SAQs
1. (a) Describe the work of breathing and its components (85% of marks).
(b) Briefly outline the efficiency of the lung (15% of marks).
CICMWrecks Answer
Work of Breathing
- WOB = Energy used by the respiratory muscles during ventilation
- Work = Pressure x Volume (measured in Joules)
- represented as area on pressure-vol loop (dynamic compliance curve)
- Tidal breathing at rest: <2% BMR; O2 requirement = 3ml/min
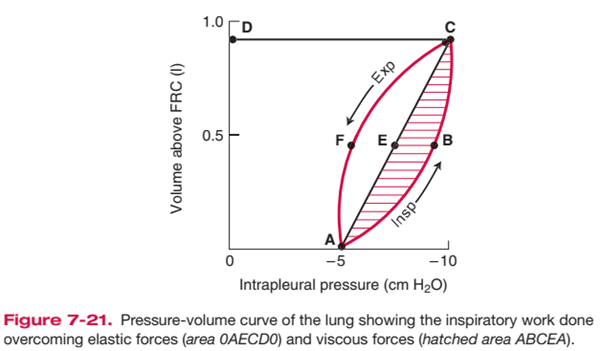
Components of Work of Breathing
WOB can be divided into:
- Inspiratory work
- Active
- Elastic + resistive work
- Expiratory work
- Usually passive (uses potential energy stored during inspiration)
- Resistive work
Inspiratory elastic work
- ~65% of total work
- work done on inspiration to overcome:
- surface tension of lung (70%)
- elastic properties of lung/ lung elastic recoil (30%)
- stored as elastic potential energy → used on expiration
Inspiratory + expiratory resistive work
- ~35% of total work; lost as heat
- energy required to overcome frictional forces: includes
- Airway resistance: between gas molecules
- depends on type of flow + airway calibre
- turbulent flow (↑RR; upper airway obstruction, ↑airway density)
→ ↑ airway resistance than laminar flow - ↓ airway calibre (radius) (e.g. bronchoconstriction, dynamic airway compression, ETT)
→ ↓ calibre → ↑ WOB
- Tissue resistance e.g. interstitial lung disease
- Airway resistance: between gas molecules
- Usually potential energy stored from insp work is sufficient to perform expiratory resistive work → expiration can become active in pathological conditions e.g. COPD, asthma
Total work and efficiency of breathing
- O2 cost of breathing:
- During a normal TV breath at rest, the total work of breathing is low – The
inspiratory muscles consumes 3 mL O2/min, which is ~1% of the body’s total
O2 consumption at rest (VO2 = 250 mL O2/min) - O2 consumption of breathing increases by up to 30% with hyperventilation (due to an increase in either minute volume or RR)
- During a normal TV breath at rest, the total work of breathing is low – The
- Total work and efficiency of breathing:
- Difficult to measure – It is calculated by measuring O2 cost of breathing, then
assuming efficiency as:
- Difficult to measure – It is calculated by measuring O2 cost of breathing, then
Normal efficiency is estimated at 5-10%
Increased WoB
- Elastic Work:
- Breathing large lung volumes: increases total work of inspiration by extending the line AEC to a higher volume above FRC, increasing area 0AECD0
- Poorly compliant lungs (e.g. restrictive disease surfactant deficiency): increases total work of inspiration by reducing slope of AEC, increasing area 0AECD0
- Resistive Work
- e.g. turbulent flow with rapid RR, lung disease associated with increased AWR
- Increases total work of inspiration by producing a larger area ABCEA (i.e. it bulges)
- This also causes AECFA to bulge out and increase area, such that when stored elastic potential is insufficient to overcome this tissue resistance force, expiration becomes an active process requiring work as expiratory muscles are recruited
Minimizing WoB
- Elastic work:
- PEEP: keep lung vol at FRC and maximise number of ventilated alveoli
- Positioning: optimise lung volume
- Surfactant: minimise surface tension
- Optimise RR: elastic WOB ↓s with ↑ RR
- Resistive work
- ↓RR: RR directly proportional to resistive work
- ↑laminar flow: more efficient than turbulent flow; can be ↑ by ↓gas density e.g. heliox
- ↑radius: ↑lung vol; bronchodilators
Kerr / Bianca
Examiner Comments
2024B 01: 29% of candidates passed this question.
This question required candidates to provide a detailed description of the work of breathing and its components. A good answer divided the components into elastic and inelastic/resistive work and provided an overview of the factors that affected each of them. This description was allocated the majority of marks. A thorough answer also discussed the role of expiration on work of breathing, whilst usually a passive process it can be active under some conditions and good answers touched upon these. The second section of the question required an overview of the fate of the energy utilised by the respiratory muscles, with a large proportion lost as heat, or through hysteresis and airway resistance.
2. Describe the regulation of systemic vascular resistance (SVR).
CICMWrecks Answer
Systemic Vascular Resistance
(Total Peripheral Resistance)
Systemic vascular resistance (peripheral vascular resistance, SVR) is the resistance in the circulatory system that is used to create blood pressure, the flow of blood and is also a component of cardiac function.
- Majority of resistance in systemic system results from arterioles – state of contraction and relaxation of smooth muscle cells of arterioles which determines distribution of blood to organs
- The % of each organ blood flow is dependent on the organ vascular resistance
- Systemic vascular resistance is the resistance of several circuits in parallel, which have both common and independent factors in their regulation.
- As they are in parallel the sum of reciprocals is used to determine the overall value.
Control
- As per Hagen Poiseuille Equation:
where: η = blood viscosity
L = length of vessel
r = radius of vessel
- radius is the most important factor
Extrinsic Control
- Extrinsic SNS control
- Arterioles have profuse SNS supply → NAd from nerve endings act on α1-adrenoceptors to cause vasoconstriction (and β2-adrenoceptors to cause vasodilation – but effect is weaker!)
- Due to the tonic SNS outflow from medullary vasomotor centres, arterioles have a basal level of vasoconstriction → the degree of basal vasoconstriction (and arteriolar resistance) can be varied by altering SNS outflow
- Extrinsic PNS control
- PNS control of arterioles is less important → vessels of external genitalia have dual ANS supply with PNS dilator nerves and SNS constrictor supply; PNS activation in heart, brain and lungs have an uncertain role
- Extrinsic hormonal control
- Adrenaline (from adrenal medulla) → effect on organ blood flow depends on the relative % of α1- and β2-adrenoceptors present in the arteriole
- AII (from RAAS) → vasoconstriction (via AT2R)
- ADH (from posterior pituitary) → vasoconstriction (via V1R)
- ANP (from RA) → vasodilation (via ANPR)
Intrinsic Control
- Autoregulation
- Ability of an organ to maintain relatively constant blood flow across variations in perfusion pressure
- flow = pressure/ resistance → as the P changes, the R also changes to maintain flow
- Outside limits of autoregulation: flow = dependent on driving pressure
- Kidneys, brain, heart
- Autoregulation is dependent on 2 mechanisms:
- Pressure autoregulation: myogenic stretch response to ↑ and ↓ in pressure → vasoconstriction, and vasodilation
- Metabolic or vasoactive autoregulation: direct action of locally derived metabolites and vasoactive substances e.g. platelets release thromboxane A2 → constriction in damage
Bianca / Kerr 2016
Examiner Comments
2024B 02: 38% of candidates passed this question.
This question integrated knowledge from different sections of cardiovascular physiology. High scoring answers gave a definition of SVR, site of highest resistance, equation and correct units. This was then followed by a structured answer that included descriptions of: inputs, both peripheral and central; the central integrator (vasomotor centre) and controllers such as neural output, hormones and local factors. Inputs included carotid and cardiopulmonary baroreceptors, peripheral and central chemoreceptors and other factors such as visceral distention. Neural outputs included the autonomic nervous system and their contribution to basal tone. Hormones included angiotensin II, Vasopressin, ANP and progesterone Local regulation included myogenic, metabolic, enothelin and endothelium derived hyperpolarisation factors. Given the significant breadth of this question it was expected that each of these factors required only a brief explanation of how they contribute to SVR and the conditions under which they operate.
3. (a) Describe the factors that determine the glomerular filtration rate (GFR) in the kidney (70% of marks). (b) Outline the methods by which GFR can be measured (30% of marks).
CICMWrecks Answer – GFR, Determinants, Factors
GFR
GFR:
- “The amount of glomerular ultrafiltrate formed divided by the time of filtration”
- Normal value is approx 125 mL/min or 180 L/day
- Renal blood flow 1.25l/min in 70kg male → Filtration fraction 0.2
Functional anatomy:
3 Distinct Layers:
- glomerular capillary endothelium
- highly specialised endothelium with fenestrations to ↓ filter thickness
- prevents cellular components of blood from coming into contact with BM
- glomerular BM
- made of CT; -vely charged
- acts as filter
- bowmans epithelial cells (podocytes)
- epithelial cells with foot processes → large SA
- negatively charged
- maintain BM + phagocytic functions
Direct GFR Determinants
The GFR (Net flux across the membrane) is the balance of hydrostatic pressure and oncotic pressure, as defined by the Classic Starling Equation.
where
Kf = Filtration coefficient
P = hydrostatic pressure
π = oncotic pressure
σ = Staverman’s reflection coefficient ie. Permeability of membrane to protein
- Normally NFP = 17mmHg
- Tubular oncotic pressure = zero throughout
- GC oncotic pressure varies from 21 to 33 mmHg as filtrate is removed.
- Thus less GFR produced at distal end of tubule.
Afferent end of Glomerular Capillary (mmHg) | Efferent end of Glomerular Capillary (mmHg) | |
---|---|---|
PGC | 60 | 58 |
PT | 15 | 15 |
πGC | 21 | 33 |
NFP | 24 | 10 |
Kf | Filtration coefficient | = LpS = Hydraulic conductivity x Surface Area Glomerular surface area = 0.8m2 • Altered by Mesangial cell contraction (see circulating factors e.g. Angiotensin II → ↓SA → ↓GFR) Patency of the normal capillary wall structure (ie in tubular dysfunction Kf ↑’s ↑GFR) |
PGC | hydrostatic pressure in capillary | relates to • RBF which is autoregulated for MAP 70-170mmHg • relative afferent/efferent arteriolar tone Affected by • Catecholamines • Local autoregulation -> Myogenic -> Tubuloglomerular feedback -> Hormones (see below) |
PT | hydrostatic pressure in tubule | relates to • obstruction to urinary flow (usually pathological) • ↑PT → ↓ GFR (ie post renal obstructiion causing renal failure) |
πGC | oncotic pressure in capillary | relates to • plasma protein concentration (incr in dehydration, decreased in heart failure) • ↑Systemic plasma oncotic pressure → ↑πGC → ↓GFR • ↓Renal plasma flow → ↑πGC → ↓GFR |
πT | oncotic pressure in tubule | • usually zero, but can increase in renal failure/proteinuria • ↑’d πT → ↑GFR |
σ | Staverman’s reflection coefficient | • Permeability of membrane to protein usually 1 (no protein leak) • can decrease with nephritis/proteinuria |
Factors influencing GFR
Circulating Factors
- Prostaglandins
- PGI2 and PGE2 → vasodilates → ↑ renal blood flow → ↑ GFR
- ↓ PGE2, PGI2 and NO levels → inhibits afferent arteriolar vasodilation → ↓ GFR
- Long term → ↓ renin release → ↓ ATII-induced efferent arteriolar vasoconstriction → ↓ GFR
- Noradrenaline / Adrenaline
- constrict renal afferent and efferent arterioles → ↓ renal blood flow and reducing filtration → ↓GFR
- Constrict mesangial cells to → ↓ GFR.
- Mesangial cell tone:
- Angiotensin 2, Endothelin, vasopressin → ↓ glomerular surface area and GFR
- ANP, PGE2, Dopamine and cAMP → all increase GFR
Solute factors
- Size
- < 7 kDa (Eg. glucose, ions, urea, H2O) filtered freely,
- > 70 kDa (Eg. albumin) are not filtered;
- neutral particles < 4 mm diameter filtered freely, > 8 mm are excluded
- Charge
- Negatively charge (Anionic) particles repulsed
- Cationic substances filtered more readily
- Protein binding
- Albumin excluded
Disease Factors
- Filtration decreases
- Shock → decreased glomerular pressure
- Obstruction → increased bowman’s capsule hydrostatic pressure
- Hypoproteinaemia → hepatic failure, nephrotic syndrome
JC / Gladwin / Sakurai / Kerr 2020
CICMWrecks Answer – GFR Measurement
GFR Measurement
Clearance:
- Renal clearance = vol of plasma completely cleared of a given substance by the kidneys per unit time (ml/min)
- Involves: glomerular filtration, secretion, reabsorption, and rarely tubular metabolism
- Renal clearance = V x [U]/[P]
- V = volume of urine or urine flow rate in ml/min
- [U] = urinary concentration of substance in mg/ml
- [P] = plasma concentration of substance in mg/ml
Estimating GFR:
- GFR = renal clearance of a substance if it is:
- Freely filtered at glomerulus
- Not secreted
- Not reabsorbed
- Not synthesized
- Not metabolised
- The amount excreted in the urine = amount filtered
- i.e. [plasma] x GFR = [urine] x urine vol
- Rearrange: GFR = urine vol x [urine] / [plasma]
Substances used to estimate GFR:
- Criteria
- easily measured in urine + plasma
- non-toxic
- easily administered
- Inulin
- Protein; polymer of fructose
- MW 5800 Da
- freely filtered
- Not reabsorbed, secreted, metabolised, or synthesized by the kidney
- Therefore clearance of inulin = GFR
- Requires continuous infusion for several hours to achieve steady state
- Creatinine
- used to approximate GFR as is more practical
- Released at a steady state from skeletal muscle cells (phosphocreatine)
- Freely filtered + not reabsorbed
- *Small amount secreted → overestimates GFR by small amount
- Cockcroft Gault formula used
JC / Gladwin / Sakurai / Kerr 2020
Examiner Comments
2024B 03: 44% of candidates passed this question.
The GFR equation provides a logical basis to structure an answer to the first part of this question. A discussion of the factors that affect each part of the equation provides a complete answer. For example, comprehensive answers used the following headings: glomerular hydrostatic pressure, Bowman’s capsule hydrostatic pressure, glomerular capillary oncotic pressure and the filtration coefficient providing information on what is responsible for each of these factors and how they may change therefore affecting GFR.
In the second section a strong answer provided a definition of clearance and description of the use of inulin versus creatinine, touching on advantages and disadvantages. This question asked for the methods of measurement of GFR thus methods used to estimate GFR such as the Cockcroft and Gault equation were not included.
4. Compare and contrast the anatomy and physiology of smooth and skeletal muscle.
CICMWrecks Answer
Anatomy
Skeletal Muscle | Smooth Muscle |
---|---|
Long, cylindrical, multi-nucleated cells | Spindle shaped single-nucleated cells |
10 μm diameter 100 μm long | 2 μm diameter 400 μm long |
Striated | Not striated |
Actin and Myosin arranged in sarcomeres | More actin than myosin Actin inserts into dense bodies and cell membrane |
Well developed sarcoplasmic reticulum and transverse tubules | Poorly developed sarcoplasmic reticulum No Transverse tubules |
Containes troponin in the thin filaments | Contains calmodulin → binds to Ca2+ → activates myosin light-chain kinase |
Ca2+ released from cytoplasm from sarcoplasmic reticulum | Ca2+ enters cytoplasm from extracellulr fluid, sarcoplasmic reticulum and mitochondria |
Cannot contract without nerve stimulation | Maintains tone in absence of nerve stimulation Visceral smooth muscle produces pacemaker potentials |
Denervation results in muscle atrophy | denervation results in hypersensitivity to stimulation |
Muscle fibers stimulated independently No gap junctions | Gap junctions usually present |
Two Types 1. Extrafusal (α-motor neuron supply) a) Slow-type (tonic) b) Fast-type (twitch) – Slow fatigue (type 1/red – slow oxidative) – Fast fatigue-resistant (type 2B/red – fast oxidative) – Fast fatiguable (type 2B/white) 2. Intrafusal (γ-motor neuron supply) – Non-contractile sensory fibers associated with muscle spindles | Two types 1. Visceral – located in hollow viscera – linked in sheets by gap junctions – contractile elements extend across multiple cells – Spontaneous depolarisation 2. Multiunit – located in eye (iris and ciliary body), large blood vessels, small airways, hair follicles – No gap junctions – Under ANS control without spontanaetiy |
Physiology
Skeletal Muscle | Smooth Muscle |
---|---|
Excitation | |
– Motor neuron depolarisation – ACh vesicular release – Multiple MEPs → End plate potential – Muscle AP propagates via T-tubles – Opens L-type Ca channels – Calcium induced calcium release from SR – Ca-troponin C interaction – Alteration and movement of Trop-tropomyocin complex – Exposure of Actin – Removal of Troponin I inhibition of myosin ATPase – Cross bridge cycling | – Motor neuron depolarisation – ACh vesicular release – Multiple MEPs → End plate potential – Muscle AP propagates – Opens L-type Ca channels |
Contraction | |
Sliding filament cross bridge cycling – “Flexed” Myosin without ATP binds to Actin. – Binding of ATP to Myosin causes release of Actin/Myosin complex and extension of the head – In the presence of Ca, Actin binding sites are open and binding of myosin/Actin/ATP complex is formed – Hydrolysis of ATP to ADP + P in myosin head causes a conformational change “initial flexing” the myosin head and releasing phosphate – Release of ADP further flexes Myosin head – In presence of Ca return to step 2. | – Influx of EC Ca → ↑ IC [Ca] – Ca binds Calmodulin → Ca-Calmodulin complex – Ca-Calmod → ↑ MLCK activity → – Phosphorylation of Myosin Light chain (MLC) → Activation of Myosin ATPase – Normal Cross-bridge cycling as per Skeletal muscle cross bridge cycling – Latch-bridging (Sustained SM contraction with minimal O₂ or ATP use) ◦ ↓ IC [Ca] + dephosphorylation of MLC does not cause unbinding of actin/myosin ◦ Unbinding occurs slowly |
Relaxation | |
– AChE metabolises ACh in the NMJ ◦ ↓ nAChR activation at MEP → ↓ EPP and muscle AP generation – SR actively sequesters Ca (via Ca-Mg ATPase) ◦ ↓ IC [Ca] → Ca removed from troponin C ◦ tropomyosin mediated inhibition of actin-myosin – Titin ◦ Elastic component of sarcomere → ↓ to normal length | – Ca removed from cell ◦ Ca ATPase ◦ Ca2+/Na+ antiport – Myosin Light Chain Phosphatase ◦ dephosphorylates MLC → inhibition of myosin ATPase |
Gladwin / JC 2020
Examiner Comments
2024B 04: 53% of candidates passed this question.
The anatomy component of this question required a detailed description of both the macro and microstructure of skeletal and smooth muscle, noting the differences and similarities between them. For skeletal muscle specific detail on the fibre arrangement, subtypes (I/IIA/IIB) and their innervation as well as the structure of the sarcomere, thick and thin bands and associated proteins was required. It was then expected that candidates would provide a description of how these components are similar or different in smooth muscle including details of unitary and multi-unit smooth muscle arrangements.When comparing the physiology of skeletal and smooth muscle, better candidates discussed not only the process of AP generation, propagation and stimulus, but also detailed sources of ATP, and highlighted the differences in requirements for energy of each muscle type. Higher scoring candidates discussed specific membrane potentials, noting the unstable membrane potential of smooth muscle which does not exhibit a resting membrane potential.
5. Describe the exocrine functions of the pancreas, including their function and regulation.
CICMWrecks Answer
Exocrine pancreas
- produces 1.5L/day pancreatic juice
- Pancreatic juice – digestive enzymes and bicarbonate
- Enzymes: synthesized and secreted from the exocrine acinar cells
- Bicarbonate: secreted from epithelial cells lining small pancreatic ducts
Contents
- Digestive Enzymes:
- Proteases: trypsin and chymotrypsin: proteolysis
- Pancreatic Lipase: Hydrolysis of triglycerides
- Amylase: Hydrolysis of carbohydrates
- Other Pancreatic Enzymes: Ribonuclease, deoxyribonuclease, gelatinase and elastase
- Bicarbonate and water:
- Bicarbonate neutralizes acid coming into small intestine from stomach
- Produced by carbonic anhydrase → secreted into the lumen of the duct → pancreatic juice
Control of pancreatic exocrine secretions
- Vagus nerve:
- Low level stimulus via Ach in response to anticipation of meal
- Enteric endocrine system:
- Cholecystokinin:
- secreted in duodenum
- Stimulated by partially digested proteins and fats
- CCK released into blood → binds to pancr acinar cells
- secreted in duodenum
- Secretin:
- Secreted in proximal small intestine
- In response to acid in duodenum
- Stimulates duct cells to secrete water and bicarb
- Secreted in proximal small intestine
- Gastrin:
- Secreted by stomach
- in response to gastric distension and irritation
- Stimulates acinar cells to secrete digestive enzymes
- Secreted by stomach
- Cholecystokinin:
Occurs in three phases: cephalic → Gastric → Intestinal
JC 2019
Examiner Comments
2024B 05: 42% of candidates passed this question.
Unfortunately, this question had an error in the wording. It should have read “Describe the exocrine secretions of the pancreas including their function and regulation”. This error was considered during marking. Despite this most candidates realised that the functions of the exocrine pancreas are secretory and went onto to provide an overview of the exocrine pancreatic cells and their secretions including the usual volume per day. Expected information included descriptions of the digestive enzymes and their role in protein, fat and CHO digestion and a description of bicarbonate formation and its functions including stomach acid neutralisation and optimisation of pH for enzyme function. Regulation of secretion was best described using the structure of digestive phases; cephalic, gastric and intestinal and the events linked to each. Extra detail on the hormonal and PNS factors, their effects and how they’re stimulated elevated the answer.
6. Describe the following with respect to lactate: (a) production (40% of marks). (b) metabolism (30% of marks). (c) and its role (30% of marks).
CICMWrecks Answer
Lactate is the conjugate base of lactic acid (an organic 3-C acid)
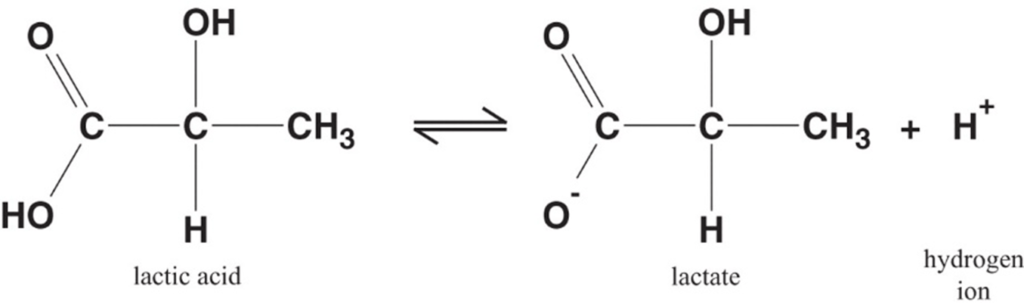
Production:
- It is produced by anaerobic metabolism of pyruvate either:
- Physiologically → in RBC (no mitochondria), renal medulla (↓ PO2), cornea/ lens (↓ PO2) → hence, normal plasma [lactate] is 0.5-2 mmol/L (and NOT zero!)
- Pathologically → reduced tissue perfusion and/or O2 delivery (Eg. shock, hypoxaemia) → thus, plasma [lactate] ↑↑↑ (> 2 mmol/L)
- Plasma [lactate] is 0.5 – 2 mM (and NOT zero) due to physiological production → it can be measured clinically as an indicator of anaerobic metabolism (Ie. ↑ anaerobic metabolism due to pathological situations lead to ↑ [lactate])
Fate of lactate:
- Persistent anaerobic metabolism (Ie. ongoing hypoxia) causes an accumulation of cellular lactate → this diffuses out of the cell into plasma along its [ ] gradient → lactate can then be:
- Used as a fuel source by the heart and brain
- Transported to the liver where it is:
- Converted back to glucose via gluconeogenesis (requires 6x ATP), which is then transported back peripherally for use → “Cori cycle”
- Converted to pyruvate intermediate → utilised locally in TCA cycle for ATP production via oxidative phosphorylation
- Resolution of hypoxia (Ie. tissue O2 tension restored) → intracellular lactate can be oxidised back to pyruvate for use in local tissue aerobic metabolism (Ie. fed into TCA cycle)
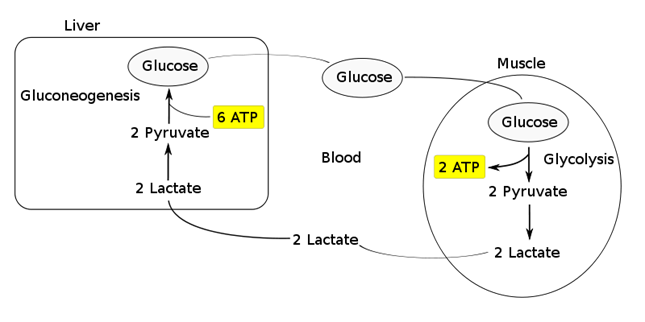
Functions of Lactate:
Lactate Sink:
- Lactate acts as a sink in heart, liver, muscle etc, allowing a period of ongoing ATP production from glycolysis when:
- cells become oxygen deplete
- Kreb’s cycle is inhibited
- Other causes of pyruvate accumulation: circulating catecholamines, exercise, sepsis or lack of mitochondria (RBCs)
Lactate Shuttle:
- Intracellular shuttle:
- Lactate may be shuttle out of:
- mitochoncrial membrane
- peroxisomes
- into cytoplasm of myocytes, neurons, astrocytes
- Ixydised by cytoplasmic LDH to pyruvate, generating NADH for energy use
- Lactate may be shuttle out of:
- Intercellular shuttle:
- Excess lactate, formed within fast-twitch fibres, is transported to other cells within the body with the oxidative capability to metabolize lactate, such as type I (slow-twitch) muscle cells, enhancing their excitability and limiting fatigue
- Furthermore, once in circulation, lactate attaches to red blood cells (RBCs) and is disassociated in the liver where inter-conversion via gluconeogenesis facilitates glucose formation, providing an alternative aerobic energy source.
Lactate as a signaling molecule:
- Redox signaling by intracellular shuttles
- Gene expression
- Increased intracellular levels of lactate can act as a signalling hormone, inducing changes in gene expression that will upregulate genes involved in lactate removal, and stimulates mitochondrial biogenesis.
- Control of lipolysis
- the shuttle regulates FFA mobilization by controlling plasma lactate levels.
- lactate functions to inhibit lipolysis in fat cells through activation of an orphan G-protein couple receptor (GPR81) that acts as a lactate sensor, inhibiting lipolysis in response to lactate
Bianca / JC 2021
Examiner Comments
2024B 06: 36 % of candidates passed this question.
A good description of lactate production should include the sites of production with some estimate of daily production. In addition, it was expected that answers would cover synthesis via glycolysis, pentosephosphate pathway (HMP shunt), the chemical reaction catalysed by LDH along with conversion of NADH to NAD. Lastly an explanation of the mechanisms other than anaerobic conditions that lead to an increased lactate production was required.The expected information for metabolism included an overview of hepatic uptake and the Cori cycle, the role of renal metabolism and excretion and mitochondrial tissue handling.With regards to its role, marks were awarded for a discussion of its use as a fuel for cells (including the myocardium, erythrocytes, astrocytes and skeletal muscle). Lactate’s role as a neurotransmitter and in autoregulation including cerebral vasculature rounded out this section.
7. (a) Categorise bacteria by their gram stain appearance and shape. Give TWO examples of bacteria for each group (30% of marks).
(b) Provide TWO examples of antimicrobials used against each group and outline their mechanism of action (20% of marks).
(c) Outline the mechanisms of bacterial resistance with ONE example for each (50% of marks).
CICMWrecks Answer: Classify Bacteria and antimicrobials
Gram Stain
Method of differentiated bacteria according to their cell wall characteristics, using
staining with crystal violet dye
- Gram positive bacteria have a thick outer cell wall composed of peptidoglycan,
which stains positive with crystal violet dye - Gram negative bacteria have an outer cell membrane enclosing a thinner
peptidoglycan cell wall, which has decreased affinity to the crystal violet dye
Classification of bacteria
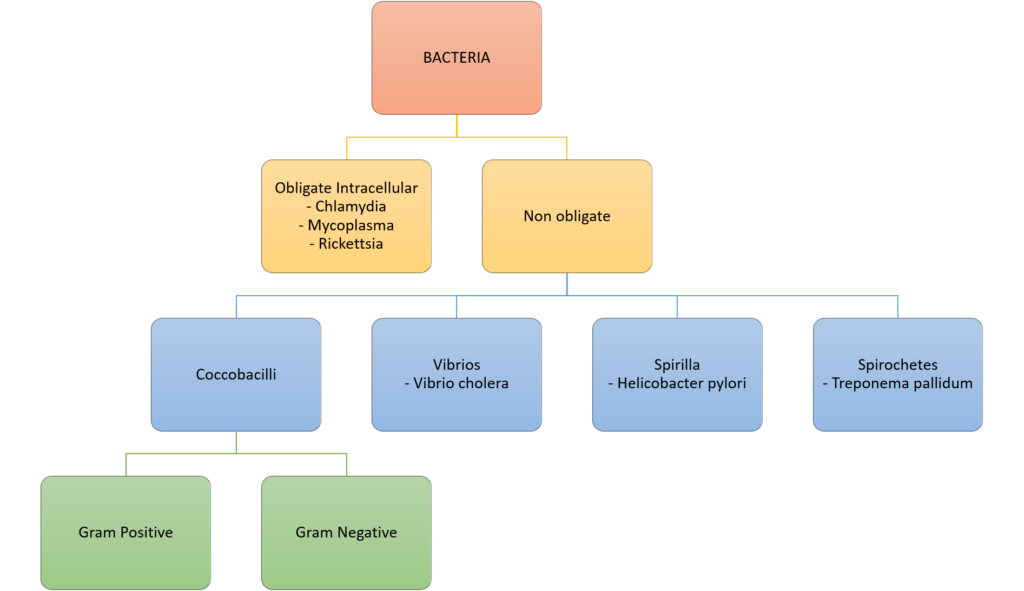
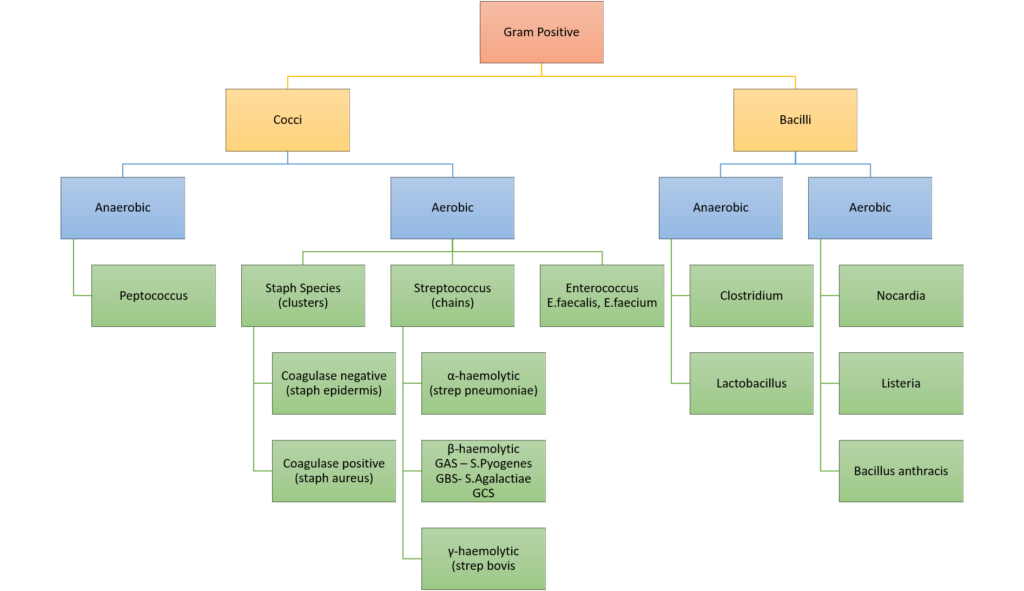
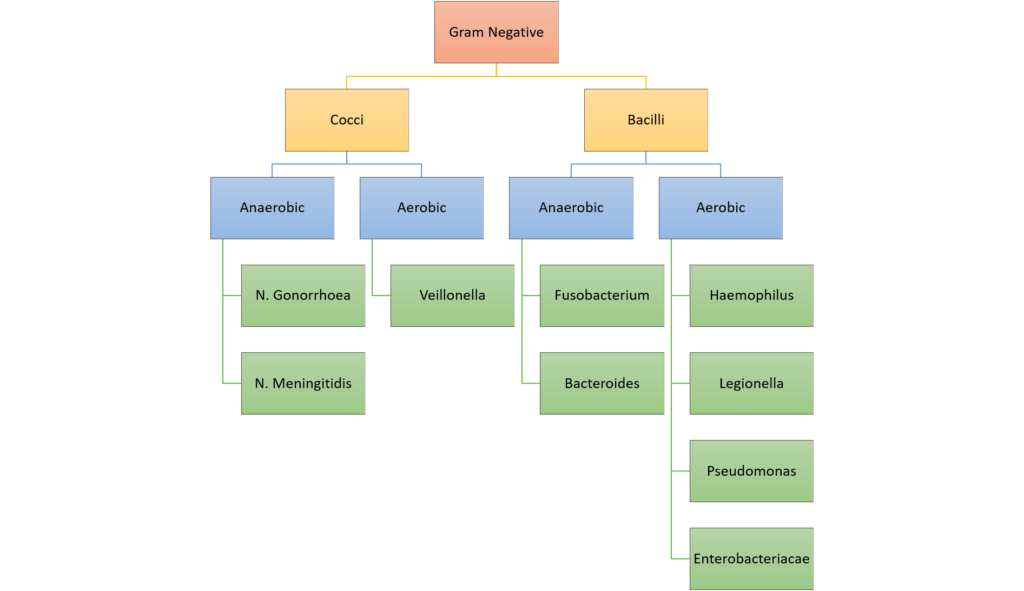
Examples of Antimicrobials + MoA
Group | Antimicrobials | Mechanism of Action |
---|---|---|
Gram Positive Cocci | Penicillin | β lactam antibiotic Binds to penicillin binding protein (transpeptidase) and prevents crosslinking of bacterial peptidoglycan impairs cell wall synthesis |
Vancomycin | Inhibits Glycopeptide synthetase prevents peptidoglycan formation in bacterial cell well (Unlike penicillins, prevents the transfer and addition of the muramylpentapeptide building blocks that make up the peptidoglycan molecule itself.) May also alter membrane permeability and selectively inhibit RNA synthesis. | |
Gram Positive Bacilli | Clindamycin | Inhibitor of bacterial 50S ribosomal subunit Prevents protein synthesis |
Erythromycin | Inhibits 50s subunit to prevent protein synthesis Bacteriocidal/static | |
Gram Negative Cocci | Ceftriaxone | Beta lactam ring binds to multiple penicillin binding proteins (carboxy/endo/ transpeptidase) and prevents crosslinking of bacterial peptidoglycan inhibits cell wall synthesis BROADER SPECTRUM Bacteria eventually lyse due to ongoing activity of cell wall autolytic enzymes (autolysins and murein hydrolases) while cell wall assembly is arrested Configuration: Stability against Betalactamases |
Ciprofloxacin | Bacteriocidal antimicrobials that block DNA replication by blocking tropoisomerase enzymes, which are essential for the supercoiling, replication and separation of circular bacterial DNA | |
Gram Negative Bacilli | Gentamicin | bactericidal Binds to the bacterial 30S ribosomal subunit to inhibit protein synthesis and thus bacterial growth |
Meropenem | Binds to several penicillin binding protein and prevents crosslinking of bacterial peptidoglycan inhibits cell wall synthesis BROADEST SPECTRUM Bacteria eventually lyse due to ongoing activity of cell wall autolytic enzymes (autolysins and murein hydrolases) while cell wall assembly is arrested Configuration: Stability against Betalactamases and ESBLs |
Gladwin / Sakurai / JC 2020
CICMWrecks Answer: Antibiotic resistance
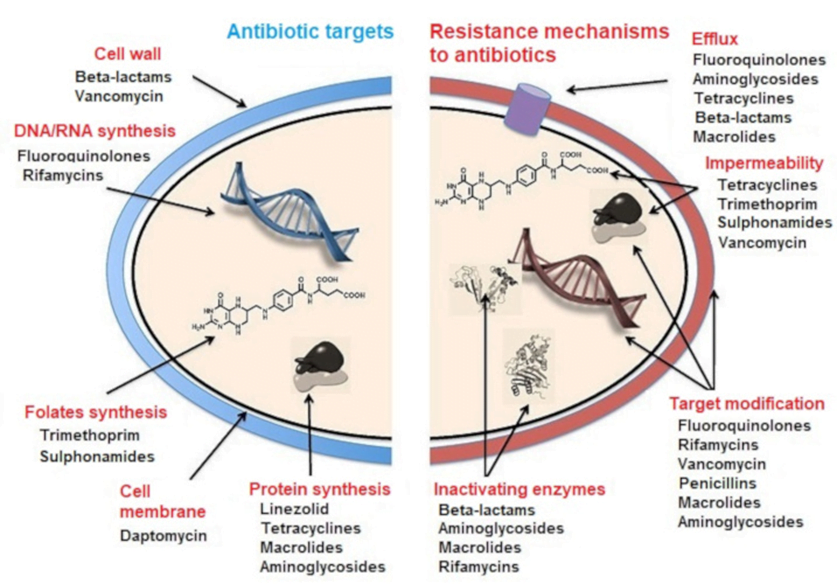
MECHANISMS OF ANTIBIOTIC RESISTANCE
- Mechanisms of Antibiotic Resistance can be classified broadly:
- Efflux Pumps
- Blocked Penetration / Alteration in access to target site
- Target Modification
- Modification of Drug or pathways
- There might be multiple resistance mechanisms at play in the same organism
Efflux Pumps: Increased efficiency or expression of efflux pumps (inner membrane proteins). | |
Removed from cell | Active transport of ciprofloxacin out of the bacterial cell |
E.Coli to tetracycline. | |
Trapped between cell wall layers | glycopeptide resistance in VRSA. |
Blocked Penetration / Alteration in access to target site | |
Narrowing of porin channels | Streptococcal resistance to penicillins typically occurs by reducing access to PBPs. |
Alteration of porins in gram negative outer membranes | downregulation of Outer Membrane Proteins (eg enterobacteriaceae, pseudomonas vs penems/cephs) |
increased selectivity of Outer Membrane Proteins (eg klebsiella outbreaks) | |
Loss of non-essential transporter channels | Anaerobes have no oxygen-transport channel which prevents penetration by aminoglycosides (Gentamicin) |
Reduced binding of the antibiotic | VanA and VanB vancomycin resistance involves a gene mutation leading to decreased affinity of vancomycin for the binding sites of peptidoglycan precursors |
Changes to the DNA-binding surface of DNA supergyrase infers resistance against ciprofloxacin | |
Target Modification | |
Modifying the enzyme that the antibiotic inhibits | Rifampicin resistance by point mutations, insertions, or deletions in RNA polymerase gene |
Production of an alternative enzyme for that which the antibiotic inhibits | MRSA develop or acquire the gene mecA which synthesizes an additional penicillin binding protein that enables it to continue cell wall synthesis in the presence of a beta lactam drug |
Overproduction of the target of the antibiotic | Trimethoprim DHFR enzyme overproduction in Escherichia coli and Haemophilus influenzae. |
Synthesis of target-protecting proteins | Ribosomal protection proteins against Tetracyclines in multiple Gram positive and gram negative bacteria |
Modification of Drug or pathways | |
Development of metabolic pathways to bypass site of action of antibiotic | Resistance to TMP-SMX by allowing bacteria to synthesize or absorb folic acid. |
Enzymes produced to Metabolize the drug | β-lactamases and cephalosporinases hydrolyse β-lactam rings |
Enzymes produced that add a chemical group to antibiotic to inhibit its activity | Aminoglycoside resistance by Staphylococcus aureus or Pseudomonas (Multiple enzymes acetyltransferase, adenyltransferase, and phosphotransferase) |
Spread of Bacterial Resistance
- Selective pressure selects for favourable mutations of resistance
- mosaic genes (from other bacteria eg strep pneumo from strep mitus) or uptake of DNA from environment
- transfer of resistant bacteria from person to person
- horizontal gene transfer;
- transduction – acquisition of bacterial DNA from a phage (a virus that propagates in bacteria);
- transformation – uptake and incorporation of free DNA released into the environment by other bacterial cells;
- conjugation – gene transfer (usually on plasmids), by direct cell-to-cell contact through a bridge.
Sources:
Microbiology Lippincott Williams & Wilkins
https://courses.lumenlearning.com/microbiology/chapter/drug-resistance/
https://www.encyclopedie-environnement.org/en/health/antibiotics-antibiotic-resistance-and-environment/
Gladwin / Sakurai / JC 2019
Examiner Comments
2024B 07: 49% of candidates passed this question.
The first part of the question asked candidates to categorise bacteria by gram stain and shape. A description of the gram stain procedure or discussion of bacterial cell wall characteristics was not required. Grouping of bacteria into gram positive cocci, gram positive bacilli, gram negative cocci, gram negative bacilli and others was expected with appropriate examples. A list of two antimicrobials that can be used against each of these classifications followed and a brief outline of their mechanism of action was expected. The largest weighting of marks was reserved for the third component of the question. Mechanisms of resistance included innate and acquired. Aspects of acquired resistance expected were genetic factors that alter the bacterial target, changes in bacterial permeability/efflux pumps and presence of enzymes that modify or negate the drug.
8. Outline the following with respect to ultrasound:
(a) The physical principles when used for tissue imaging using the following headings:
i. generation and detection of the ultrasound; ii. reflection and scattering; iii. attenuation; iv. refraction. (35% of marks)
(b) The relationship between transducer properties, image resolution and tissue penetration. (35% of marks).
(c) The principles of the doppler effect and its applications. (30% of marks).
CICMWrecks Answer
Ultrasonography Principles
- Definition
- A sound wave with a frequency > 20 kHz → higher than frequency range audible by human ear
- Used medically → typically involves frequency range of 2-15 MHz
- Piezoelectric and converse piezoelectric effect
- Change of polarization of molecules in a quartz crystal in response to
mechanical stress – Interconverts electrical and sound energy - Application of electrical field creates mechanical deformation in a crystal
- Change of polarization of molecules in a quartz crystal in response to
- US Generation: Piezoelectric crystal within probe is stimulated by electrical current to vibrate → produce sound wave
- US Detection: Sound wave reflected by medium causes same crystals to vibrate → produce electrical signal
- Piezoelectric transducers in US
- Electrical current converted into precise sound waves (1~20mHz)
- Sound waves reaches interface of two mediums of differing density (or acoustic impedance).
- Acoustic impedance = tissue density x acoustic velocity
- unique to tissue type (e.g. fat, bone, etc.)
- At interface:
- Reflection
- sound wave reflected directly back to transducer
- Amount of reflection depends of ratio of acoustic impedance (or density)
- Increased density ~ increased reflection
- Refraction
- sound wave is deflected within the medium
- based on Snell’s law
- Reflection
- Attenuation: Loss of energy or strength of an ultrasound wave as it travels through a medium. Occurs through:
- Absorption: The ultrasound wave energy is converted into heat as it interacts with the tissue.
- Reflection: The sound waves bounce off the boundaries between tissues with different acoustic impedances.
- sound wave reflected back to transducer
- Amount of reflection depends of ratio of acoustic impedance
- Increased density ~ increased reflection
- Scattering: The sound waves are deflected in various directions by irregularities in the tissue and do not reach transducer
- Refraction: bending of the sound wave as it travels from one tissue to another
- results in a change in the wave’s direction
- relationship between the angle of incidence (θi), the angle of refraction (θt), and the speeds of sound (c1 and c2) in the two media is described by Snell’s Law: sin(θi) / c1 = sin(θt) / c2
- Central Processor
- Electrical current generated by piezoelectric crystal signaled to CPU
- CPU calculates the distance between transducer and object according to
- Speed of sound (1540m/sec)
- Delay in echo return
- Information relayed to display for visualization
- Gain
- Sensitivity of CPU to signals received from transducer
- Time-Gain Compensation – selective sensitivity of CPU to different interval of sound delay
Resolution and Penetration
- Ultrasound Resolution:
- Defined as the ability to differentiate b/t structures that are closely related
- Resolution is ↑ with either:
- (i) ↑ frequency (or ↓ wavelength) of sound wave → but this ↓ tissue penetration
- (ii) ↑ amplitude of sound wave → but this ↑ artefact
- (iii) ↑ gain → but this ↑ noise
- Types of Resolution: Spatial (Axial, Lateral, Elevational), Temporal
- Spatial Resolution:
- Axial Resolution: The ability to distinguish two structures that are side-by-side and parallel to the ultrasound beam.
- Achieved with a higher frequency and shorter pulse length.
- Mathematically, it’s half the spatial pulse length.
- Lateral Resolution: The ability to distinguish two structures that are side-by-side to the ultrasound beam.
- Achieved with a narrower ultrasound beam, which is related to the width of the beam.
- Higher frequencies generally lead to narrower beams and better lateral resolution.
- Lateral resolution is roughly three times worse than axial resolution at the focal region of the beam.
- Elevational resolution (aka slice thickness resolution)
- refers to the ability to distinguish structures that are close together in the direction perpendicular to the imaging plane. (similar to lateral resolution, but in perpendicular plane)
- crucial for accurately visualizing the depth and thickness of tissues
- Temporal Resolution:
- The ability to distinguish between instantaneous events of rapidly moving structures.
- Achieved with a high frame rate.
- A higher frame rate means the ultrasound machine can capture and display more images per second, allowing for better visualization of movement.
- Axial Resolution: The ability to distinguish two structures that are side-by-side and parallel to the ultrasound beam.
- Ultrasound penetration:
- defined as the depth to which ultrasound waves can travel into tissue
- Primarily determined by the frequency of the ultrasound waves, with lower frequencies generally penetrating deeper than higher frequencies.
- Trade-off between Resolution and Penetration:
- There’s an inherent trade-off between image resolution and penetration depth.
- Higher resolution requires higher frequencies, which means less penetration, and vice versa.
Modes of Ultrasound
- A (amplitude scan): Amplitude of U/S signal plotted against time → provides information about tissue depth (BUT is no longer used)
- B (brightness): Depth recorded as bright spot (rather than a spike as in A-mode) →
amplitude of U/S signal is proportional to brightness - M (motion): B-mode plotted against time (Ie. assess heart valve movement over time)
- 2-D: Sequential B-mode across 90° (most commonly used) → requires an array of crystals
- Doppler: Uses “Doppler shift” to establish velocity of moving object which is reflecting sound waves → superimposed on 2D mode with colours representing direction of movement (red = towards, blue = away)
Doppler effect
- Change in apparent frequency of sound for an observer moving relative to its source
- Use in ultrasound
- Sound waves reflected off objects moving toward or away from transducer
(usually blood)- If object moving toward transducer, frequency appears increased – displayed as red (however this is not standardized across machines)
- If object moving away from transducer, frequency appears decreased –
displayed as blue
- Can be used to measure velocity of flow, as well as direction
- Sound waves reflected off objects moving toward or away from transducer
Sakurai 2016
Examiner Comments
2024B 08: 75% of candidates passed this question.
In this context an “outline” question requires a definition of the principle followed by a brief description or overview. Good answers used the structure of the question to provide a definition of the concepts of generation/ detection/ reflection/ scattering/ attenuation and refraction and then used this to describe how the ultrasound image is produced or disrupted due to these factors. The discussion around resolution required candidates to link how the transducer and its frequency affects axial, linear, contrast and temporal resolution. The principle underpinning this is the relationship between frequency/wavelength, tissue penetration and image resolution. The doppler effect component of this question required an explanation of the doppler effect and how it is used in medical ultrasound for example measurement of blood flow and direction. The Doppler equation was not required for full marks though did help some candidates explain the doppler effect.
9. Compare and contrast metoprolol and verapamil using the following headings:
(a) Class and indications for use (20% of marks).
(b) Mechanisms of action (25% of marks).
(c) Pharmacodynamics and adverse effects (55% of marks).
Examiner Comments
2024B 09: 21% of candidates passed this question.
The most effective format for this question was to split information into the headings provided. The higher scoring responses were done as a table, with headings as per the question, concise facts and clear comparisons. Information on pharmaceutics, dose and pharmacokintetics were not required. When effects of the drugs were discussed detail regarding the mechanism of pharmacodynamics and adverse effects was expected. Cardiovascular effects of these drugs required specificity in their description and included their action on the duration and slope of the cardiac action potential thus affecting the HR, as well as effects on SVR and BP. Other adverse effects included respiratory, neurological and gastrointestinal actions.
10. Outline the mechanisms of action and adverse effects of the following:
(a) Biguanides (35% of marks).
(b) Sodium-Glucose cotransporter – 2 (SGLT-2) inhibitors (SGLT-2) (35% of marks).
(c) Sulfonylureas (30% of marks).
Examiner Comments
2024B 10: 52% of candidates passed this question.
The information required for this question was largely self-explanatory. Higher scoring answers were able to demonstrate understanding by providing some discussion and context with regards to adverse effects rather than a simple list.
11. (a) Explain the mechanisms responsible for the cell resting membrane potential (70% of marks).
(b) Describe the Gibbs Donnan effect (30% of marks).
CICMWrecks Answer
Equilibrium Potential
Nernst Equation
The potential difference generated by a permeable ion in electrochemical equilibrium when there are different concentrations on either side of the cell can be calculated via the Nernst Equation:
where
E is the equilibrium potential for the ion
R is the gas constant (8.314 J.K-1.mol-1 )
T is the temperature in Kelvin
F is Faraday’s Constant
z is the ionic valency (e.g. +2 for Mg2+, -1 for Cl–)
EK = -90 mV
ENa = +55mV
ECl = -65mV
Goldman-Hodgkin-Katz Equation
The Nernst equation describes the equilibrium potential for a single ion, and assumes that the membrane is completely permeable to that ion.
However, calculation of membrane potential requires examining the effects of many different ions with different permeability. This can be performed with the Goldman-Hodgkin-Katz equation:
where,
Px is the permeability constant for the ion, x
If the membrane is impermeable to x, then Px = 0
Note that:
This model does not consider valency
The concentrations of negative ions are reversed relative to positive ions
Resting Membrane Potential
- Definition: The potential difference (units volts) that exists across the cell membrane (i.e. between the intracellular and extracellular environment) when the cell is in a unexcited state, where the reference voltage is the extracellular environment.
- Resting Membrane Potential (RMP) is the result of two key properties of cells:
- the cell membrane is semi-permeable, that is it has variable permeability to different species in solution.
- differing concentration gradients exist across the semi-permeable cell membrane for different ions in solution, the most important determinant of these gradients being the Na+-K+-ATPase.
- At rest, membranes are:
- Permeable to potassium
- Impermeable to other ions
- Generation of membrane potential:
- Intracellular potassium concentration is much higher than extracellular potassium concentration – Due to the action of the Na+-K+pump.
- As the membrane is permeable to potassium, potassium will attempt to diffuse down this gradient, generating a negative intracellular charge which opposes further diffusion
- At some point, an electrochemical equilibrium is reached between:
- The concentration gradient dragging potassium out of the cell
- Negative electrical charge pulling it in
Determinants of RMP
- K+ Diffusion Potential
- From the Nernst equation above we can see that the normal RMP of most tissue is relatively close to the K+ equilibrium potential (-94mV). From this (and the Goldman equation) we can infer that the membrane is likely to be most permeable to K+ at rest. Indeed the primary determinant of the RMP is K+. Relative permeability of the membrane to K+ vs Na+ is 100:1.
- This has the corollary that changes in K+ concentration will have the most major effect on RMP. This is particularly the case with [K+]o due to its low value – small absolute changes in [K+]o are a relatively large proportion of [K+]o.
- Note that while the RMP is as close (or closer) to the equilibrium potential for Cl–, Cl– is not the primary determinant of the RMP as the concentration gradient for Cl– is largely the passive result of the electrochemical gradient created by the Na+-K+-ATPase.
- Na+ Influx
- While the resting membrane is very impermeable to Na+ the electrochemical gradient for its movement into the cell is so large that there is a small ‘leak current’ of Na+ into the cell.
- This Na+ leak is the single factor responsible for most of the deviation of the RMP from the equilibrium potential for K+ (contributes roughly +8mV to the RMP).
- Na+-K+-ATPase
- As noted there is a constant slow leak of Na+ into the cell. Because of the deviation away from the K+ equilibrium potential that this causes, there is also an electrochemical gradient to cause a slow leak of K+ out of the cell. As such the Na+-K+-ATPase is essential to maintain the relative concentration gradients of these ions and thus the RMP.
- In addition the Na+-K+-ATPase itself is electrogenic, transferring as it does 3 Na+ out for every 2 K+ pumped in, leaving a net negative charge balance on the inside of the cell membrane. This contributes roughly -4mV to the RMP.
- Gibbs-Donnan Effect
- The Gibbs-Donnan effect accounts for the effect of non-diffusible ions on the RMP. In vivo the high concentration of negatively charged intracellular proteins has a small but significant effect on RMP. The presence of this net fixed negative charge on the inside of the cell effects the distribution of permeable ions across the membrane.
Resting Membrane Potential in Different Tissues
Typical values of RMPs:
- Ventricular Myocyte: -90mV
- Cardiac Pacemaker cell: -60mV
- Skeletal Muscle cell: -80mV
- Myelinated Axon: -70mV
Gibbs – Donnan Effect
- Describes the tendency of diffusable ions to distribute themselves such that the ratios of the concentrations are equal when they are in the presence of non-diffusable ions.
- Occurs when:
- A semi-permeable membrane separates two solutions
- At least one of those solutions contains a non-diffusable ion
- The distribution of permeable charged ions will be influenced by both their valence and the distribution of uncharged ions, such that at equilibrium the products of the concentrations of paired ions on each side of the membrane will be equal:
- The two main contributors to the Gibbs-Donnan effect in the body are sodium and protein. This occurs because cell membranes:
- Are impermeable to protein
Intracellular protein concentration is high. - Effectively impermeable to sodium
Due to the Na+-K+ ATP-ase pump.
- Are impermeable to protein
- Changing Gibbs-Donnan equilibriums also change the tonicity on each side of the cell membrane, causing movement of water which then upsets the Gibbs-Donnan effect – therefore there is no stable state.
- The Gibbs-Donnan Effect is important for:
- Maintenance of cell volume
Na+ acts as an effective osmole, reducing cellular swelling. - Plasma oncotic pressure
Increased plasma ion concentration increases oncotic pressure. - Resting Membrane Potential
- Maintenance of cell volume
JC 2019
Examiner Comments
2024B 11: 57% of candidates passed this question.
This question required a description of the separation of charge across the cell membrane due to selective ion permeabilities and concentrations. The equilibrium voltage of an individual ion was best expressed using the Nernst Equation and of multiple ions by the Goldman-Hodgkin-Katz equation. Better responses were able to use the correct eponym, constants and/or logarithmic transformation (from the ln to log10). The fundamental role of potassium conductance and the Na/K ATPase was also expected. The Gibbs-Donnan relationship was allocated 30% of marks so an explanation of the role of negatively charged intracellular proteins and the effect on ionic flux was expected.
12. Describe the physiological factors that affect the arterial partial pressure of carbon dioxide. (Measurement factors are not required).
CICMWrecks Answer
PaCO₂ is determined by the balance of production and elimination.
CO2 production:
- Normally 200ml/min (compared to average oxygen intake of 250ml/min)
- Byproduct of aerobic metabolism
- Produced in the cell mitochondria via TCA
- Entry to TCA as acetyl CoA (from CHO, FA, aa)
- TCA products
- CO2
- Reduced compounds FADH and NADH
- H+ ions
- 2 molecules of CO₂produced for each 1 molecule of ATP
- Amount of CO2 produce per molecule of substrate varies with the substrate.
- 1.5 Mole CO₂ per mole of Glucose
- Respiratory quotient
- R = CO2 production / O2 consumption
- Depends on fuel substrate
- 0.7 for fat, 1.0 for CHO
- 0.8 for typical western diet
CO2 Storage and transport
- ~ 120 litres predominantly as bicarbonate ion
- CO2 is carried in the blood in 3 forms – bicarbonate (70-80%), carbamino compounds (20-25%), dissolved (5%)
- Bicarbonate:
- Main form of CO2 delivery in blood
Via Hendersen-hasselbach:
CO2 + H2O ⇔ H2CO3 ⇔ H+ + HCO3–
- Main form of CO2 delivery in blood
- Carbamino compounds:
- CO2 combines with terminal amines (mainly Hb) to form carbamic acid dissosciates → at physiological pH to carbamate
- Dissolved:
- CO2 ~20 x more soluble than O2
- Henry’s law: p=kHc (Given a constant temperature, the amount of gas dissolved in a liquid, is proportional to the partial pressure of that gas. where c=solubility of a gas at a fixed temp, k=Henry’s Law (equilibrium) constant p = partial pressure of the gas))
- thus via Henry’s Law more CO2 is dissolved
Elimination:
- Small amount dissolved in sweat
- Most via lungs
- PaCO₂ ~ PACO₂
- PACO₂ determined by alveolar ventilation equation
Control of Ventilation
Afferents
- Chemoreceptors
- Central: retrotrapezoidal nucleus – sensitive to changes in CSF [H+]
– ↑CO2 → ↑[H+] → stimulates respiration - Peripheral: Aortic bodies (Vagus) and Carotid bodies (Glossopharyngeal)
– sensitive to PO2, [H+], PCO2 and blood flow, CO2
– ↑CO2 → ↑ respiration
- Central: retrotrapezoidal nucleus – sensitive to changes in CSF [H+]
- Baroreceptors:
- Aortic arch and carotid sinuses
- ↓MAP → less stretch → ↑ sympathetic outflow from medullary vasomotor centre → ↑respiration
Pulmonary Receptors
- Stretch receptors:
- Inflation reflex: ↑stretch inhibits inspiration
- Deflation reflex: collapse inhibits expiration
- J Fibers:
- Nociceptive mechano-chemoreceptors
- On stimulation → Bronchospasm, apnoea, bradycardia and hypotension
Others: Joint and muscle receptors, pain and temperature
Central control of breathing
- Central input: Hypothalamus and cortex (cortex can overrise medulla)
- Controller:
- Medullary resp centre: Dorsal resp group (Nucleus tractus solitarius – inspiration and timing), Ventral resp group (Pre-botzinger, Botzinger – control of respiration, airway dilatation, central pattern generation)
- Pontine respiratory group: Pneumotaxic centre (resp rate and pattern of breathing), Apneustic centre (intensity of breathing)
- Efferents:
- Phrenic nerve – diaphragm
- Spinal nerves to intercostal muscles
- Brachial plexus to pec major
- Accessory muscle to sternocleidomastoid
- Spinal nerves to abdo muscles
Factors which ↑PaCO₂
- ↑Shunts or ↑V/Q scatter → ↑PaCO₂
- ↑ Alveolar CO2 → ↑PaCO2
- ↑ Production (VCO2)
- During vigorous exercise
- Moderate exercise doesn’t effect pCO2 and may actually result in ↓pCO2 due to ↑MV
- Hypermetabolic states (Sepsis, Fever, MH, neurolept malignant syndrome)
- Altered (ie pure carb) diet
- ↑ Barometric pressure/↓Pressure of water
- ↑ Inspired CO₂
- Determined predominantly via alveolar ventilation which depends on:
- Dead space (DS):
- PE, low CO state, ↑d PEEP → ↑West’s zone
- ↑DS → ↓Alveolar Vent → ↑Alveolar CO2 → ↑PaCO2
- Respiratory Rate: ↓RR → ↓Alveolar Vent → ↑Alveolar CO2 → ↑PaCO2
- Tidal Volume (Tv): ↓Tv → ↓Alveolar Vent → ↑Alveolar CO2 → ↑PaCO2
- Dead space (DS):
- ↑ Production (VCO2)
Gladwin / Mooney / Sakurai / JC 2020
Examiner Comments
2024B 12: 30% of candidates passed this question.
This question was broad and required content from several areas of the syllabus. Given this breadth less detail expected. A good answer used a structured approach discussing CO2 production, carriage in the blood, CO2 elimination and the control of ventilation, providing specific detail on how each of these affected arterial partial pressures of CO2.
“CO2 production” should include an overview of CO2 as a product of aerobic respiration, an approximation of the rate of production, how it varies with metabolic activity and finally the concept of RQ with its determinants. “Carriage” should include an estimate of CO2 content, how PaCO2 depends upon the amount dissolved (as per Henry`s Law), how CO2 is carried in the blood enabling more total content relative to the amount dissolved, the influence of the Haldane effect, and how CO2 is more soluble at lower temperature lowering PaCO2. “Elimination” should emphasise the importance of minute ventilation, dead space (including effective dead space due to high VQ lung units), and the effect of rebreathing CO2). “Control of ventilation” required a brief overview of the sensors, including central and peripheral chemoreceptors, integrators and effectors that influence the minute ventilation and ultimately PaCO2.
13. (a) Define ventricular diastole, including its usual duration (15% of marks).
(b) Describe the cardiovascular events that occur during this period (85% of marks). (ionic and cellular events not required).
CICMWrecks Answer
Diastole in the cardiac cycle corresponds with cardiac myocyte relaxation, and includes periods of isovolumetric relaxation and ventricular filling. It lasts approximately 430ms.
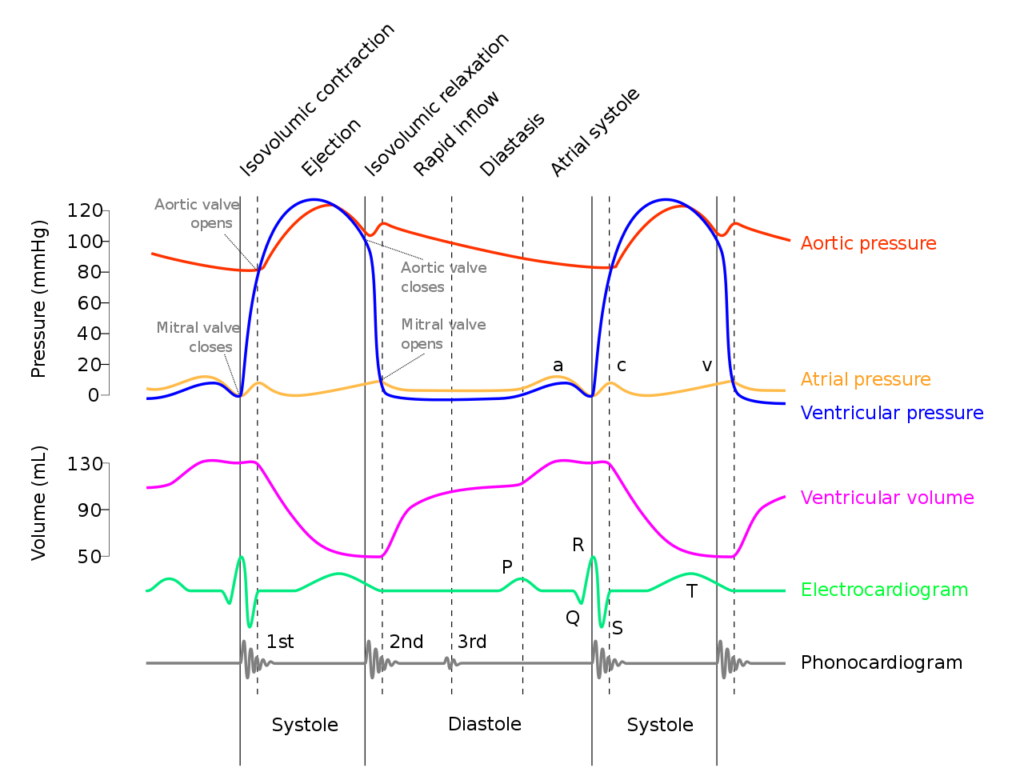
Isovolumetric relaxation
- As aortic pressure overcomes ventricular pressure, and the inertia of forward flow of blood out of the LVOT, the aortic valve closes
- The ventricular myocytes relax, while both the mitral (or tricuspid) and aortic (or pulmonary valves) remain closed
- The volume of blood within the ventricle remains constant (LV End-Systolic Volume ~50mls) , however ventricular pressure decreases from 120mmHg to close to 0mmHg (or 20 to 0mmHg in R ventricle
Ventricular filling
- Passive phase
- As ventricular pressure decreases below the atrial pressure, the mitral (or tricuspid) valve opens allowing forward flow of blood from the atria to the ventricles leading to a progressive increase in ventricular volume and pressure.
- The first two-thirds of the ventricular filling is due to passive filling
- Active phase
- 20% of ventricular filling is provided by the atrial kick during the final quarter of ventricular filling resulting in a slight increase in ventricular pressure
- Ventricular pressure overcomes atrial pressure and the mitral (or tricuspid) valves close
Electrochemical events
- The cardiac action potential is tied to contraction via excitation-contraction mechanism.
- Diastole corresponds to phase IV where voltage gated L and T-type Ca channels close, ceasing Ca influx, and ongoing K efflux repolarizes the myocyte.
- ECG
- The T wave corresponds with ventricular repolarization and occurs immediately prior to ventricular diastole.
- The QRS complex corresponds with ventricular depolarization and occurs immediately prior to ventricular systole
- Diastole corresponds with the terminal part of the T wave to immediately after the QRS complex
- Electrical current through cardiac cycles
- During depolarization, current runs from the base of the heart to the apex (corresponding to flow down bundle of His) the just before the end of depolarization, the direction of flow reverses (corresponding to flow down Purkinje fibres)
- During repolarization, the order reverses, from Purkinje system → Bundle of His
Coronary artery perfusion
- Due to the high ventricular pressures impeding blood flow during systole in a starling-resistor model, left ventricular perfusion (or right ventricular perfusion during pressure-overloaded states) occurs predominantly during diastole.
- As right ventricular pressures are significantly lower than aortic pressures even during systole, perfusion continues (in a normal right ventricle)
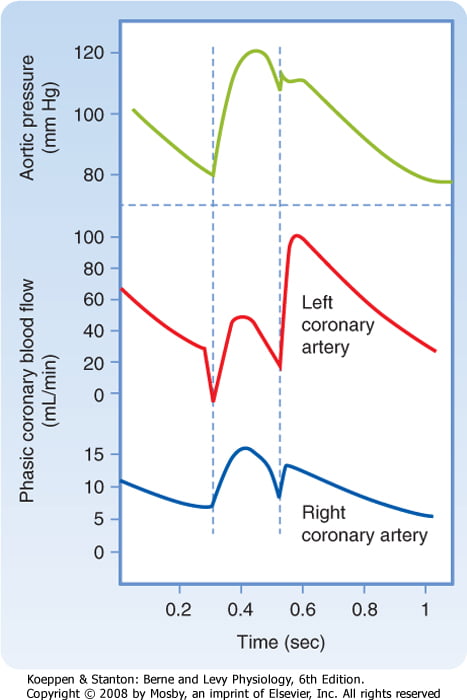
Sakurai 2016
Examiner Comments
2024B 13: 38% of candidates passed this question.
A thorough response to this question provided an accurate definition of ventricular diastole providing detail about duration (either duration at a certain heart rate or the usual ratio with changes due to tachycardia). The second part was best structured by dividing the events into cardiac mechanical and electrical events and circulatory changes. Mechanical events included a description of isovolumetric relaxation, early filling, atrial ejection and isovolumetric contraction, with an explanation of what marks the beginning and end of each of these phases as well as what happens to pressures within the cardiac chambers. Electrical events included the correlation of diastole with ventricular repolarisation and refractory periods as well as the corresponding ECG waves. Finally, an overview of the changes that occur in the coronary, pulmonary and systemic circulations during diastole was expected.
14. Explain how the kidney handles an acid load.
CICMWrecks Answer
Non-volatile acid
” An acid produced from sources other than carbon dioxide, which cannot be excreted in the lungs. “
- e.g. lactate, sulphate, phosphate and ketone bodies
- 70-100mmol non-volatile acid produced per day
- 4000-5000mmol HCO3– filtered by glomerulus per day
- Renal acid-base is largely determined by handling of bicarbonate ions
- 80% of filtered bicarbonate resorbed in proximal tubule
- 20% resorbed in distal tubule and collecting duct
- Urinary Buffers (Dibasic Phosphate and ammonia) play a role
Bicarbonate Resorption
Proximal tubule
In the tubular cell:
- H+ leaves the tubular cell through the apical membrane via
- H+/Na+ antiporter (secondary active transport via Na+/K+ ATPase on basolateral membrane)
- The HCO3– re-enters the circulation via the Na/HCO3– symporter
- H+ combines with HCO3– in tubule to form H2CO3
- H2CO3 switches back to CO2 and H2O, CO2 is resorbed to turn back into the tubular cell to generate more HCO3 for resorption
More bicarbonate resorbed when:
- Higher filtered HCO3–
- Higher PaCO2
- Lower luminal flow rate
- Angiotensin 2
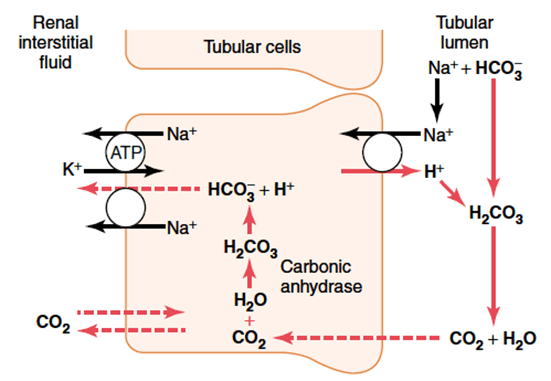
Distal convoluted tubule and collecting duct
- Similar mechanism with regards to resorption of HCO3– via secretion of H+
- Difference is that H+ is transported across the apical membrane via an H+ ATPase (active transport)
Role of Buffers
- The maximum urinary pH is 4.5
- To eliminate more protons, they must be buffered in the tubular fluid
- H+ is bound preferentially to buffers only in the presence of low tubular HCO3–
- Buffering, and so elimination of H+ without its conjugate HCO3–, requires resorption of HCO3– in the proximal convoluted tubule
Phosphate (HPO42-):
- Becomes concentrated in tubules
- pKa = 6.8, so operates effectively as a buffer at tubular pH
Ammonia
- Glutamine broken down in tubular epithelia cells to two NH4+ and two HCO3–
- Comes from hepatic amino acid metabolism
- The new HCO3– is transported back to the circulation via the Na/HCO3– symporter to re-enter the circulation
- NH4+ becomes ion trapped in the tubular fluid
- Proximal tubular fluid is not acidic enough to keep NH4+ in the tubule
- It is resorbed in the ascending limb, moves through the medulla to re-enter the collecting duct
- Here urine has a low pH, and so NH4+ can be eliminated with its proton attached
Mooney 2016
Examiner Comments
2024B 14: 34% of candidates passed this question.
This question was best answered through a description of how the entire nephron excretes acid. This required a detailed description of bicarbonate reabsorption, ammonium excretion and titratable acidity including phosphate. Explanations were expected to describe the locations, cells and the mechanisms or transporters involved in the resorption of bicarbonate or excretion of acid and how these changes depending on acid load.
15. (a) Outline the general classification of nerve fibres including details on their function, size and conduction speed (30% of marks).
(b) Describe the mechanisms of action potential generation and propagation along a myelinated peripheral nerve fibre (70% of marks).
CICMWrecks Answer
Classification of Nerve Fibres
(Erlanger and Gasser Classification)
Type | Appearance | Function | Diameter | Conduction velocity (m/s) |
---|---|---|---|---|
A – α | Large peripheral myelinated fibres | Motor | 10-20 µm | 60-120 |
A – β | Touch, pressure | 5-10 µm | 40-70 | |
A – γ | Proprioception | 3-6 µm | 15-30 | |
A – δ | Pain, temperature, touch | 2-5 µm | 10-30 | |
B | Small myelinated preganglionic ANS fibres in visceral nerves | Preganglionic ANS | 1-3 µm | 3-15 |
C | Small unmyelinated motor and sensory fibres | Pain/temperature | 0.5-1 µm | 0.5-2 |
Action potential generation and propagation
“Action Potential” (AP) → large rapid change in membrane potential that occurs in excitable cells
- AP = electrical response of neurons and other excitable tissues during which membrane potential rapidly ↑ and ↓
- All or nothing phenomenon
- Allow rapid signalling within excitable cells over long distances
- AP results from brief ↑ in membrane conductance to Na+, followed by slower ↑ in membrane conductance to K+
- Key parameters
- RMP -70mV
- Threshold potential -55mV
- Peak potential (depolarisation) +20-40mV
- Duration of AP 1-2ms
Events of an AP:
- Phase 1 – threshold potential: depolarisation stimulus reaches neuron → CM reaches -55mV → activation of voltage gated Na+ channels → Na+ influx > K+ efflux
- Phase 2 – AP: rapid influx of Na → further depolarisation → +ve feedback → rapid upstroke → drives membrane potential to Nernst potential for Na of ~+50mV → peak potential +30mV
- Phase 3 – repolarisation: AP never reaches theoretical max (+50mV) due to 2 events:
- Inactivation of voltage gated Na+
channels → ↓ membrane permeability to Na+ - Delayed activation of voltage gated K+
channels: ↑ membrane K+ permeability → K+
efflux → membrane potential driven back towards Nernst for K+ of ~-90mV - Membrane potential briefly more –ve than RMP = “after hyperpolarisation” – due to gradual closure of voltage-gated K+
channels
- Inactivation of voltage gated Na+
- Phase 4 – restoration of RMP
- -70mV maintained by: Na/K ATPase (EC gradient) + Na/K pump
Refractory period
- Time following an AP
- 1. Absolute Refractory Period:
- AP cannot be triggered whatever the size of the stimulus
- starts from when voltage-gated Na+ channels open → continues until repolarisation 1/3 complete
- 2. Relative Refractory Period:
- Repolarisation: K leak channels + voltage gated K channels open = K permeability is highest
- AP only with ↑↑stimulus to counteract ↑K+ efflux
- Important for 2 reasons:
- Ensure unidirectional propagation of APs
- Limiting frequency of APs
Saltatory conduction
- Saltatory conduction: propagation of AP along myelinated axons, whereby wave of depolarisation “jumps” from one rode of Ranvier to the next
- Mechanism of saltatory conduction
- Depolarisation of a node → influx of Na ions → creating a sink (area of –ve charge at the surface)
- +ve charge on nodes ahead flows into sink → ↓ polarity inside the membrane → AP → propagating current activates fast Na channels → wave of
depolarisation down axon - minimal electrical signal degradation as axon is insulated by myelin sheath
- AP reaches next node of Ranvier → continues down myelinated fibre
- Nerve impulses appear to rapidly jump from one node to the next
Bianca / Kerr 2016
Examiner Comments
2024B 15: 47% of candidates passed this question.
The first component of this question required a list of the different nerve fibre types including the breakdown of A, B and C fibres along with their primary function, for example motor/sensory/parasympathetic/sympathetic, presence of myelin, size and velocity of conduction.The second component of this question required the mechanism by which an action potential arises and is propagated in a myelinated nerve. This required a detailed overview of electrolyte movement across the neuronal membrane during depolarisation, timing and explanation of the refractory period, the basis for unidirectional movement and the mechanism of saltatory conduction which occurs in a myelinated nerve.
16. Outline the following with respect to platelets: (a) Formation (15% of marks). (b) Structure (40% of marks). (c) Function (45% of marks).
CICMWrecks Answer
PLATELET
Formation
- produced during hematopoiesis in a sub-process called thromopoiesis, or production of thrombocytes.
- Bone marrow: Common myeloid progenitor cells → promegakaryocytes → megakaryocytes
- Megakaryocytes produce protoplatelets within their cytoplasm → released in cytoplasmic extensions upon cytokine stimulus
- Protoplatelets break up into hundreds of platelets that circulate throughout the bloodstream
- The remaining nucleus of the ruptured megakaryocyte is consumed by macrophages.
- Megakaryocyte and platelet production is regulated by thrombopoietin (hormone produced by the liver and kidneys)
- Thrombopoietin stimulates differentiation of myeloid progenitor cells into megakaryocytes and causes the release of platelets.
- Thrombopoietin is regulated by a negative feedback mechanism based on platelet levels
- Each megakaryocyte produces between 5,000 and 10,000 platelets
- Altogether, around 1011 platelets are produced each day in a healthy adult
Fate
- Average lifespan of a platelet is 5 to 10 days
- Old platelets are destroyed by macrophage phagocytosis in the spleen and by Kupffer cells in the liver
- Up to 40% of platelets are stored in the spleen as a reserve, released when needed by sympathetically-induced splenic muscle contractions during severe injury.
Structure
- Small anucleated cells derived from megakaryocytes which originate from haematopoietic stem cell
- Normally 150~300 x 103 /μl
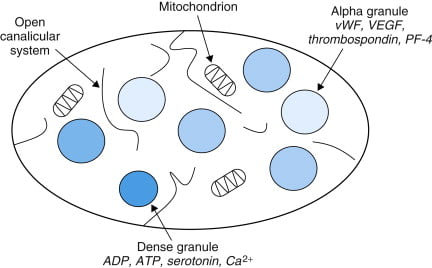
- Actin and myosin
- Remnants of the ER, SR storing calcium
- Mitochondria
- Enzyme systems → production of prostaglandins
- α granules
- Thrombin
- PDGF
- P-selectin
- Fibronectin
- vWF
- Dense granules
- ADP
- ATP
- Ca2+
- Serotonin
- Histamine
Function
- Haemostasis
- Formation of platelet plug
- Interact with collagen exposed at damaged endothelium by GPIa
- Activated by PAF, Thrombin
- Morphological change → irradiating pseudopods
- Degranulation
- Aggregation
- Expression of GPIIb/IIIa → binds fibrin and vWF
- Formation of platelet plug
- Immunomodulation
- Deployed to sites of inflammation and infection and secrete cytokines and chemokines
Sakurai / JC 2019
Examiner Comments
2024B 16: 58% of candidates passed this question.
Good candidates structured their answers into formation, structure, and function of the platelets. The structure should be divided into the surface of the platelets, including the receptors and antigens, and contents of the platelets. The function should be divided into adhesion, activation, and aggregation in that order with an explanation of the triggers and events during these phases followed by a discussion of the interaction between platelets and the coagulation system in haemostasis.
17. Describe the physiological changes that occur in the pregnant person during parturition (labour). (Changes that occur within the foetus are not required).
CICMWrecks Answer
First Stage of Labour
Onset of Regular Uterine Contractions to Full Cervical Dilation (10 cm)
Endocrine and Uterine Changes
- ↑ Oxytocin (posterior pituitary):
- Stimulates uterine contractions via calcium-mediated pathways
- Enhanced by Ferguson reflex (positive feedback from cervical stretch)
- ↑ Prostaglandins (PGE2, PGF2α):
- Promote cervical ripening by enzymatic degradation of collagen and increased water content
- Enhance myometrial contractility
- ↑ Estrogen:Progesterone ratio:
- Increases oxytocin receptor expression in myometrium
- Reduces uterine quiescence
- Relaxin softens the cervix and pelvic connective tissues
Uterine and Cervical Physiology
- Myometrial contractions begin in the fundus and progress caudally
- Frequency: ~2–3 per 10 min early, increasing to 4–5 per 10 min by late first stage
- Duration increases from 30–60 to 60–90 seconds
- Cervical effacement and dilation proceed:
- Effacement: cervix thins from ~3 cm to a thin membrane
- Dilation: progresses from 0 to 10 cm
Cardiovascular Changes
- Cardiac output increases by ~30–50% above baseline
- Stroke volume ↑ due to enhanced venous return between contractions
- HR increases by 15–20 bpm
- Autotransfusion: ~300–500 mL of blood is displaced from uterus into systemic circulation during each contraction
- Systolic BP may rise transiently during contractions
Respiratory Changes
- Oxygen consumption increases by ~40–60%
- Minute ventilation increases by ~50%, driven primarily by increased tidal volume
- Resultant respiratory alkalosis: PaCO₂ decreases to 28–32 mmHg, with a slight ↑ in pH (up to 7.48)
Metabolic Changes
- ↑ Glucose demand for uterine muscle contractions
- Beginning of lactate accumulation due to intermittent anaerobic metabolism during strong contractions
- Mild ↑ in catecholamines and cortisol stimulate gluconeogenesis and energy mobilisation
Gastrointestinal and Neurological
- ↓ Gastric motility and LOS tone → increased risk of nausea/vomiting
- Pain mediated via visceral afferents (T10–L1) from uterine and cervical stretch
- ↑ Endorphins modulate pain and provide some stress-buffering effects
Second Stage of Labour
Full Cervical Dilation to Delivery of the Neonate
Endocrine and Uterine Muscular Changes
- Stronger, more coordinated fundal-dominant uterine contractions
- Frequency: ~5 contractions per 10 minutes
- Peak intrauterine pressure: 100–120 mmHg
- Maternal voluntary effort via abdominal muscle contraction and Valsalva manoeuvre aids fetal descent
Neurohormonal and Pain Modulation
- Somatic pain (S2–S4) due to distension of lower birth canal, perineum, and pelvic floor
- Further ↑ in β-endorphins from hypothalamus and pituitary provide endogenous analgesia
- Sympathetic activation → ↑ catecholamines (adrenaline, noradrenaline)
- Excessive stress can inhibit contractions and uteroplacental blood flow
Cardiovascular Changes
- Cardiac output peaks at 80–100% above pre-labour baseline
- Increased systemic blood pressure and heart rate during maternal pushing
- Risk of aortocaval compression in supine position → reduced venous return and hypotension; left lateral positioning recommended
Respiratory Changes
- ↑ Oxygen consumption approximates levels seen in moderate-to-heavy exercise
- Reaches 2.0–2.5 mL/kg/min
- Hyperventilation continues; persistent hypocapnia may lead to lightheadedness, tingling
Metabolic and GI Changes
- High lactate production in uterine muscle → risk of maternal acidosis in prolonged labour
- Glucose depletion in long second stages contributes to maternal fatigue
- Vomiting more likely due to raised intra-abdominal pressure and gastric stasis
Third Stage of Labour
Delivery of the Placenta
Endocrine and Uterine Haemostasis
- Oxytocin surges post-delivery:
- Promotes strong myometrial contraction
- Facilitates placental separation
- Prostaglandins also contribute to sustained myometrial tone
- Contraction of spiral arteries and “physiological ligature effect” from muscle fibres reduces haemorrhage
- Active management (e.g. oxytocin IM) used prophylactically to prevent postpartum haemorrhage
Cardiovascular Adjustments
- Blood loss ~300–500 mL in vaginal delivery
- May exceed 500 mL in postpartum haemorrhage
- Cardiovascular system compensates via vasoconstriction, ↑ HR, and continued autotransfusion from contracting uterus
Respiratory and Metabolic
- Respiratory demand begins to decline as physical effort ceases
- Continued elevation in metabolic rate for tissue repair and recovery
- Restoration of acid–base balance after lactate clearance
Neurological and GI
- Emotional relief and oxytocin release facilitate bonding and lactation initiation
- Endorphin levels remain elevated immediately postpartum
- Risk of vomiting persists due to residual gastric stasis and ongoing hormonal effects
Examiner Comments
2024B 17: 39% of candidates passed this question.
This question required consideration of the alterations of the uterus, and the hormonal changes during labour, as well as discussion of the effects of this on the other body systems. Cardiovascular and respiratory changes held most of the mark weighting however gastrointestinal, metabolic and neuronal changes were also required.
A good answer was structured using the 3 stages of labour to explain the hormonal changes that initiate and maintain labour (oxytocin and positive feedback the primary but not the only factor described), changes to the cervix, uterine muscle and active muscular effort, delivery of neonate and placenta and uterine mechanisms to limit blood loss. Using this structure a description of cardiovascular and respiratory changes should follow with some detail about pain/endorphins, abdominal pressure/LOS sphincter and metabolic changes (lactate/glucose utilisation) also expected. The question was specific to parturition. Physiological changes throughout pregnancy were not required.
18. Describe the anatomy relevant to the insertion of an intercostal catheter.
CICMWrecks Answer: Anatomy relevant to ICC Insertion
- Triangle of safety
- Lateral border of pectoralis major
- Anterior border of latissimus dorsi
- Superior to the 5th intercostal space – be aware the diaphragm (and liver/spleen) may be unexpectedly superior
- Layers (Superficial → Deep)
- Skin
- Subcutaneous connective tissue
- Fat
- Ribs and intercostal muscles
- Neurovascular bundle (intercostal artery, vein and nerves) lie in intercostal groove on infero-interior surface of the ribs
- External intercostal muscle
- Internal intercostal muscle
- Innermost intercostal muscle
- Parietal pleura
- Potential pleural space (pneumothorax)
- Visceral pleura
- Lung
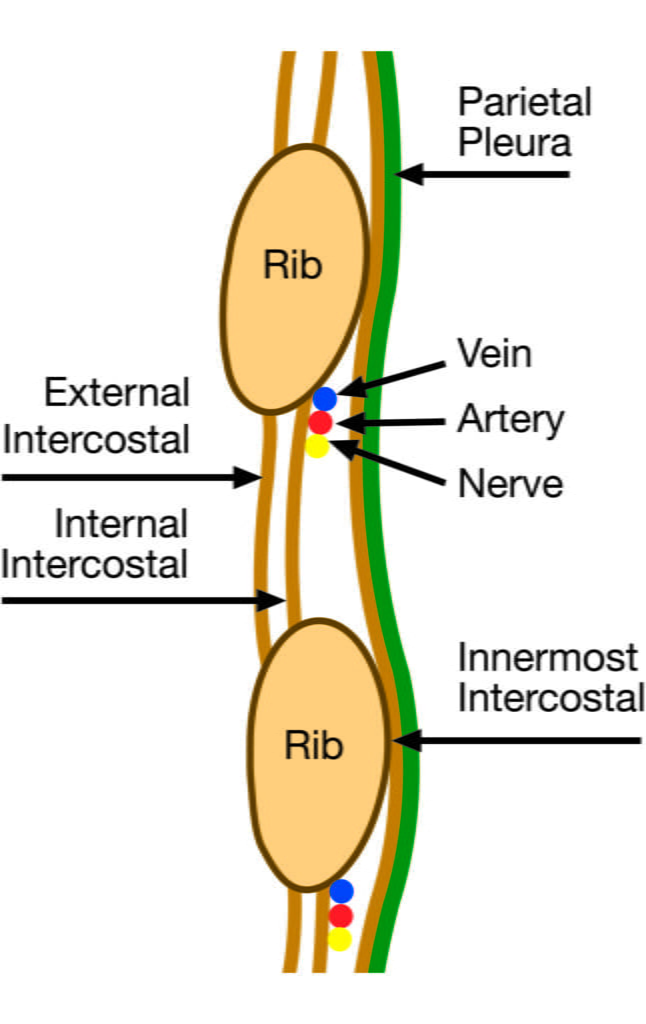
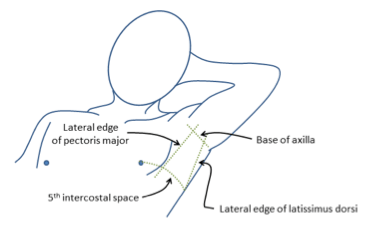
Sakurai / Mooney 2016
Examiner Comments
2024B 18: 36% of candidates passed this question.
This anatomy question reflects the knowledge base of a common ICU procedure. Good answers included insertion sites (lateral and anterior approaches) with the rationale and the relevant surface anatomy including the anatomical boundaries and neighbouring structures (pleural space, heart, liver and spleen) in relation to ICC insertion. A description of the layers of tissues traversed during ICC insertion including mention of the skin, subcutaneous tissues, superficial fascia, external – internal – innermost intercostal muscles, endothoracic fascia and parietal pleura was also required. Specific details describing the importance of the neurovascular bundle including its contents, the origin, trajectory and termination is important as this educates procedural considerations examined in other parts of the curriculum.
19. Describe the pharmacology of oxygen.
Examiner Comments
2024B 19: 33% of candidates passed this question.
Candidates were expected to describe the pharmacology of oxygen under the following headings: pharmaceutics/preparation, mechanism of action, indications/uses, administration methods, pharmacokinetics, pharmacodynamics and toxicity. Given the breadth of the question the detail of answers will be inherently limited by time however some specific details were important. Pharmaceutics included the properties of oxygen and its manufacturing and storage. Administration by inhalation may be via fixed and variable performance devices. Indications included causes of hypoxaemia but there are others such as de-nitrogenation of gas filled spaces, and various indications for hyperbaric O2 therapy. Mechanism of action involved mentioning oxidative phosphorylation occurring in the mitochondria where O2 functions as the final electron acceptor. Other mechanisms include biochemical pathways requiring O2 as a substrate, and generation of reactive oxygen species by neutrophils and macrophages for antimicrobial purposes. Pharmacokinetics required a description of diffusion across alveolar membrane, binding to Hb and the small amount in solution, metabolism in mitochondria and excretion subsequently of CO2 and H20. Answers were elevated by quantitation of O2 uptake, O2 carriage, mention of importance of pressure gradients (O2 cascade) and O2 stores. Pharmacodynamics required mention of systemic vasoconstriction, effects on the respiratory system such as de-nitrogenation of gas filled spaced, absorption atelectasis, inhibition of hypoxic pulmonary vasoconstriction and risk of hypercapnia in select patients with COPD. Side effects involve reactive oxygen species, and awareness of dose and time of exposure as relevant factors. There is a risk of delirium/seizures in hyperbaric oxygen, and longer-term risks of pulmonary toxicity and to the premature neonate of retrolental fibroplasia and retinopathy.
20. Explain the mechanism of action by which the following drugs exert their clinical and pharmacological effect when used to treat drug toxicity or overdose, including the time taken to exert this clinical effect:
(a) N-acetylcysteine (25% of marks).
(b) Digoxin FAb (25% of marks).
(c) Naloxone (25% of marks).
(d) Lipid emulsion (i.e. Intralipid) (25% of marks).
Examiner Comments
2024B 20: 65% of candidates passed this question.
This question was best answered by using each drug as the heading with their subsequent mechanism of action and time of onset of their clinical effect. Brief discussion of what drug toxicity or overdose these agents offset and how is implied in the mechanism of action and was included in our marking rubric.
VIVAs
A. Pharmaceutics | |
B. Pharmacokinetics | PK – single compartment model. Properties of drug. |
C. Pharmacodynamics | |
D. Variability in Drug Response | |
E. Cellular Physiology | |
F. Respiratory | West Zones. Pulmonary Blood flow distribution |
G. CVS | LV – PV loop, effect of drug X. |
H. Renal | |
I. Body Fluids and Electrolytes | Calcium |
J. Acid Base | Steward approach. ABG. |
K. Neuro | CSF flow from production to absorption |
L. Musculoskeletal | |
M. ANS | |
N. Liver | Liver lobule – diagram |
O. GIT | |
P. Nutrition and Metabolism | |
Q. Haematology | Factors which prevent blood clot propogation |
R. Thermoregulation | |
S. Immunology | |
T. Microbiology | |
U. Endocrine | |
V. Obstetrics | |
W. Measurement and Monitoring | |
X. Procedures |
Recent Comments