receptive fields |
Merkel (tactile) discs | – Sensitive to pressure – Non-encapsulated; located in epidermis of hairless skin – Slow adapting; small receptive fields | |
Ruffini (bulbous corpuscles | – Sensitive to skin stretching – Encapsulated; located in dermis of all skin – Slow adaptingl big receptive fields | |
Pacinian (lamellar) corpuscles | – Sensitive to vibration – Encapsulated; located deep in dermis/ subcutaneous tissue of all skin – Fast adapting; big receptive fields | |
PROPRIOCEPTORS | Muscle spindle | – detect when muscle stretched – Located throughout perimysium, AKA connective tissue around muscle cells |
Golgi tendon organ | – Detect when tendon stretched – Located in tendons close to muscle insertion | |
Joint receptors | – Detect joint position, motion – Located in joint | |
THERMORECEPTORS | – Transient receptor potential channels mediate sensations – At extremely cold/hot temperaturs, nociceptors take over | |
NOCICEPTORS | Thermals | sense extremely cold/hot temperatures |
Mechanical | sense excess pressure/deformation | |
Polymodal | Sense combination of both |
Examiner Comments
2022B 03: 35% of candidates passed this question.
Many candidates struggled with this question due to poor structure and limited knowledge with incorrect facts. Good answers were able to outline the various pathways from receptor, through the spinal cord to the higher centres with some detail of each aspect of the pathway whilst highlighting some points of difference between the pathways. For example, information expected regarding the types of receptors involved included, vibration, pain, touch, pressure, thermoreceptors, nociceptors and free nerve endings. Information required for the spinal nerve component would include myelinated versus unmyelinated and linked to the specific receptor, eg myelinated A alpha fibre for assessment of proprioception. As the question specifically asked for more than one pathway those answers describing a single somatosensory pathway failed to score well.
4. List the physiological factors which increase respiratory rate and explain their mechanism
CICMWrecks Answer
Central control of ventilation
- Brainstem
- Medulla (DRG, VRG)
- Pons (Pneumotaxic, Apneustic)
- External inputs
- Sensors from lungs/airways/chemoreceptors
- Cortex
- Can override brainstem function and alter breathing patterns
- Limbic/Hypothalamic Systems
- Alters breathing patters depending on affective states (fear/anxiety/pain)
Peripheral Receptor Inputs alter respiratory rate/depth (ventilation)
- URT receptors (in nose, NP, larynx, trachea)
- mechanical/chemical stimuli
- bronchoconstriction, sneezing/coughing reflexes, and laryngeal spasms
- Joint/Muscle receptors
- limb movement (Eg. exercise) can stimulate ventilation
- Gamma-system (in muscle spindles)
- muscle spindles sense muscle elongation,
- can cause sensation of dyspnoea
- Arterial baroreceptors (in AB and CB)
- ↓ BP causes ↑ ventilation
- ↑ BP can cause ↓ ventilation (and even apnoea)
- Nociceptors
- pain causes apnoea initially, then stimulates ventilation
- Thermoreceptors
- ↑ temperature stimulates ventilation
- ↓ short deep inspiration
Hypocaponea/Hypoxia sensed by Chemoreceptors
- Central CR response
- ↓’d perfusion → ↑[H]/↓PaO2 → CR stimulation
- Afferent signal → Sinus nerve (of herring) / Vagus Nerve → Chemosensitive area of medulla
- Vasomotor area → ↑ peripheral vasoconstriction
- Respiratroy center → ↑rate and depth of respiration → ↑ venous return
- ↓’d perfusion → ↑[H]/↓PaO2 → CR stimulation
- Peripheral CR response
- PCRs found in “Glomus cells”
- ↓ PaO2 – via inhibition of O2 sensitive K-channels
- ↑ PaCO2 and/or ↓pH – via effect on pH sensitive K-channels
- Type I cells (rich in NAd, DA, ACh)
- Hypoxia causes release of NTs
- NAd/ACh – ↑ AP firing rate of AB or CB afferent fibres
- DA – Damping of type 2 cell responses
- Type II cells (rich in capillary supply)
- ↓ PaO2, ↑ PaCO2 and/or ↓pH
- ↓ IC [ATP] which leads to ↑ NT production and release
- ↑ AP firing rate of AB or CB afferent fibres
- PCRs found in “Glomus cells”
Response to:
- ↓ PaO2
- Gradual ↑ MV PaO2 < 500mmHg,
- Rapin ↑MV PaO2 < 50 mmHg
- ↑ PaCO2
- Central CRs (CCRs)
- Most (80%) of response to ↑ PaCO2 by CCRs
- Peripheral CRs
- Response to ↑ PaCO2 is < 20% of total response
- Much FASTER cf. CCR response
- Role is to match ventilation to sudden ∆ in PaCO2
- ↓ blood pH
- Sensed by PCRs in CB only
- Central CRs (CCRs)
CO₂ most important factor

- Minute ventilation is directly proportional to PaCO2 MV ↑ by 2-3 L/min per mmHg PaCO2
- Hypoxaemia has a synergistic effect on hypercapnoeic-ventilatory drive↓ PaO2 causes↑ MV for a given PaCO2,
- ↑ ∆ MV per ∆ PaCO2
O₂ Less important in normal ranges
- PaO2 plays a SMALL role in ventilatory control
- Effect of PaO2 on ventilation:At normal PaCO2PaO2 < 500 mmHg MV ↑ slowly as ↓ PaO2 decrease
- PaO2 < 50 mmHg MV ↑ drastically
- There is synergistic ↑ in ventilatory response in the presence of hypercapnoea and/or acidosis
- MV ↑ drastically when PaO2 is < 100 mmHg

Gladwin 2016
Examiner Comments
2022B 04: 22% of candidates passed this question.
It was expected that candidates would provide not only a list of factors which increase respiratory rate but to give physiological reasoning behind each mechanism. Providing a list of factors was not sufficient for a pass. Vague, imprecise answers attracted fewer marks. A request to explain a mechanism requires the candidates to write a comprehensive physiological reasoning. Many candidates did not demonstrate a comprehensive understanding of these mechanisms. Most answers failed to include all the stimulants of respiratory rate, especially the non-chemical controls. Many answers were not structured or structured in a way that meant they missed many of the mechanisms or that they repeated the same information through the answer which used time but failed to gain additional marks.
5. Outline the structure of fast cardiac sodium channels and describe in detail how they work.
CICMWrecks Answer
Sodium channels
Classified into:
- Voltage-gated sodium channels
- In exitable cells such as myocytes, neurons, certain glia
- Very selective to Na+
- Responsible for rising phase of action potentials
- Triggered by change in membrane potential (voltage)
- Ligand-gated sodium channels
- NMJ – Nicotinic receptors (ACH – ligand)
- Permeable to Na+ and K+
- Triggered by binding of ligands to channel
- Leak sodium channel
- Ungated, always open
- Contributes to depolarization from K+ potential
- Increasing permeability lowers RMP, brining it closer to trigger of an action potential
Voltage-gated Sodium Channel
- Family consisting of Nav 1.1-1.9 encoded by genes SCN1A to SCN9A respectively
- Human Cardiac Sodium channel Nav 1.5 encoded by SCN5A
- responsible for the generation of the rapid upstroke of the myocardial action potential
- determines impulse conduction velocity in cardiac tissue (affects action potential upstroke velocity — extent of intercellular communication via gap junctions)
- Mutations in the gene encoding this channel (SCN5A) linked to three forms of primary electrical disease (channelopathies)
- the long QT syndrome (LQTS)
- the Brugada syndrome (BS) and
- cardiac conduction defects such as broad QRS, LBBB/RBBB, prolonged PR intervals, and other channelopathies like familial AF and idiopathic VF.
Structure
composed of an α subunit (forms ion conduction pore) and one or more auxiliary β subunits (several functions incl. modulation of channel gating)
α subunit
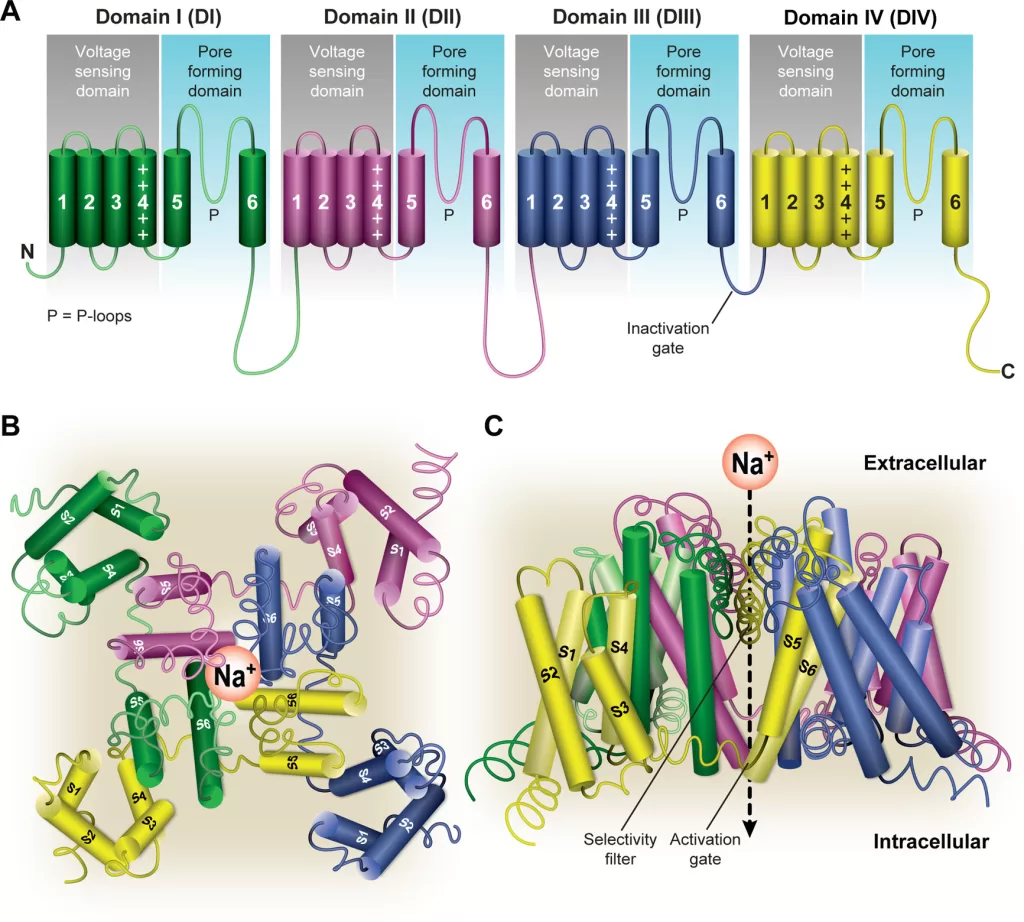
- α subunit is the largest subunit and contains both the pore region of the channel and the voltage sensor
- forms core of the channel and is functional on its own
- indepently able to form a pore in the cell membrane that conducts Na+ in a voltage-dependent way
- When accessory proteins assemble with α subunits, the resulting complex can display altered voltage dependence and cellular localization.
- Principal α-subunit composed of four homologous domains (DI–DIV), each containing six transmembrane segments (S1–S6). The four domains are attached to one another by cytoplasmic linker sequences.
- Highly conserved S4 segment acts as the channel’s voltage sensor
- When stimulated by a change in transmembrane voltage, this segment moves toward the extracellular side of the cell membrane, forming the central pore cavity and allowing the channel to become permeable to ions
- Central Pore Cavity
- Conduct ions
- External portion(i.e., more extracellular): formed by the “P-loops” (the region between S5 and S6) of the four domains. This region is the most narrow part of the pore and is responsible for its ion selectivity (Selectivity filter). Keep out anions and large cations (K+)
- Inner portion (i.e., more cytoplasmic): is the pore gate and is formed by the combined S5 and S6 segments of the four domains.
- Also features lateral tunnels or fenestrations: that run perpendicular to the pore axis. Connect the central cavity to the membrane. Proposed to be important for drug accessibility.
Gating
- have two gates
- Activating gate (m) (voltage-dependent): Opening of the activating gate allows the influx of sodium and cell depolarization.
- Inactivation gate (h) (time-dependent): closing of the inactivation gate will stop the flow of sodium regardless of the status of the activation gate
- Highly conserved S4 segment acts as the channel’s voltage sensor
- When stimulated by a change in transmembrane voltage, this segment moves toward the extracellular side of the cell membrane, forming the central pore cavity and allowing the channel to become permeable to ions
- The cytoplasmic linker between domains III and IV of the α subunit is responsible for inactivation of the Na channel.
- After the channel opens, this segment of the protein is drawn to the inner surface of the channel like a “hinged lid,” – physically wedges the pore gate shut – blocks Na+ ions from passing through the pore
- 3 main conformational states: open, closed, inactivated
- Ion permeable only in open state
- Open: m gates open rapidly
- Closed(resting): m closed, h open
- Inactive:
- Transitions:
- Activation/Deactivation (Closed ⇌ Open)
- Inactivation/Reactivation (Open ⇌ Inactivated)
- Recovery from inactivation/closed-state inactivation (Inactivated ⇌ Closed)
Ionic Events
Action Potential | Membrane Potential | Target Potential | Gates | NA+ / RP | Gate’s Target State | Phase of Cardiac AP |
---|---|---|---|---|---|---|
Resting | −90 mV | −70 mV | m closed | Ready to go | Deactivated → Activated | Phase 4 |
Rising | −70 mV | 0 mV | m open rapidly (0.1-0.2sec) | Na flow in | Activated | Phase 0 |
Rising | 0 mV | +20 mV | h starts closing | Na flow slows | Activated → Inactivated | Phase 0 |
Falling | +20 mV | 0 mV | h closed (takes 10msec) | Na+ channel refractory period starts | Inactivated | Phase 1 |
Falling | 0 mV | −90 mV | h remains closed | RP ongoing | Inactivated | Phase 2,3 |
Undershot | −90 mV | −95 mV | h open, m closes | RP ends | Inactivated → Deactivated | Phase 4 |
Rebounding | −95 mV | −90 mV | m closed | ready to go | Deactivated | Phase 4 |
β subunit
- Four different protein isoforms
- each about one-tenth of the mass of the α subunit
- anchored in the cell membrane through one transmembrane segment
- Extracellular domains are large and have structures similar to those of immunoglobulins.
- Regulate the expression and subcellular targeting of Na channels, and modulate the function (gating) of the α subunit
Examiner Comments
2022B 05: 18% of candidates passed this question.
The expectation for this question was an appropriate description of the fast sodium channel structure included outlining its single alpha and 2 beta sub-units, activation (m) and inactivation (h) gates and sodium selectivity. It was expected that candidates would comment on cycling between the 3 states (resting, open and inactive), corresponding conformational changes to the fast sodium channel, triggers for these changes (voltage or time) and ionic events, including reference to and description of absolute and relative refractory periods. Better candidates were able to relate events to a diagram of a fast action potential, simply drawing a diagram without referencing events at the sodium channel scored few marks. No marks were awarded for description of drug effects on the fast sodium channel. The question specifically relates to fast Na channels, so descriptions of other channels (e.g. ligand gated sodium channels) did not score marks. Details of the subsequent contraction of cardiac muscle following an action potential was also not part of the question and did not score marks.
6. Outline the dose (10% marks), composition (75% marks) and side effects (15% marks) of enteral feeds.
CICMWrecks Answer
Enteral feeding in critically ill
- Primary goal to alter course and outcome of critical illness
- Major goals determined by following principles:
- Catabolism > anabolism. Calorie consumption reduced with better management of critical illness
- Carbohydrates preferred as fat mobilization impaired
- Protein: mitigate breakdown of muscle protein into AA
- Recovery phase: Anabolism>catabolism. Nutritional support provides substrate for anabolic state
Dose
- Mainly observational evidence and clinical experience
- Adjustments can be made based upon an estimate of individuals’s Resting Energy Expenditure (REE) or their body weight
- Adjustment based on body weight, variations based on age, using equations or indirect calorimetry
- Indirect calorimetry
- better than REE equations
- not routinely accessible, not adequately tested
- Predictive equations significant inaccuracy
- Dosing weight is hence routinely based on body weight / BMI with specific adjustments
Dosing weight
- Estimate current or pre-admission dry body weight
- Choose appropriate body weight based on BMI from which to calculate caloric and protein intake
- Underweight (BMI <18.5 kg/m2): current weight as initial dosing weight. Ideal body weight could lead to excess initial calories and induce refeeding syndrome
- Normal weight (BMI 18.5-24.9 kg/m2): current weight as dosing weight
- Overweight (BMI 24.9-29.9 kg/m2): current weight as dosing weight
- Obese (BMI >= 20 kg/m2): Adjusted body weight
- Ideal Body Weight (Devine formula):
- IBW (men) = 50 kg + 2.3 kg x (height, in – 60)
- IBW (women) = 45.5 kg + 2.3 kg x (height, in – 60)
- Adjusted Body Weight
- AdjBW = IBW + (0.4 * [Actual weight – IBW])
- or AdjBW = 1.1 x IBW
- AdjBW = IBW + (0.4 * [Actual weight – IBW])
DAILY NUTRITIONAL REQUIREMENTS
Nutrient | Requirement |
---|---|
H2O | 30-40 mL/kg/day |
Energy | 25-30 kcal/kg/day (can ↑ up to 1.5-1.75x with disease/stress) |
Nitrogen | 0.5 g/kg/day (can ↑ to 1-1.5 g/kg/day with disease/stress) |
Glucose | 3 g/kg/day |
Lipid | 2 g/kg/day |
Na+ | 1-2 mmol/kg/day |
K+ | 0.7-1 mmol/kg/day |
Cl- | 1 mmol/kg/day |
Ca2+ | 0.1 mmol/kg/day |
Mg2+ | 0.1 mmol/kg/day |
PO4 | 0.1 mmol/kg/day |
BUT these vary according to:
- Physiological factors:
- Age, gender, body size
- Pregnancy
- Activity level
- Hydration status
- Pathological factors
- Burns and sepsis → ↑ protein and caloric intake
- Renal failure → ↓ volume, protein and electrolyte (esp K+) content
- CCF → fluid reduction
- Hepatic failure → ↓ a.a/protein content to prevent encephalopathy
- Respiratory failure → ↓ glucose to minimise CO2 production
- Calories
- Fewer calories in the first week of critical illness: approx 8-10 kcal/kg/day
- 25-30 kcal/kg/day in stable patient
- upto 35 kcal/kg/day if weight gain is desired in stable patient and in a lower inflammatory state
- 25kcal/kg/day or less if extubation is imminent
- Protein
- Mild-mod Critical illness: 0.8-1.2 g/kg/day
- Severe Critical Illness: 1.2-1.5kg/day
- Severe burns: upto 2g/kg/day
Composition
FORMULATIONS:
- Common differences between formulas include osmolarity, caloric density, and amount of protein per calorie, as well as electrolyte, vitamin, and mineral content.
- Most are formulated to provide 100 percent of recommended daily vitamin and mineral dose when a minimum of approximately 1000 or more kilocalories are delivered per day.
STANDARD
- Isotonic to serum
- Caloric density of approximately 1 kcal/mL
- Lactose-free
- Intact (nonhydrolyzed) protein content of about 40 g/1000 mL (40 g/1000 kcal)
- Nonprotein calorie to nitrogen ratio of approximately 130
- Mixture of simple and complex carbohydrates
- Long-chain fatty acids (although some are now including medium-chain and omega-3 fats)
- Essential vitamins, minerals, and micronutrients (usually RDA provided in ~ 1000ml of feed)
OTHERS
- CONCENTRATED
- useful in fluid restriction
- mildly hyperosmolar
- caloric density of 1.2,1.5 or 2.0 kcal/ml
- no mortality or morbidity benefit
- PREDIGESTED
- protein hydrolyzed to short-chain peptides, less complex carbohydrates
- reduced total fat, increased MCT or altered TGs
- weakly supported by data
- Potential benefit in: Thoracic duct leak/chylothorax, digestive defects/malabsorbtion syndromes, failure to tolerate standard EN
- Caloric density of 1 or 1.5 kcal/ml
COMPOSITION
- CARBOHYDRATE/FAT
- Standard 49-53% / 29-30%
- Other (not recommended for routine EN):
- Low carb/high fat 28-40%/40-55%
- high carb/low fat 85%/15%
- PROTEIN
- High protein EN (1.2-2g/kg/day) – improved mortality
- Acute/chronic renal disease: Low-protein EN not recommended for routine use. Renal formulas only if difficult to manage volume and electrolytes
- Standard feeds for patients on CRRT
- Peptides – unlikely to be beneficial
- Omega-3 fatty acids and antioxidants, glutamine, probiotics/prebiotics – unlikely to be beneficial, and may be harmful
- Fiber
- no benefit in diarrhoea prevention.
- Mixed fibre feeds may help in persistent diarrhoea
- avoid in pressors/ reduced mobility due to bezoars
- Vitamins and trace elements
- included in feed upto RDA amounts
- Routine supplementation usually not necessary. Definitely donot provide more than RDA
Side Effects
Complication | Mechanism | Management | |
---|---|---|---|
Aspiration | higher risk of pneumonia – causality poorly established | – backrest elevation, post-pyloric feeding – PEG – motility agents (poor evidence in solely reducing aspiration) | |
Diarrhoea | – 15-18% (vs 6% without EN) – alteration of intestinal transit or microflora – commonly associated with medications that can cause diarrhoea (eg antibiotics, PPIs) or suspensions in non-absorbable sugars like sorbitol | – Removal of potential culprit agents – Fiber (use with caution in impaired peristalsis, pressors) | |
Metabolic | Hyperglycaemia | glucose content, insulin resistance | – correction |
Micronutrient deficiencies | usually in malnutrition or GI/renal losses | – supplementation | |
Refeeding syndrome | – rapid changes in fluids and electrolytes – glucose causes release of insulin and intracellular shift of electrolytes – insulin also causes ATP and 2,3-DPG production which utilises phosphate stores – predominantly hypophosphatemia (incl. CV collapse, resp failure, rhabdomyolysis, seizures, delirium) – hypokalemia, hypomagnesemia – Higher risk in acute weight loss, and rapid weight restoration | – reduce nutritional support – aggressive correction of electrolytes | |
Fluid/water | – ~20ml/kg water – potential for water deficit | – assess hydration status – water flushes/ replacement as necessary | |
Mechanical | Constipation | – in feeds with fiber – esp when peristalsis is impaired – impaction, distention, perforation, death | – Careful monitoring and appropriate management |
Fecal impaction | |||
Fiber bezoars | |||
Other | Device related complications | PEG/NG complications related to insertion, dislodgement, mechanical complications |
Examiner Comments
2022B 06: 60% of candidates passed this question.
Generally, most candidates had a reasonable approach to structuring their answers using the headings provided in the question. To score well candidates were required to outline a method of dosing (per body weight, variations based on age, using equations or indirect calorimetry) and describe the composition in terms of the macronutrient daily requirements and energy content as well as other included components (micronutrients). Very few candidates discussed the variations in formulations beyond concentration change nor the reasons for such variations in sufficient detail. Candidates that did not score well were often lacking in detail or missing sections such as side effects or significant elements of the composition. No marks were awarded for content related to the presence of delivery tubes, administration details (e.g. definitions of gastric tolerance, trophic feeding, commencement rate) or for details that related specifically to parenteral nutrition.
7. Describe the physiological mechanisms by which the kidney is able to maximally concentrate urine.
CICMWrecks Answer
Medullary concentrating gradient
- physiological process which sets up a concentration gradient from cortex through to medulla
- allows formation of concentrated urine
- Mechanisms:
- Counter current Multiplier: creates concentrated medullary interstitium
- Counter current Exchange (vasa recta): maintains intersisital osmotic gradient
- Recycling of urea: contributes to high osmolarity of medullary interstitium
- Normal cortico-medullary gradient 300-1400mOsmol.
Counter-current Mechanism in Kidney
Counter Current Multiplier:
Set-up
- Descending limb LoH Permeable to water only
- Ascending limb LoH Permeable to NaCl only
- Thin passive NaCl reabs
- Thick NaCl via active NKCCT cotransport and paracellular
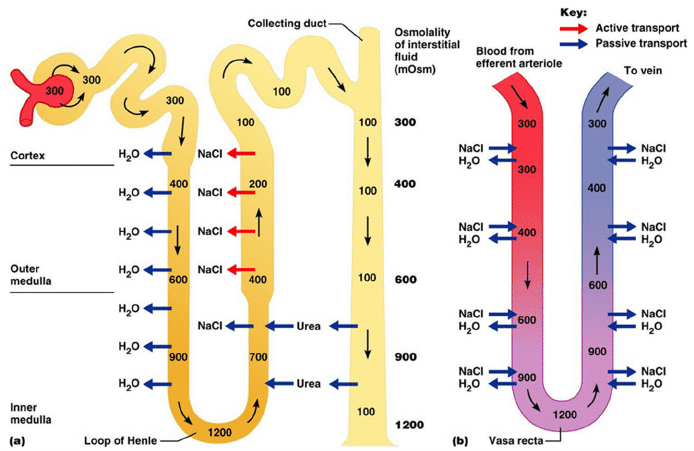
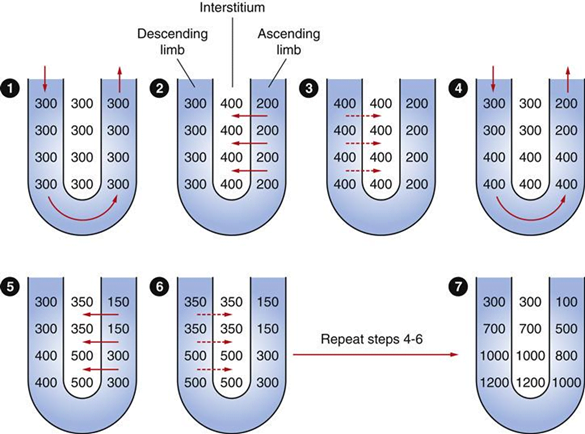
Generation
- Enters LoH osmolarity throughout = 300mOsm
- Max effort of NKCCT = 100mOsm/kg
- Tubular OSM = 200/kg
- Interstitial Osm = 400/kg
- Descending limb diffuses water down gradient
- Descend limb OSM now 400
- Process repeats (flow, pump salt, equilibrate water)
Counter Current Exchange:
- Hairpin loop arrangement of the vasa recta in the juxta-medullary nephrons.
- Provides blood flow to medullary tissue without impact of the cortico-medullary gradient.
- Relies on slow flow of blood.
- Mechanism
- On descent, water is lost from capillaries and NaCl is absorbed into capillaries increasing osmolarity
- On ascent water is reabsorbed and solute is lost
Role of Urea in Medullary Concentration Gradient
- 900mmol/day filtered
- 350-550mmol/day reabsorbed.
- Comprises 50% of osmolality (300-650 mOsm/kg)
- Freely filtered at the glomerulus
- Secreted by thin limbs of LoH down concentration gradient from medullary interstitium to tubular fluid.
- As water and Na are reabsorbed, tubular [Urea] increases
- [Urea] = >500mmol/L at collecting ducts
- Equilibrates with interstium due to slow flow rates.
- ↑ADH
- urea transporters UT1 and UT3 insertion → ↑ CD permeability to urea
- → ↑ medullary gradient (further 10% reabsorption if required)
Gladwin / JC 2020
Examiner Comments
2022B 07: 35% of candidates passed this question.
Answering this question well required the demonstration of understanding of the concept of achieving maximum urinary concentrating ability. Answers required a description of the usual concentrating processes and the changes that would occur in circumstances where maximally concentrated urine would be made. A key concept was the creation of a medullary concentration gradient to allow water reabsorption independent of solute reabsorption. This required an explanation of the contribution of the loop of Henle, the vasa recta, and urea cycling in the creation and maintenance of this gradient, along with the impact of ADH. More detailed explanation of each contribution was required as overarching statements were not sufficient to attract all marks for each section. Answers that focused solely on the counter-current exchange and multiplier process were insufficient on their own to achieve a passing mark. The examiners commented that a significant proportion of candidates excluded the role of urea in their answers.
8. Classify calcium channel blockers providing examples (15% marks). Describe the pharmacology of verapamil (85% marks).
CICMWrecks Answer
Calcium Channel Blocker Classification
- Class I – Phenylalkylamines:
- Verapamil
- Reduces HR, contractility and causes vasodilatation, decrease in cardiac contractility, heart rate, and both coronary and peripheral vascular tone.
- Class II – Dihydropyridines:
- main effects are on vasodilatation
- 1st gen = nifedipine
- 2nd gen = felodipine, nimodipine
- 3rd gen = amlodipine
- Class III – Benzothiazepines:
- diltiazem
- some reduction in HR and contractility, also causes vasodilatation, mild to moderate decrease in myocardial contraction, and decreases heart rate by decreasing both the automaticity of the SA node and the rate of conduction in the AV node.
Calcium Channel Blocker Pharmacology
(Include as per the question)
Click here to Open CICMWrecks Table: Calcium Channel Blockers
Class Effects:
- Heart
- V-W Class 4 antiarrhythmic
- Decr SA node automaticity
- Decr AV node conductivity
- Vasodilation
- Negative inotropy
- reflex tachycardia
- 2) Vasculature
- Decr SVR and BP
- Decr Hypoxic pulmonary vasoconstriction
- Incr coronary and cerebral blood flow
- 3) Other
- Tocolytic
- NDMR potentiation.
Gladwin / JC 2021
Examiner Comments
2022B 08: 35% of candidates passed this question.
Most candidates provided an acceptable classification of calcium channel blockers. Candidates are encouraged to read the question as a few failed to provide relevant examples with their classification. A basic understanding of the common features of verapamil (indeed most of these are common to the calcium channel blockers as a group) in clinical practice and toxicology was sufficient to pass. Marks allocated to dosing and presentation were not gained for writing that it is available as tablets or as a clear colourless solution, unless relevant information was given. Many candidates listed neuropsychiatric effects of verapamil, which are not a feature normally associated with calcium channel blockers. Many also listed QT prolongation among its effects, which is not listed in the standard texts and is the opposite to the true effect. The examiners noted that in some papers there appeared to be a contradiction between the effect of Verapamil on the cardiac action potential and the detail provided about the drugs clinical effects, these answers demonstrated a lack of solidity in the understanding of the underlying physiology.
9. List any five classes of antibiotics with anti-staphylococcal activity and provide one example of each class. Outline the mechanism of action and side effects of the five drugs.
CICMWrecks Answer
Anti-staphylococcal antibiotics
Class | Antibiotic | Mechanism of Action | Side Effects |
---|---|---|---|
Penicillin | Flucloxacillin | Beta lactam ring binds to penicillin binding protein (transpeptidase) and prevents crosslinking of bacterial peptidoglycan inhibits cell wall synthesis Bacteria eventually lyse due to ongoing activity of cell wall autolytic enzymes (autolysins and murein hydrolases) while cell wall assembly is arrested Less bactericidal but stable to staph beta-lactamases | Up to 10% of the population have allergies to penicillins. Due to the high percentage excreted renally unchanged dose adjustment is required in low urine output states. Severe cholestatic hepatitis has been reported idiosyncratically. |
Glycopeptide | Vancomycin | Inhibits Glycopeptide synthetase prevents peptidoglycan formation in bacterial cell well (Unlike penicillins, prevents the transfer and addition of the muramylpentapeptide building blocks that make up the peptidoglycan molecule itself.) May also alter membrane permeability and selectively inhibit RNA synthesis. Antimicrobial activity Dependent on Duration above MIC, not concentration | Hypersensitivity reactions including anaphylaxis. Rapid infusion is associated with histamine release – red-man syndrome. Ototoxicity rare, dose related. Nephrotoxicity has declined with improved formulations, usually resolves with cessation of drug |
Cephalosporin | Cefazolin | Beta lactam ring binds to multiple penicillin binding proteins (carboxy/endo/ transpeptidase) and prevents crosslinking of bacterial peptidoglycan inhibits cell wall synthesis BROADER SPECTRUM Bacteria eventually lyse due to ongoing activity of cell wall autolytic enzymes (autolysins and murein hydrolases) while cell wall assembly is arrested Configuration: Stability against Betalactamases | Generally well tolerated Hypersensitivity reactions (10% cross reactivity with penicillin allergies), thrombophlebitis, interstitial nephritis, low prothrombin, flushing and headaches with EtOH. |
Lincosamide | Clindamycin | Inhibitor of bacterial 50S ribosomal subunit Prevents protein synthesis | – Pseudomembranous colitis due to c.diff – Contact dermatitis – Metallic taste – Anaphylaxis |
Linezolid | Oxazolidinone | inhibits bacterial protein synthesis by binding specifically to the 50s ribosomal subunit, thereby preventing initiation complex formation. | Headache, LFT derangement, Taste Alteration, GI disturbances Skin/bleeding problems, phlebitis, pancreatitis |
Daptomycin, Rifampicin
Examiner Comments
2022B 09: 82% of candidates passed this question.
In general, the question was well answered. Better answers provided examples of antibiotics from different categories of mechanism of action and were able to describe specific side effects relevant or unique to that antibiotic. Mechanisms of action that were generic in terms of site of action attracted fewer marks. Some examples of antibiotics that candidates provided were not anti-staphylococcal antibiotics (eg; Benzyl Penicillin, Clavulanic acid). The examiners commented that the use of a tabular format as an answer template resulted in answers scoring marks in most time efficient manner. Frequent omissions or commissions resulting in lost marks noted by the examiners were as follows; many papers showed a lack of detail regarding the precise mechanism of action of penicillins particularly around inhibition of transpeptidases whilst the use of non-specific side effects such as nausea vomiting and diarrhoea did not attract marks.
10. Draw a labelled diagram of both the aortic root and radial artery pressure waveforms in a young adult using the same axis (60% marks). Explain the factors that account for the differences between these two waveforms (40% marks).
CICMWrecks Answer
The arterial pulse waveform:
- The arterial pulse waveform can be separated into three distinct components
- The systolic phase, characterised by a rapid increase in pressure to a peak, followed by a rapid decline. This phase begins with the opening of the aortic valve and corresponds to the left ventricular ejection
- The dicrotic notch, which represents the closure of the aortic valve
- The diastolic phase, which represents the run-off of blood into the peripheral circulation.
Windkessel effect
is a term used in medicine to account for the shape of the arterial blood pressure waveform in terms of the interaction between the stroke volume and the compliance of the aorta and large elastic arteries (Windkessel vessels) and the resistance of the smaller arteries and arterioles.
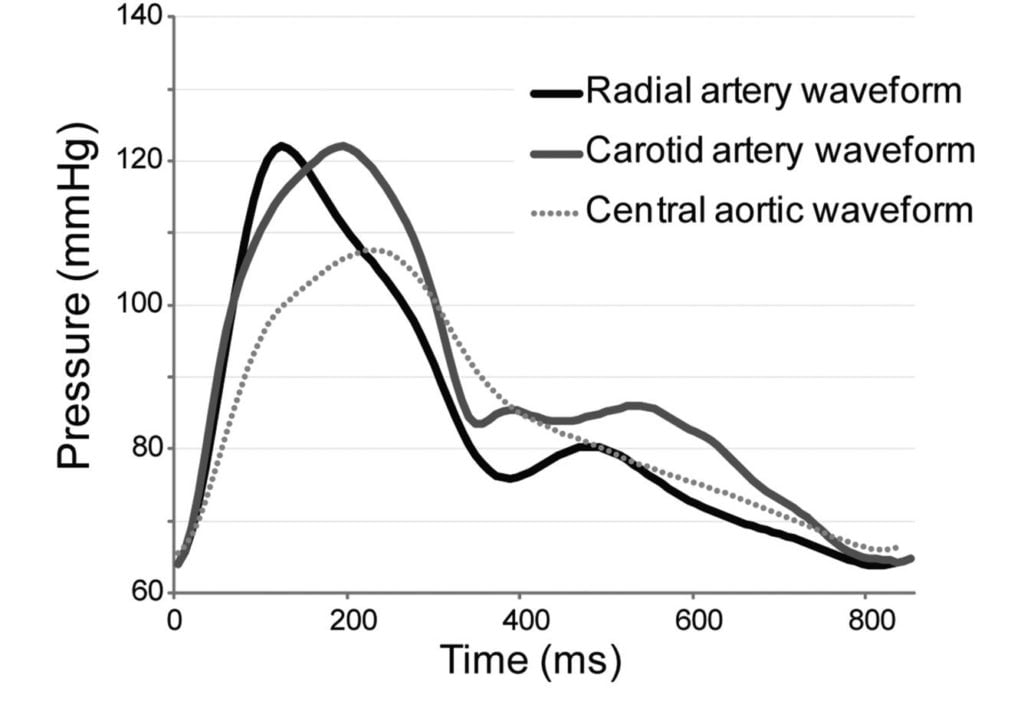
The further you get from the aorta
- The taller the systolic peak (i.e. a higher systolic pressure)
- The further the dicrotic notch
- The lower the end-diastolic pressure (i.e. the wider the pulse pressure)
- The later the arrival of the pulse (its 60msec delayed in the radial artery)
Waveform Difference: Radial vs Aortic
Radial waveform (vs Aortic) | Reason |
---|---|
Delayed start 60msec | · Time taken for wave front to travel (note much faster than blood flow) |
Narrower and higher systolic component Steeper upstroke = ↑dp/dt max | · Less elastic tissue · Relatively more muscular tissue · ↓ compliance (δv/δp) |
Lower diastolic component | · Less elastic tissue · Less Windkessel effect (storage and release of energy) |
Slightly lower mean | · Above factors |
Widened pulse pressure | · Above factors |
Disappearance of high freq components | · Damping affects high > low freq · Damping ∝ frictional tissue interactions per length or time |
Appearance of dicrotic notch | · Reflection and resonance. (peripheral dicrotic notch owes more of its shape to the vascular resistance of peripheral vessels than to the closing of the aortic valve) |
Source: Deranged Physiology, Ketamine nightmares
Examiner Comments
2022B 10: 21% of candidates passed this question.
This question required the integration and understanding of cardiovascular monitoring with what influences the changes as the arterial pulse moves through the vascular tree. In general, the waveforms drawn were often of a poor standard, with little attention given to accurately representing the different shapes, correct axis (pressure and timing) and labelling. The description of the differences was of a better standard however, there were often contradictions between what was drawn and described. For example, several answers correctly described a delay in the systolic peak seen in the radial artery, but this was not illustrated in the diagram. The windkessel effect was often correctly mentioned but not further explained to help describe the changes seen in the waveforms. Interruptions to the arterial traces from the anacrotic and diacrotic notches, the origins of the diacrotic hump, and insuria were often incorrect and poorly explained. The examiners reported that answer template structure was not a major issue, but a lack of integration of the waveform and translation of that knowledge into a description of the cardiac cycle was often poorly done.
11.Describe the body fluid compartments.
CICMWrecks Answer
DEFINITION
Body fluid compartment is defined as collections of physiologically significant fluid characterised as →
(i) being easily defined, largely separate from another compartment by some form of physical barrier
(ii) having a similar composition within the compartment
(iii) behaving predictably to certain fluid interventions
DISTRIBUTION OF TOTAL BODY WATER (TBW)
- TBW is 60% of body weight (42 L in 70 kg ♂ adult) → BUT this varies according to:
- Body fat content – ↑ fat content → ↓ %TBW of body weight
- Age – Aging causes ↓ %TBW of body weight (due to ↑ fat content)
- Gender – ♀ TBW is 55% body weight (due to ↑ fat content)
- TBW is broken into various “body fluid compartments” as per the table below:
COMPARTMENT | % TBW | Volume (L) | % Body Weight | |
---|---|---|---|---|
TBW | 100% | 42 L | 60% | |
ICF | 55 | 23 | 33 | |
ECF | 45 | 19 | 27 | |
ECF Components | Interstitial Fluid | 20 | 8.4 | 12 |
Plasma Volume | 7.5 | 3.2 | 4.5 | |
Dense CT H2O | 7.5 | 3.2 | 4.5 | |
Bone H2O | 7.5 | 3.2 | 4.5 | |
Transcellular fluid | 2.5 | 1 | 1.5 |
Composition
ICF | ECF | |
---|---|---|
Consists of all fluids within the cell membrane | consists of all fluids outside the cell membrane | |
% TBW | 55% (23L) | 45% (19L) |
% Body Weight | 33% | 27% |
Mainly | Mainly K+ (150 mmol/L), PO43- (100 mmol/L) and proteins (+++) | Mainly Na+ (140 mmol/L) and Cl- (100-105 mmol/L) |
Smaller amounts | Na+ (10 mmol/L) Cl- (3 mmol/L) HCO3- (10 mmol/L) Mg2+ (0.5 mmol/L) Ca2+ (< 0.01 mmol/L) | HCO3- (27 mmol/L) K+ (3.5-5 mmol/L) Ca2+ (1.1 mmol/L) PO43- (1.1 mmol/L) Mg2+ (0.5 mmol/L) protein (+) |
Osmolality | 290 mosm/kg H2O | 290 mosm/kg H2O |
does NOT exist not a single united fluid compartment → exists as 1014 discrete separate packets (cells) of solution |
Extracellular fluid (ECF) Sub-compartments
Compartment | TBW | %Bdy Wt | |
---|---|---|---|
Interstitial fluid | 20% (8.4L) | 12% | Fluid that bathes all cells in body and links their ICF with Plasma volume (PV) |
role in transfer of metabolic substrates (O2/nutrients), waste products and chemical messengers | |||
Similar composition to PV → BUT very ↓ protein composition cf. PV | |||
Includes “lymph” → role in returning excess ISF and protein to circulation | |||
Plasma fluid | 7.5% (3.2L) | 4.5% | Role in transport function within body (Ie. of metabolic substrates, waste products, chemical messengers) → relies on high “bulk flow” |
Similar composition to ISF → BUT ↑↑↑ protein content | |||
Dense Connective tissue (CT) H2O | 7.5% (3.2L) | 4.5% | |
Bone H2O | 7.5% (3.2L) | 4.5% | |
Transcellular fluid | 2.5% (1L) | 1.5% | Diverse group of small fluid collections |
Eg. CSF, bile, joint fluid, aqueous humour, bowel fluid, bladder urine, body cavity fluids, Etc. | |||
This type of fluid is specially characterised by: – having special physiological roles in body – being in contact with ICF across an epithelial cell membrane (rather than ISF), – and being formed by specific cellular transport activity in epithelial-lined spaces |
There are two groups of ECF:
- “Functional ECF” (PV + ISFV → 27.5% TBW)
- Kinetically active (fast) ECF → vital in determining compartment distribution of acutely infused fluids
- Explains 2:1 ECF:ICF ratio with acute IVF intervention (as functional ECF:ICF ratio, rather than total ECF:ICF ratio which is 55:45)
- “Non-functional ECF” (TCF + bone H2O + dense CT H2O → 17.5% TBW)
- Kinetically inactive (slow) ECF → minor role in determining compartment distribution of acutely infused fluids
Blood volume
- Blood volume (5 L) consists of BOTH ECF and ICF compartments → PV from ECF (3.2 L) and red cell volume from ICF (1.8 L)
- Blood volume = PV (from ECF) + red cell volume (from ICF)
Factors controlling distribution of TBW
- All body fluid compartments are isotonic (or iso-osmotic in terms of “effective”
osmoles) as H2O easily and rapidly moves (via osmosis) across cell membranes - Distribution of TBW in body fluid compartments is due to various factors (see
below) that cause H2O to shift across membranes into a specific compartment
TBW distribution between ICF and ECF
→ determined by ECF content of Na+ because:
- ECF Na+ content is main determinant of ECF osmolality → this is because
Na+ and its associated anion (Cl-) are the main osmotically active solutes in ECF (90% ECF osmolality) → but since ∆ in ECF Cl- content occur 2° to ∆ in ECF
Na+ content, so ECF Na+ content is the true determinant of ECF osmolality- Remember → ECF Na+ is controlled by arterial, venous and cardiac baroreceptors that measure ECFV (which is determined by ECF Na+)
- ECF osmolality determines TBW distribution between ECF and ICF → this is
because cell membranes are H2O-permeable and thus ∆ ECF osmolality cause
H2O movement across it via “osmosis” until both ICF and ECF osmolalities
equalise (Ie. hypertonic ECF draws H2O out from ICF until ICF/ECF osmolality
are equal)- Remember → Semi-permeable membrane (permeable to H2O but impermeable to most solutes) allows osmotic gradients to develop on both sides of the membrane.
“Osmosis” is a process where H2O passively diffuses across the membrane from ↓ to ↑ osmolality until osmolality is same on both sides of the membrane
- Remember → Semi-permeable membrane (permeable to H2O but impermeable to most solutes) allows osmotic gradients to develop on both sides of the membrane.
TBW distribution between ISF and intravascular compartments
→ determined by “Starling forces” → balance of PHYDROSTATIC and PONCOTIC across the capillary membrane determines net ultrafiltration of H2O into ISF:
- Hydrostatic pressure gradient (PIV – PISF) → PIV at arterial end and venous end of capillary is 35 mmHg and 15 mmHg, respectively, while PISF is 0 mmHg → thus, a PHYDROSTATIC gradient exists favouring net H2O filtration into ISF (decreasing from 35 mmHg at start of capillary to 15 mmHg at the end)
- Oncotic pressure gradient (πIV – πISF) → plasma colloids (esp plasma proteins) cannot cross capillary membrane into ISF, so [protein] in capillaries is >> ISF (Ie. 80 vs 20 g/L) → πIV >>. πISF (28 mmHg vs 3 mmHg) → constant but small net oncotic pressure gradient throughout capillary length favouring H2O retention in intravascular space
- KF → = capillary surface area (↑↑↑) and its hydraulic permeability (membrane is semi-permeable → permeable to H2O and most solutes EXCEPT large proteins)
- σ → Reflection coefficient
- Thus → NFP at arterial end of capillary is +10 mmHg (favouring filtration in interstitial space) while at venous end it is – 10 mmHg (favouring reabsorption in capillary) → there is net 2 mL/min fluid filtered into interstitial space
TBW distribution between ECF sub-compartments
→ determined by:
- Starling forces → balance of PHYDROSTATIC and PONCOTIC across the membrane determines ultrafiltration of H2O across compartments (as above)
- Active and passive transport of solute across the membrane (Eg. passive diffusion, facilitated transport, active transport) → influences osmotic gradient across the membrane and causes osmosis of H2O between compartments
- Gibbs-Donnan effect → non-diffusible ion in one compartment causes equal concentration ratios of diffusion ions across the membrane at equilibrium → results in ↑ osmotic pressure in compartment with non-diffusible ion → leads to osmosis of H2O into it
Source: Bianca’s notes
Examiner Comments
2022B 11: 25% of candidates passed this question.
This question was answered poorly. Many answers provided incorrect facts relating to the body fluid compartments, their approximate sizes, the factors that regulate and contribute to those sizes and their constituents. Total body water was often incorrectly calculated and associated with limited explanation as to the factors that may affect it.
12. Describe the effects of ageing on the cardiovascular system.
CICMWrecks Answer
Ageing = physiological time-dependent process which results in ↓ cellular function + ↓ reserve
- Heart
- ↑ fibrous infiltration of myocardium → ↓ ventricular compliance (esp LV > RV)
- ↓ # myocytes but compensated by hypertrophy → ↑ myocardial wall thickness (concentric) → ↓ ventricular compliance (esp LV > RV)
- ↑ fibrous infiltration of endocardium → ↓ ventricular compliaince (esp LV > RV)
- Calcification of heart valves → valvular incompetence (stenosis/regurgitation)
- Conducting system (Ie. SAN, AVN, bundle of His, Purkinje):
- ↑ fibrous/fatty infiltration
- ↑ loss of pacemaker cells
- ↓ # and sensitivity of β receptors within pacemaker cells
- Peripheral vasculature:
- ↓ elasticity of large arteries due to thickening and calcification of vessel wall
Change with ageing | Reason | |
---|---|---|
HR | Resting HR → unchanged ↓ maximum HR (Max. HR = 220 – age) ↑ arrhythmias (AF, AV heart block, VEBs, BBB) | Conduction system (SAN, AVN, bundle of His, Purkinje) → fibrous/fatty infiltration and loss of pacemaker cells ↓ # and sensitivity of β receptor of pacemaker cells |
SV | ↓ resting SV ↓ maximum SV | ↓ ventricular compliance (2° to fibrous infiltration of myocardium/endocardium, and ventricular hypertrophy) Valvular disease |
CO | ↓ due to preconditioning (Ie. sedentary lifestyle) or age-related disease Unchanged in “healthy” subjects Nb. ↑ C.O. occurs mainly by ↑ SV (preload) via Frank-Starling mechanism (rather than ↑ HR) | ↓ HR – see above ↓ preload (↓ SV) – see above ↑ afterload (↑ SVR) – see below ↑ reliance on “atrial kick” (30% of C.O. vs 5% in adults) → AF/↑HR not well tolerated |
BP | ↑ MAP and SBP DBP ↑ slightly (until age 60), then ↓ ↑ pulse pressure (due to ↑ SBP > DBP) | ↓ elasticity of large arteries due to thickening and calcification of vessel wall |
Pulmonary circulation | ↑ PAP a/w ↑ PVR | |
Control of CVS | ↓ maximum exercise tolerance (due to age-related ↓ maximum HR, SV, C.O.) Impaired BRR → postural hypotension ↓ β receptor response of CVS to catecholamines (↓ # or affinity of receptor, ↓post-receptor signalling, ↓ myocardial contractile response with stimulation) ↑ vagal tone |
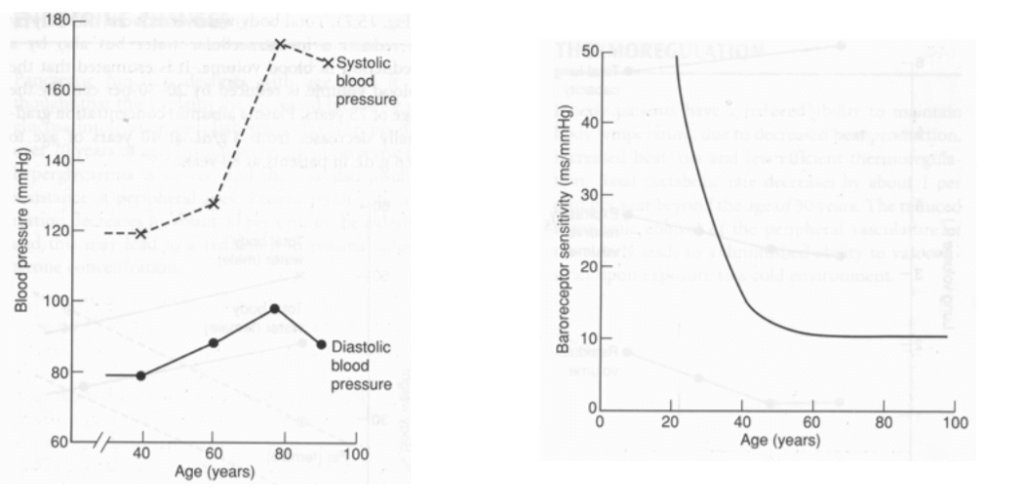
Bianca 2016
Examiner Comments
2022B 12: 46% of candidates passed this question.
The physiological changes associated with ageing are well described in the major texts although candidates do need to assimilate this information from a number of sources. Core components of the answer templates that were frequently missed included those of; concentric hypertrophy and its effects on afterload, as well as the difference between compliance, elastance, elasticity, elastin and collagen and how ageing affects these elements. A template using broad headings such as the effects on the heart/myocardium, the vasculature, the autonomic nervous system, the conduction system and perhaps epithelial function would be a good starting point when constructing an answer to this question. The examiners noted that several candidates wrote at length about various pathologies that increase in incidence with ageing which does not adequately address the core of the question.
13. Compare and contrast the pharmacology of EPHEDRINE and METARAMINOL.
Examiner Comments
2022B 13: 27% of candidates passed this question.
The ‘compare and contrast’ pharmacology question indicates the use of a standardised structure that incorporates pharmaceutics, pharmacokinetics and pharmacodynamics. The best answers provided excellent detail, ie. precise descriptions of mechanisms of action and emphasised noteworthy areas of contrast between the two drugs. Highlighting opportunities for use and areas of caution/drug limitations.
Overall, most candidates seemed to have a sufficient knowledge of metaraminol but details surrounding ephedrine were often lacking.
14. Describe the physiological role, distribution and regulation of potassium ions (K+ ).
CICMWrecks Answer
Potassium
- Predominany intracellular cation
- Total Stores: Approx 3200mmol (50mmol/kg)
- Key Functions:
- Main determinant of ICF osmolality and tonicity
- Responsible for RMP of excitable cells via Goldmann-Hodgkin-Katz due to ↑↑gK relative to other species
- Role in action potential → repolarisation phase
- Secretion of insulin and multiple other KATP dependant processes
- Regulation of IC processes (protein/glycogen synthesis)
- Involved in Na+/K+ ATPase in cell membranes
Potassium balance / Determinants of Serum Potassium
- Intake
- Oral – very variable
- Approx. 50-200mmol/day
- Distribution
- Exchangable Pool
- ICF (90%): [K+] 150 mmol/L (1° ICF cation)
- ECF (2%): [K+] 3.5-5 mmol/L
- Non-exchangable Pool
- Bone (8%)
- Exchangable Pool
- Transcellular balance
Factor | Mechanism | Effect on Serum K+ |
---|---|---|
Insulin | Causes intracellular shift of K+ | ↓ |
β2 adrenergic agonism | Causes intracellular shift of K+ | ↓ |
Aldosterone | Upregulate Na/K ATPase → Intracellular shift of K+ | ↓ |
pH | Increased H+ (decreased pH) causes extracellular shift of K+ | ↑ |
Plasma osmolarity | Hyperosmolar plasma initiall causes osmosis of water out of cells increasing intracellular osmolarity and [K+] → causes extracellular shift of K+ | ↑ |
Skeletal muscle activity | Causes K+ leakage into serum | ↑ |
Cell death | Causes K+ leakage into serum | ↑ |
- Elimination
- Faecal – 8mmol/day
- Renal – 92mmol/day (See next heading for details)
- Urinary K+ excretion = [K+ filtered by glomerulus] + [K+ secreted by CCD/LDCT] – [K+ reabsorbed by renal tubules]
- Glomerular filtration: Freely filtered = 756mmol/day (180L x 4.2mmol/L)
- PCT: 65% reabsorption
- LoH: 25~30% reabsorption
- DCT/Collecting Ducts – variable
- Determined by Aldosterone, Plasma [K+], Tubular Flow rate
- Secreted by principal cells
- Reabsorbed by intercalating cells
Renal K+ regulation:
- Renal K+ regulation occurs MAINLY via control of K+ secretion at the distal nephron (CCD and LDCT) via the following factors:
- Circulating factors:
- Plasma [K+ ]
- ↑ [K+ ] causes ↑ K+ secretion by → (i) Directly stimulating Na+ /K+ ATPase in principal cells, and (ii) Directly stimulating Aldosterone release from adrenal cortex
- Aldosterone
- ↑ aldosterone causes ↑ K+ secretion by → ↑ production of Na+ /K+ ATPase, K+ channels and ENaC Na+ channels in the principal cells
- Plasma pH
- Alkalosis via ↓ plasma [H+ ] causes ↑ K+ secretion by → stimulating Na+ /K+ ATPase in principal cells
- Plasma [K+ ]
- Luminal factors:
- Flow of tubular fluid in DCT and CCD
- ↑ tubular fluid flow causes ↑ K+ secretion by → maintaining ↓ luminal [K+ ] (Ie. continuously washing it away) → permits passive diffusion of K+ ↓ its [ ] gradient into tubular lumen
- ↑ Na+ delivery rate to DCT and CCD → ↑ K+ secretion
- -ve lumen potential difference → ↑ K+ secretion
- Flow of tubular fluid in DCT and CCD
- Circulating factors:
JC / Gladwin / Sakurai 2020
Examiner Comments
2022B 14: 51% of candidates passed this question.
The best answers demonstrated an appreciation of the multiple roles of potassium in normal physiology and described the integrated regulation of potassium concentration/distribution as opposed to many answers that seemed to focus purely on the renal handling of a filtered potassium load.
15. Describe the anatomical (20% marks) and physiological (80% marks) features of the pulmonary circulation.
CICMWrecks Answer
Anatomy
- Thin walled arteries and veins, contains little smooth muscle → easily distorted by lung expansion
- Pulmonary arteries (deoxygenated blood) -> pulmonary capillaries (gas exchange) → pulmonary veins (oxygenated blood)
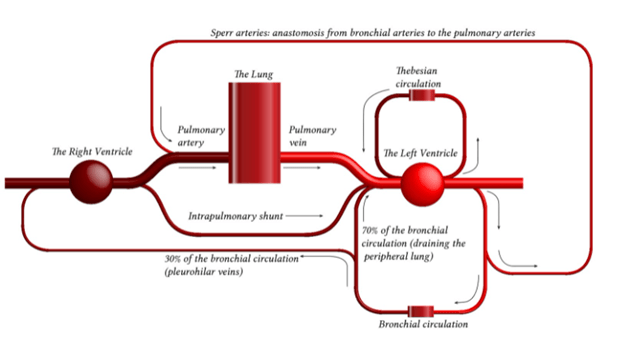
- Pulmonary capillaries are exposed to alveolar pressure and not supported by solid tissue → prone to collapse
- Contains 10% of circulating blood volume – 500mls
Physiology
- General:
- Low pressure 25/8 MPAP 15mmHg
- Low resistance 20-120 dynes.sec.cm-5
- Flow: total of cardiac output 5L/min
- Pulmonary blood volume
- ~500mls of blood – blood reservoir
- Varies over course of the respiratory and cardiac cycle and in response to gravity
- Varies in response to changes in intrathoracic pressure
- Pulmonary blood pressure
- Normal PA systolic pressure = 18-25 mmHg
- Normal PA diastolic pressure = 8-15 mmHg
- Normal mean pulmonary arterial pressure = 9-16 mmHg
- Capillary pressure is 8-10 mmHg
- Venous pressure 6-12 mmHg
- Resistance
- The resistance in the pulmonary circulation can be calculated as follows:
- PVR = 80 × (mPAP- PAOP)/CO
- The normal value for PVR is 100-200 dynes/sec/cm-5
- Approximately 1/10th of systemic circulation
- Regulation of blood flow/resistance
- West zones
- In zone 1, PA > Pa > Pv No flow of blood
- In zone 2, Pa > PA > Pv Resistance to flow is determined by alveolar pressure (Starling resistor effect)
- In zone 3, Pa > Pv > PA Resistance to flow is determined by venous pressure Venous pooling causes increased distension of pulmonary capillaries
- In zone 4 Low lung volume causes narrowing of extra-alveolar vessels
- Hypoxia – pulmonary hypoxic vasoconstriction
- Lung volumes
- West zones
- Regional distribution
- Distension of partially collapsed capillaries
- Recruitment of completely collapsed capillaries
- Local mediators: NO, prostacycline
- Function
- Gas exchange
- Filtration of clots and debris
- Immunological – pulmonary macrophages, IgA production
- Metabolic – metabolism of drugs, removal of proteases
- Endocrine – source of ACE
Guo 2021
Examiner Comments
2022B 15: 23% of candidates passed this question.
Many candidates described the anatomical pattern of right ventricle to arteries to smaller arteries to arterioles to capillaries to venules to veins to the left atrium. To obtain full credit one needed to describe relevant aspects of anatomy including main pulmonary artery ~5cm in length divides into L and R pulmonary arteries. Arteries are relatively thin walled with little smooth muscle, capillaries form an extensive sheet of blood flow over the alveolar wall, and the pulmonary circulation drains into four pulmonary veins that empty into the left atrium. A structured approach would then follow regarding physiology. There is ~500 ml of blood in the pulmonary circulation with ~10% in the capillaries and half the remainder in each of arteries and veins. The system has a high capacitance and is very distensible. The volume can halve or double to adjust for posture, respiratory effort and changes to the systemic circulation. Values for normal pulmonary artery pressure were expected and an explanation that this is just adequate to reach the apices of the lung and that if the pulmonary pressure was higher there would be a risk of compromised perfusion and flow. Comparisons with the systemic pressures or detail such as capillary values gained extra credit. For example, highlighting that regional distribution and regulation is relatively passive compared to the systemic circulation and thus gravity and posture have significant effects. Many candidates gave the units incorrectly. Of the candidates who described the West Zones, most seemed aware of the influence of alveolar pressure, but few seemed aware of the importance of low pulmonary pressure relative to the effect of gravity, such that the pulmonary arterial pressure was just adequate to reach the apex of the lung. Most candidates were aware of the important role of hypoxic pulmonary vasoconstriction to optimise VQ matching. The autonomic system has relatively little effect upon regulation especially compared to the systemic circulation. Many candidates wrote in generalities about the physiology of circulatory systems without discussing special features of the pulmonary circulation. The detail supplied was often less than the expected level.
16. Describe how the values for PaO2, PaCO2, pH and bicarbonate are determined on a blood gas sample.
CICMWrecks Answer
Blood Gas: pO2
- Measured using Clark Electrode
- Platinum cathode
- Coated in gas permeable membrane à permeable to O2
- Membrane impermeable to other oxidizing molecules
- O2 + 4e– → 2O–
2O– + 2H2O → 4OH–
- Silver chloride anode
- 4Ag → 4Ag+ + 4e–
4Ag+ + 4Cl– → 4AgCl
- 4Ag → 4Ag+ + 4e–
- Phosphate buffer with KCl
- Platinum cathode
- Cathode voltage potential to -0.65v
- In absence of O2 current = 0v
- In presence of O2, O2 diffuses through gas-permeable membrane and is reduced, absorbing 4e–
- Micro-ampmeter measures movement of electrones between anode and cathode
- The current produced is proportional to the pO2 of the test solution
- Sensitivity – related to thickness of membrane and size of cathode area
Blood Gas: pCO2
- Measured using Stowe-Severinghaus Electrode
- Modified pH electrode with outer semi-permeable membrane (Teflon or Silicon Elastic)
- CO2 diffuses into electrolyte layer
- CO2 + H2O → H2CO3 → H+ + HCO3+
- H+ diffuses across glass electrode and alter pH
- Change in pH measured
- Henderson-Hasselbach Equation used to determine pCO2
Blood Gas: pH
- Measured using Sanz Electrode
- 2 half-cells connected by KCl bridge
- Measurement half-cell contain glass membrane with layers of hydrated and non-hydrated glass, permeable or sensitive to H+
- Measurement electrode consists of Silver-Silver chloride bathed in phosphate buffer at known pH 6.84
- Reference half cell contains mercury-mercury chloride in KCl saturated solution
- Measuring electrode responds to the H+ in the sample and the difference between reference and measurement cells measured by a voltmeter or pH meter
- At 37 degrees – change in one pH unit alters voltage by 61.5mV
Blood Gas: Bicarbonate
- Calculated from pH and CO2 using Henderson-Hasselbach Equation
- Actual bicarbonate
- Aerobically drawn arterial sample
- Standard bicarbonate
- Bicarbonate level in an oxygenated plasma specimen at 37 degrees and a pCO2 of 40mmHg
Sakurai 2016
Examiner Comments
2022B 16: 36% of candidates passed this question.
This question about how PaO2, PaCO2, pH, and HCO3 are obtained was not well answered by most candidates. Arterial blood gasses are routinely performed in most ICU on a daily basis. This question relates to a Level 1 (L1) topic in the CICM First Part Syllabus. Most answers simply lacked enough information. Details of how the Clark, Severinghaus, and Sanz electrode’s function was expected. Many candidates confused the pH and PaCO2 electrodes and confused the Clark (Polarographic) electrode with a Fuel Cell. Some knowledge about the types of electrodes and chemical reactions (e.g. reduction of O2 at the Platinum cathode in the Clark electrode) occurring in these devices was expected.
17. Describe the site of action, mechanism of action and the biochemical effects of five different classes of diuretics; include an example in each class.
CICMWrecks Answer
Sites and Mechanisms of actions of diuretics
Class | Drug | Sites | Mechanism of Action | Biochemical effects |
---|---|---|---|---|
Carbonic Anhydrase Inhibitor | Acetazolamide | PCT | Prevents the intracellular conversion of CO2 and H2O to H2CO3 → Resulting HCO3 usually reabsorbed via Na/HCO3 co transporter therefore decreased HCO3 leads to decreased Na reabsorption → decreased reabsorption of water | Metabolic acidosis Hyponatraemia Hypokalemia Hyperchloremia |
Loop | Frusemide | LoH | Inhibits Na/K/2Cl transporter on luminal membrane preventing ion reabsorption → increases osmolality of luminal fluid → decreased osmotic gradient for water reabsorption | Hypochloraemic metabolic alkalosis Hypernatraemia Hypokalaemia Hypomagnesaemia Hypophosphatemia Acidification of urine |
Thiazide | Hydrochlorothiazide | DCT | Inhibits Na/Cl transporter on the luminal membrane preventing Na and Cl reabsorption → increases osmolality in luminal fluid → decreased osmotic gradient for water reabsorption | Hypochloraemic metabolic alkalosis Hyponatraemia Hypokalaemia Dyslipidaemia Hyperglycaemia |
Aldosterone Antagonist | Spironolactone | CD | Prevents activity of aldosterone → Decreased ENaC channels for Na reabsorption in the collecting duct and decreased Na/K ATPase activity in collecting duct → Prevents Na reabsorption and consequently reduced osmosis | Hyponatremia Hyperkalaemia Metabolic acidosis |
Osmotic | Mannitol | Other | Increases osmolality of luminal fluid → decreases osmotic gradient for H2O reabsorption | Hypokalemia Hyponatremia Hypocalcemia |
CICMWrecks 2019
Further Reading:
Examiner Comments
2022B 17: 90% of candidates passed this question.
This question was best structured using the headings supplied in the stem (site, mechanism of action, biochemical effects and an example). Some answers utilised a table format which often meant that the content was squashed. Given the amount of information required a better format was simply to use separate sections for each class. Non-biochemical effects did not attract any marks. Similarly, an introductory statement about diuretics was not required and attracted no marks. Biochemical effects were not answered clearly or completely. Effect on acid-base was answered correctly by very few candidates. Vague statements such as “increased sodium” did not score as well as more precise terminology like “hypernatraemia” or “raised serum sodium”. A few answers incorrectly stated that the mechanism of action of thiazides was ENaC inhibition.
18. Describe the generation of ATP by mitochondria (50% marks) and outline the processes by which ATP is generated in red blood cells (50% marks).
CICMWrecks Answer
ATP Generation by mitochondia
- Mitochondria form ATP via oxidative phosphorylation (Major), via Kreb’s cycle and Electron transport chain (ETC)
- mitochon found in high conc in cells with high metabolic demands eg myocardium (23% of cell), brown fat (neonate)
- exercise ↑s numbers
- OP = production of ATP associated with oxidation by the flavoprotein cytochrome system in mitochondria
- Structure
- The inner and outer membranes of mitochondria define three compartments within the organelle, each with its distinct role and corresponding protein components.
- Outer membrane separates mitochondria from cytoplasm
- The innermost compartment, surrounded by the inner membrane, is the mitochondrial matrix
- The inner membrane of the mitochondrion contains the components of the electron transport chain.
- The high pH of the mitochondrial matrix creates the trans-membrane electrochemical gradient that drives ATP synthesis
- Oxidation/reduction reactions along the components of the electron transport chain generate a proton gradient that is used by ATP synthase to phosphorylate ADP, thereby producing ATP.
- To increase the capacity of the mitochondrion to synthesize ATP, the inner membrane is folded to form cristae. These folds allow a much greater amount of electron transport chain enzymes and ATP synthase to be packed into the mitochondrion.
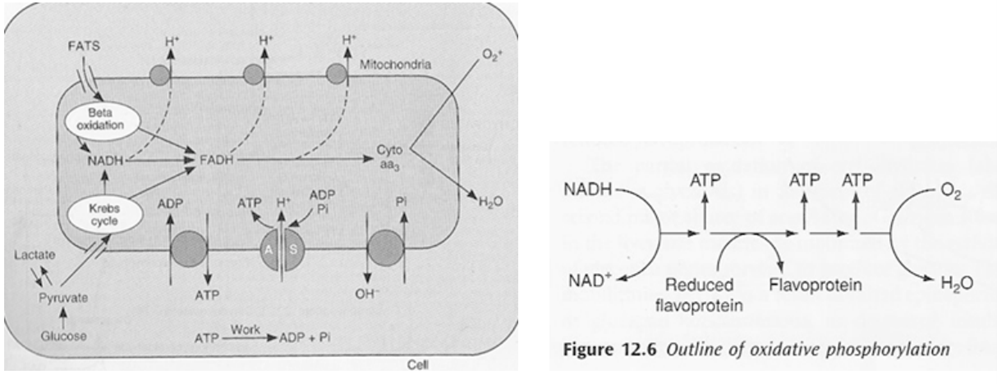
- Kreb’s cycle (TCA or citric acid cycle)
- Carbohydrate / Protein / Lipid ⇒ Acetyl coenzyme A or other intermediates
- Acetyl CoA ⇒ Kreb’s cycle
- Electron Transport Chain (ETC)
- The metabolic pathway through which the electron passes, starting with one transporter and then onto the next, is known as the electron transport framework (ETS).
- The electron transport framework happens in the inward mitochondrial layer.
- The electron transport chain contains the accompanying:
- Complex I: NADH dehydrogenase
- Complex II: succinate dehydrogenase
- Complex III: cytochromes bc 1
- Complex IV: cytochromes a-a3
- Complex V: ATP synthase
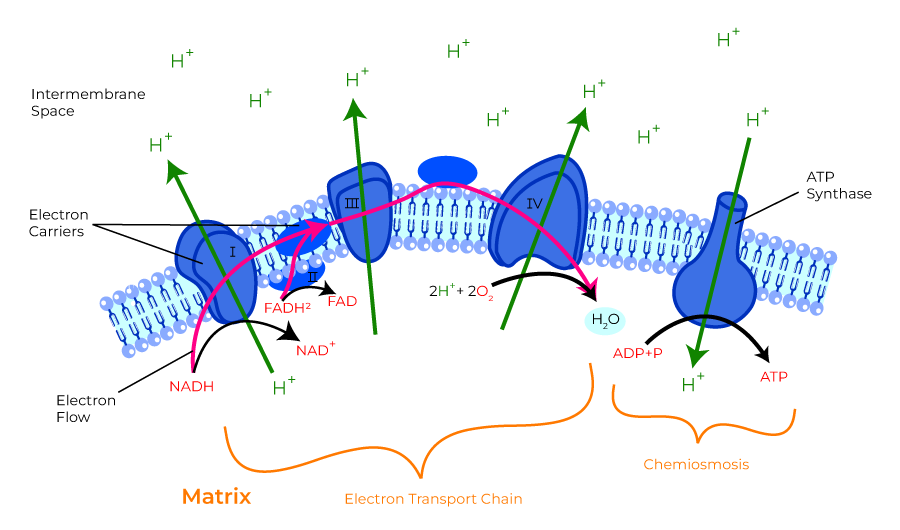
- ATP formed in electron transfer chain:
- Substrate diffuses into mitochon cytoplasm
- Hydrogen removed by a dehydrogenase
- NAD carries hydrogen to respiratory chain
- Hydrogen ionises and protons pass along series of carrier molecules across insulating membrane (inner membrane of mitochondria – forms cristae)
- Movement of protons creates an electrochemical gradient for transport of protons from intermediate space back into matrix ⇒ this drives a reversible ATPase in inner membrane (ATP synthase)
- ATP synthase: ADP + Pi ⇒ ATP
- @end:
- ATP produced
- Reduction of O2 to water – catalysed by cytochrome oxidase
- cyanide inhibits this oxidase ∴ inhibits OP in mitochon
- O2 required to oxidise NADH
- Eg’s of carrier molecules in electron transfer chain
- Flavoprotein
- Cytochromes A, A3, B, C, C1
- Ubiquinone
- Several iron sulphide proteins
- OP depends on:
- Adequate supply of ADP: +ve feedback loop e.g. ↑ATP utilisation ⇒ ↑ADP ⇒ ↑OP
- Rate of delivery of fats, lactate, glucose to interior of mitochon
- Availability of O2: Pasteur point = 1-2mmHg i.e. point below which OP cannot occur
- ∴ cardioresp works in harmony to ensure o2 reaches cells
- defined by oxygen flux equation:
ATP Generation by RBCs
- RBC have no Mitochondia – Cannot perform aerobic metabolism
- All ATP is generated via anaerobic glycolysis (Embden-Meyerhof pathway / EMP) (90% glycolysis)
- 10-step Catabolic pathway in cytosol
- by degradation of glucose molecule
- produces 2x ATP + pyruvate/lactate per glucose → ATP is used by Na+/K+ATPase, which is implicated in maintaining RBC shape, volume and flexibility
- Steps
Step | Substrate | Enzyme | Product | ATP |
---|---|---|---|---|
1 | Glucose | hexokinase | Glucose-6-phosphate | ATP consumed |
2 | Glucose-6-phosphate | glucose phosphate isomerase | Fructose-6-phosphate | |
3 | Fructose-6-phosphate | phosphofructo kinase | fructose-1,6-diphosphate | ATP consumed |
4 | fructose-1,6-diphosphate | aldolase | 2x glyceraldehyde-3-phosphate | |
5 | 2x glyceraldehyde-3-phosphate | glyceraldehyde-3-phosphate dehydrogenase | 2x 1,3-bisphosphoglycerate | |
6 | 2x 1,3-bisphosphoglycerate | phosphoglycerate kinase | 2x 3- phosphoglycerate | 2x ATP produced |
7 | 2x 3- phosphoglycerate | 3-phosphoglycerate mutase | 2x 2-phosphogylcerate | |
8 | 2x 2-phosphogylcerate | enolase | 2x phosphoenolpyruvate | |
9 | 2x phosphoenolpyruvate | pyruvate kinase | 2x pyruvate | 2x ATP produced |
10 | pyruvate | lactate dehydrogenase | lactate |
- 3 shunts that come off the anaerobic glycolytic pathway (produce no ATP)
- the Rapoport-Luebering shunt (BPG Shunt): 2,3-DPG is interconverted from 1,3-DPG (glycolytic intermediate)
- Hexose monophosphate shunt (HMP shunt or pentose phosphate pathway) – generates NADPH – protects RBC from oxidative damage (10% glycosis)
- NADH generated – used by MetHb reductase to reduce oxidised Hb (MetHb) to Hb
Examiner Comments
2022B 18: 15% of candidates passed this question.
Excellent answers focused on oxidative phosphorylation and the chemiosmotic mechanism in their description of ATP production by mitochondria. This required a description of the structure of the mitochondrial components involved in ATP production, the establishment of an electrochemical gradient of protons across the inner membrane, how the electron transport chain works and its components, and the roles of cytochrome oxidase and ATP synthase. Excellent answers describing ATP generation in red blood cells focused on anaerobic glycolysis in the absence of mitochondria, with an emphasis on the key steps that consume or produce ATP. The role of lactate dehydrogenase in regenerating NAD+ was also emphasised. Some candidates described other pathways of metabolism that do not generate ATP, for which no marks were awarded.
19. What are receptors? (20% marks). Discuss the relationship between the properties of a drug and potential receptor response under the following headings: agonists, partial agonists, inverse agonists and antagonists (80% marks)
CICMWrecks Answer
Receptors
- Receptor → protein-based moiety that contains a region that binds a natural ligand (or drug) to bring about a response
- They are located either:
- Within the cell membrane → ligand is poorly lipid-soluble (Ie. cannot cross cell
membrane) - Intracellularly → ligand is lipid-soluble (Ie. can cross cell membrane)
- Within the cell membrane → ligand is poorly lipid-soluble (Ie. cannot cross cell
- Drug-receptor binding can invoke 3 responses:
- Elicits an effect → agonist response
- Prevent the action of a natural ligand → antagonist response
- Reduce a constitutive effect of a receptor → inverse agonist response
- There are 5 classes of receptors:
- Ligand-gated ion channels
- G-protein coupled receptors (GPCR)
- Membrane guanylyl cyclase
- Tyrosine kinase receptors
- Intracellular receptors
Receptor | Structure / Mechanism | Class | Action | Example | ||
---|---|---|---|---|---|---|
Ion channel receptors (ionotropic) | Ligand-gated | – part of membrane-spanning complex of protein subunits that can form a channel through the membrane – generally found in excitable tissue | Ligand (or drug) binds to receptor to regulate ion flow through channel | Pentameric family | Possess 5x membrane-spanning units | nAChR – Na GABA-A receptor – Cl 5-HT3R glycine receptor |
Ionotropic glutamate | Glutamate is an excitatory NT in CNS that acts on various ligand-gated ion channels | NMDA receptor – Ca2+ AMPA, Kainate receptors – Na / K | ||||
Ionotropic purinergic receptors | ATP acts on PX1/PX2 receptors → involved in pain and mechano-sensation | PX1/PX2 receptors – Na,K,Ca – mechanosensation and pain | ||||
Voltage-gated | Have a common 4-subunit structure (each with 6 transmembrane segments) surrounding a central pore This pore is selective for the particular ion | Undergo a conformational change when the threshold potential is reached This is sensed by the S4 helix, which acts to open and close the channel | Na+ | Important in generating and transmitting an action potential by permitting sodium influx into cells | Located in myocytes and neurons | |
Ca+ | L | Muscular contraction | ||||
T | Cardiac pacemaker | |||||
N/P/Q | Neurotransmitter release | |||||
K+ | Located in myocytes and important in repolarisation following an action potential. | |||||
GPCR (metobotropic) | – Membrane bound protein with serpentine structure – associated with a heteromeric G-protein (α, β, γ subunits – α subunits binds either GTP or GDP) on the intracellular aspect of cell membrane | Ligand (or drug) binds to GPCR extracellularly → induces conformational change that activates G-protein, which triggers a cascade of intracellular signalling mechanisms – Inactive: GDP bound to α subunit, which is associated with a βγ-dimer – Active: G-protein activated by ligand-bound GPRC – GTP replaces GDP → activates or inhibits effortor protein: adenyl cyclase (AC) / phospholipase C (PLC) / ion channel – Intrinsic GTPase in α subunit hydrolyses GTP to GDP → regenerates an α-GDP subunit which reassociates with βγdimer → inactive | Gs | α-subunit activates AC → ↑ cAMP synthesis → cAMP binds protein kinase A (PKA) to produce cellular effect (Ie. ∆ gene transcription, ∆ ion permeability of membrane) | β1, β2, β3 receptors H2 receptor | |
Gi | α-subunit inhibits AC → ↓ cAMP synthesis → ↓ PKA activation → cellular effect | α2 receptor M2 and M4 receptors | ||||
Gq | α-subunit activates PLC → cleaves membrane phospholipid (phosphatidylinositol biphosphate; PIP2) into: – Inositol tri-phosphate (IP3) → causes Ca2+ release from ER – Diacylglycerol (DAG) → activates protein kinase C | α1 receptor M1, M3 and M5 receptors H1 receptor | ||||
Enzyme-linked receptors (Catalytic) | MONOMER: Membrane guanylyl cyclase | activated by a ligand and cause enzymatic activity on the intracellular side | Membrane-bound receptors a/w an intrinsic guanylyl cyclase | Ligand or drug binds to receptor → activates intrinsic guanylyl cyclase → ↑ cGMP → phosphorylation of IC enzymes → cellular effects | guanylyl cyclase (ANF receptor) Guanylyl cyclase for NA | |
DIMER: Tyrosine kinase receptors | activated by a ligand and cause enzymatic activity on the intracellular side | Transmembrane receptor-enzyme complex that consists of – extracellular ligand-binding domain (2x α-subunits) and – membrane-bound domain (2x β-subunits) associated with a cytoplasmic enzyme (Tyrosine kinase) | Ligand or drug binds to the α-subunits (extracellular domains) which causes the β-subunits (membrane-bound domains) to dimerise → IC tyrosine residues on β-subunits are then phosphorylated leading to activation of tyrosine kinase → this phosphorylates various IC proteins that elicits a cellular effect | Fibroblast GF receptor Insulin receptor | ||
Intracellular receptors | act as “ligand-regulated transcription factors”: – receptors reside within the cytoplasm → held in the inactive form by association with inhibitory proteins – Binding of hormone (or drug) induces conformational change that activates receptor (by releasing inhibitory protein) → receptor-ligand complex translocates to nucleus and associates with DNA promoter sequences → alter gene transcription | Ligands acting on these receptors are lipid soluble → cross cell membrane and bind to receptors within the cytoplasm | Steroid Receptor Superfamily which include steroids, sterols, thyroxine, retinoic acid and vitamin D. |
Drug – Receptor Response
Agonist:
Defined as a ligand or drug that binds to a receptor and alters the receptor state resulting in a biological response
Antagonist:
Defined as a ligand that binds to a receptor but does not activate it, instead blocking that receptor to a natural agonist
Efficacy:
- Defined as the ability of drug to elicit the maximal effect (EMAX) when bound to receptor
- Measured by height of plateau phase (or EMAX) in “log dose-response curve” → ↑ height of plateau phase (or EMAX) = ↑ efficacy
- It reflects “intrinsic activity” of the drug (Ie. magnitude of effect drug has once bound)
- Full agonists → 100% efficacy (or IA = 1)
- Partial agonists → efficacy b/t 0 and 100% (or 0 < IA < 1)
- Antagonists → 0% efficacy (or IA = 0)
- It is vital when selecting drugs (Ie. paracetamol and morphine are both analgesics but with different efficacy)
Potency:
- A comparative measure b/t drugs that have the same action on a receptor (Ie. have same log dose-response curve slopes) → refers to the different doses of two drugs needed to produce the same drug effect (Ie. more potent drug evokes a ↑ response at a ↓ dose)
- Measured by the EC50 in “log dose-response curve” → ↑ EC50 = ↓ potency
- It reflects the “affinity” of the drug for the receptor → ↑ receptor affinity = ↓ KD or ↑ KA = ↑ potency
- It is not as vital when selecting drugs (cf. efficacy), as long as the effective dose can be administered conveniently
Pharmacological effect of drug-receptor binding depends on:
- Properties of the drug:
- Affinity (Ie. how avidly a drug binds to a receptor)
→ determined by KA or KD of drug (such that ↑ KA or ↓ KD affinity - Intrinsic activity (IA; Ie. magnitude of effect drug has once bound)
→ drugs have IA b/t 0 and 1 (Nb. inverse agonists have IA b/t -1 and 0)
- Affinity (Ie. how avidly a drug binds to a receptor)
- State of receptor activation:
- Receptors exist in an equilibrium b/t “active form” and “inactive form”, which is altered by the presence of a drug
Receptor Affinity | Intrinsic Activity | State of receptor activation | Example | |
---|---|---|---|---|
Abscence of Drug | Equilibrium favours most receptors being in inactive form | |||
Full Agonist | High | Full (IA=1) | Drug binds receptors → shifts equilibrium towards ALL receptors being in “active form” | phenylephrine or noradrenaline at α1 receptor |
Partial Agonist | High | Fractional (0<IA<1) | Drug binds receptors → shifts equilibrium towards a FRACTION of receptors being in “active form” | buprenorphine at μ receptor |
Inverse Agonist | High | -ve (full or fractional) (-1=<IA<0) | Drug binds receptors → shifts equilibrium towards ALL or FRACTION of receptors being in “inactive form” | naloxone at μ receptor |
Antagonist | High | no (IA=0) | Drug binds to both receptor forms but does not alter equilibrium b/t “active” and “inactive” | Reversible, competitive: NDMR at nAChR Reversible, non-competitive: ketamine at NMDA receptor Irreversible: phenoxybenzamine at α receptor |
Examiner Comments
2022B 19: 31% of candidates passed this question.
The description of a receptor was worth 20% thus it was expected that detailed information on the different forms of receptors, their structure, the resultant conformational change when activated and where they are found would be provided for full marks. Most candidates were able to correctly define an agonist, antagonist, partial agonist and inverse agonist. Unfortunately, this was the limit of most answers. Candidates were expected to provide details of drug or agonist/receptor interaction discussing the terms affinity/intrinsic activity and how different mechanisms of binding and interacting with the receptor alters these terms.
20. Describe the pharmacokinetics of prednisone (50% marks). List the cardiovascular, renal, metabolic, haematological and immunological effects of prednisone (50% marks)
CICMWrecks Answer
Prednisolone
Pharmacokinetics
- Absorption:
- PO and Rectal
- Rapidly and completely absorbed by both routes – 80–100%.
- Distribution:
- reversibly bound to albumin and a specific corticosteroid-binding globulin
- Protein binding: 80–90% (at low conc), 60-70% (at higher conc.)
- VD: 0.35-0.7 L/kg
- Metabolism:
- liver – hydroxylation with subsequent conjugation.
- Excretion:
- 11–14% unchanged in the urine
- Dose-dependent clearance: 170 to 200 ml/min
- Elimination half-life: 2.6–5 hours
Effects
- CVS
- positive effect on myocardial contractility
- causes vasoconstriction by increasing the number of alpha-1adrenoreceptors and beta-adrenoreceptors and stimulating their function.
- In the absence of corticosteroids, vascular permeability increases; small blood vessels demonstrate an inadequate motor response, and the cardiac output decreases.
- Renal
- weak mineralocorticoid effects and produces sodium retention and increased potassium excretion
- urinary excretion of calcium is also increased
- increases the glomerular filtration rate and stimulates tubular secretory activity.
- Metabolic
- profound effects on carbohydrate, protein, and lipid metabolism.
- stimulates gluconeogenesis and inhibits the peripheral utilization of glucose
- cause a redistribution of body fat, enhance lipolysis, and also reduce the conversion of amino acids to protein.
- Haematological
- increases red blood cell, neutrophil, and haemoglobin concentrations
- depresses other white cell lines and the activity of lymphoid tissue.
- Immunological
- potent anti-inflammatory agent which inhibits all stages of the inflammatory process
- inhibits neutrophil and macrophage recruitment, blocking the effect of lymphokines
- inhibits the formation of plasminogen activator.
- CNS
- increases the excitability of the CNS
- absence of glucocorticoid leads to apathy, depression, and irritability.
- GIT
- increases the likelihood of peptic ulcer disease
- decreases the gastrointestinal absorption of calcium.
Source: Drugs in Anaesthesia and Intensive care 5th Edition
Examiner Comments
2022B 20: 40% of candidates passed this question.
The question asked for detailed information on the pharmacokinetics and selected pharmacodynamics of prednisone. Most candidates provided unnecessary details of the mechanism of action and pharmaceutics significantly wasting time. Many provided incorrect information and general information without specifics which is represented by the low pass rate. The pharmacokinetics part was answered poorly. It was expected from the candidates to have detailed knowledge on the prednisolone binding capacity and affinity to albumin and corticosteroid binding protein as well as the important metabolism of prednisone.
VIVAs
A. Pharmaceutics | |
B. Pharmacokinetics | |
C. Pharmacodynamics | |
D. Variability in Drug Response | |
E. Cellular Physiology | Plasma proteins, concentrations |
F. Respiratory | Regional variability in V&Q throughout upright lung Oxygen cascade Work of breathing and components, diagram |
G. CVS | Idealized LV P-V loop LV P-V loop – Mechanical events Cardiovascular function curves |
H. Renal | Renal blood flow, regulation Renal vasculature, explain divisions beyond arcuate artery |
I. Body Fluids and Electrolytes | |
J. Acid Base | ABG Interpretation (pH 7,3 pCO2 19 pO2 79 HCO3 9 BE -16.4 Na 133 K 4.8 Cl 104 Lactate 7.5, FiO2 30% Temp 37.5) ABG Interpretation (pH 7.3 pO2 400 pCO2 30 HCO3 14 BE -8 Na 140 K 4 Cl 120) |
K. Neuro | Consequence of unexpected cut to hand Cerebral blood flow: relation with PaCO2, PaO2, CPP P-V relationship for ICP Pain, detection and response, Analgesics GABA receptors, Anticonvulsants |
L. Musculoskeletal | Motor unit, skeletal contraction and relaxation |
M. ANS | |
N. Liver | Liver lobule |
O. GIT | |
P. Nutrition and Metabolism | Carbohydrate metabolism, factors that increase insulin secretion Carbohydrate metabolism, factors that increase insulin secretion |
Q. Haematology | Viscoelastic assay, parts of curve Red cell production, storage and transfusion |
R. Thermoregulation | Temperature, measurement |
S. Immunology | |
T. Microbiology | |
U. Endocrine | |
V. Obstetrics | Identify curves which belong to major vessels involved in placental gas transfer |
W. Measurement and Monitoring | |
X. Procedures |
Recent Comments